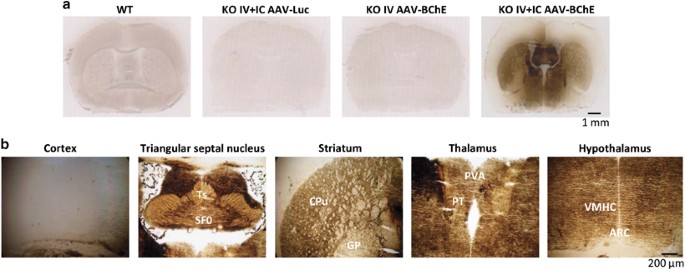
- Select a language for the TTS:
- UK English Female
- UK English Male
- US English Female
- US English Male
- Australian Female
- Australian Male
- Language selected: (auto detect) - EN
Play all audios:
ABSTRACT BACKGROUND: Ghrelin is the only orexigenic hormone known to stimulate food intake and promote obesity and insulin resistance. We recently showed that plasma ghrelin is controlled by
butyrylcholinesterase (BChE), which has a strong impact on feeding and weight gain. BChE knockout (KO) mice are prone to obesity on high-fat diet, but hepatic BChE gene transfer rescues
normal food intake and obesity resistance. However, these mice lack brain BChE and still develop hyperinsulinemia and insulin resistance, suggesting essential interactions between BChE and
ghrelin within the brain. METHODS: To test the hypothesis we used four experimental groups: (1) untreated wild-type mice, (2) BChE KO mice with LUC delivered by adeno-associated virus (AAV)
in combined intravenous (i.v.) and intracerebral (i.c.) injections, (3) KO mice given AAV for mouse BChE (i.v. only) and (4) KO mice given the same vector both i.v. and i.c. All mice ate a
45% calorie high-fat diet from the age of 1 month. Body weight, body composition, daily caloric intake and serum parameters were monitored throughout, and glucose tolerance and insulin
tolerance tests were performed at intervals. RESULTS: Circulating ghrelin levels dropped substantially in the KO mice after i.v. AAV–BChE delivery, which led to normal food intake and
healthy body weight. BChE KO mice that received AAV–BChE through i.v. and i.c. combined treatments not only resisted weight gain on high-fat diet but also retained normal glucose and insulin
tolerance. CONCLUSIONS: These data indicate a central role for BChE in regulating both insulin and glucose homeostasis. BChE gene transfer could be a useful therapy for complications linked
to diet-induced obesity and insulin resistance. SIMILAR CONTENT BEING VIEWED BY OTHERS GENETIC OR PHARMACOLOGICAL GHSR BLOCKADE HAS SEXUALLY DIMORPHIC EFFECTS IN RODENTS ON A HIGH-FAT DIET
Article Open access 25 May 2024 GIPR-AB/GLP-1 PEPTIDE–ANTIBODY CONJUGATE REQUIRES BRAIN GIPR AND GLP-1R FOR ADDITIVE WEIGHT LOSS IN OBESE MICE Article Open access 29 April 2025 BCAAS ACUTELY
DRIVE GLUCOSE DYSREGULATION AND INSULIN RESISTANCE: ROLE OF AGRP NEURONS Article Open access 06 June 2024 INTRODUCTION Butyrylcholinesterase (BChE, EC 3.1.1.8) is present in all mammals,
synthesized by the liver and secreted into the circulation. BChE is abundant in the bloodstream, but is also widespread elsewhere in the body, including the brain, skin, muscles, intestine,
lung, stomach, spleen, kidney, heart, thyroid and spinal cord.1 BChE previously attracted attention as a bioscavenger of drugs and organophosphate or carbamate insecticides.2 Recent findings
point toward a more specific physiological role in hydrolyzing the appetite-promoting hormone, 'ghrelin'.3, 4 Ghrelin is an acylated peptide released mostly by the stomach, but it
crosses the blood–brain barrier to communicate with central rewarding systems.5 BChE modulates ghrelin's biological functions by cleaving the peptide's _n_-octanoyl group, leaving
an inactive desacyl peptide.6, 7, 8 Thus, BChE orchestrates many physiological pathways involving ghrelin. Large clinical studies have reported that low serum BChE serves as a biochemical
marker in clinical conditions involving hepatocellular impairment from acute and chronic liver damage, cirrhosis and liver metastases.9 Low plasma BChE levels are also found in
protein–energy malnutrition and inflammatory states.10 On the contrary, plasma BChE activity is elevated in obese subjects and patients with type 1 or type 2 diabetes.10, 11 High plasma BChE
activity is associated with aberrant lipid profiles, insulin resistance and hypertension.11, 12 Together, these findings suggest a role for BChE in many metabolic functions and, possibly,
in the insulin resistance syndrome. However, it is not known whether high BChE levels are a cause of such metabolic lesions, or whether they are a response to them. In light of BChE's
impact on ghrelin regulation and its altered expression in obese and diabetic patients, we hypothesize that it serves to adjust energy balance, especially on rich diets. To investigate
physiological roles of BChE, 10 years ago, Lockridge and co-workers13 generated the first BChE knockout (KO) mice. Their only observed phenotype was readily growing obese on high-fat
diets.14 We recently confirmed that result while also revealing that their plasma ghrelin was 50% higher.15 However, this effect was completely suppressed by one systemic injection of
adeno-associated viral vector coding for BChE. Surprisingly, however, although treated KO mice retained healthy weight and normal calorie intake, they still developed hyperinsulinemia.15 A
plausible explanation is that systemic BChE vector injection elevates enzyme only in the periphery, leaving nonexistent BChE in the brain. Here, we used bilateral vector brain injections to
dissect the functional roles of peripheral and central BChE in regulating body fat as well as insulin secretion. MATERIALS AND METHODS ANIMAL SUBJECTS AND ETHICS Adult male wild-type C57BL/6
and BChE KO mice of the same strain were obtained from Jackson Labs (Bar Harbor, ME, USA) under protocol A53015 approved by Mayo Clinic's Institutional Animal Care and Use Committee.
Experiments were conducted in accord with the Guide for Care and Use of Laboratory Animals16 in a facility approved by the American Association for the Accreditation of Laboratory Animal
Care. Under our protocol, 1-month-old mice with initially similar body weight were fed for 6 months on a diet containing 45% calories from fat, 20% from protein and 35% from carbohydrate
(Research Diet). INTRAVENOUS INJECTION Complementary DNA encoding luciferase (Luc) or mouse BChE was subcloned into an adeno-associated virus (AAV) backbone. The resulting transfer vectors
were co-transfected into HEK293T cells with helper vectors pHELP (Applied Viromics, Fremont, CA, USA) and pAAV 2/8 (University of Pennsylvania) as described.17 Virus in cell lysates was
isolated by ultracentrifugation, and viral particles were quantitated by real-time PCR. Vector (200 μl, 1 × 1012 viral particles) was given to 10-week-old mice via tail-vein, followed by 200
μl of 0.9% sterile NaCl. INTRACEREBRAL INJECTION BChE KO mice (10-week-old) were anesthetized with 50 mg kg−1 pentobarbital, and 10 μl of AAV–BChE containing 1.5 × 1012 viral particles was
delivered (1 μl min−1) in two injections, bilaterally targeting the hypothalamic arcuate nucleus. Intracerebral injection was performed on a Kopf stereotaxic frame with mouse adapters at
coordinates: _A_=2.1 mm, _L_=0.5 mm, _V_=5.0 MM. Cannulae were left in place for 3 min and then withdrawn. BODY COMPOSITION AND FOOD INTAKE Fat mass and lean mass were assessed in an
EchoMRI-100 body composition analyzer (EchoMRI LLC, Houston, TX, USA). Food intake was determined daily for 2 weeks in singly housed mice with cage bedding replaced by 'Iso-pads'
in order to account for every grain of residual food. PLASMA ANALYSIS Random-fed samplings were made between 0900 and 1000 hours, and fasting samples were obtained at 1400 hours after 6 h of
food deprivation. Blood glucose concentrations were measured by blood glucometers from mouse tail tips (Bayer HealthCare, Mishawaka, IN, USA). Other parameters were measured from plasma.
Blood (~0.2 ml) was taken by cheek puncture with a mouse-lancet in ice-cooled EDTA tubes and centrifuged for 10 min at 8000 _g_. Insulin concentrations were measured by an ELISA kit (Alpco,
Salem, NH, USA). BChE activity was determined by Ellman assay as described previously.18 Ghrelin samples were taken after 16 h fasting and collected in cooled EDTA-treated tubes with 0.1 vol
of 1N HCl, protected by proteinase inhibitors (1 mM p-hydroxymercuribenzoic acid; Sigma-Aldrich, St Louis, MO, USA and 1.5 μM aprotinin; Roche). Samples were centrifuged 10 min at 8000 _g_
and stored at −80 °C. ELISA kits were used to measure ghrelin and desacyl-ghrelin levels (Cayman Chemical, Ann Arbor, MI, USA). GLUCOSE TOLERANCE TEST AND INSULIN TOLERANCE TEST
Six-hour-fasted mice were given 1.0 g kg−1 of glucose (for glucose tolerance test) or 0.75 U kg−1 of Eli Lilly human insulin (for insulin tolerance test) by intraperitoneal injection. Blood
glucose concentration was monitored before and 15, 30, 60, 90 and 120 min after injection. Mice were single-caged in random order. HISTOCHEMICAL BCHE DETECTION Cryostat brain sections (14
μm) were fixed in 4% paraformaldehyde (pH 7.4) for 30 min. BChE activity was examined by Koelle–Friedenwald histochemistry with minor modification.19 Brain sections were incubated overnight
in a copper glycinate medium (pH 5.0) containing 3 mM copper sulfate, 10 mM glycine, 35 mM sodium acetate, 3 mM butyrylthiocholine and 200 μM 1,5-bis(4-allyldimethylammoniumphenyl)
pentan-3-one dibromide (BW284C51; Sigma-Aldrich). After four cycles of rinsing with distilled water, slices were incubated in 160 mM sodium sulfide buffer (pH 7.5) for 30 min, followed by
another four water rinses. The reaction was finished up in 1% silver nitrate for 30 min. Rinsed sections were air-dried and mounted in EZ-Mount mounting medium (Thermo Scientific, Waltham,
MA, USA). Images were captured on a light microscopic (Carl Zeiss Microscopy GmbH, Jena, Germany). DATA ANALYSIS Two-group comparisons were conducted with a two-tailed _t-_test, and
_P_<0.05 was considered significant. Daily food intake data were analyzed by two-factor mixed analysis of variance followed by Holm–Sidak multiple comparison tests.20 Sample sizes (10
mice per group) were chosen in light of our previous experiments of similar design and supported by previous power analysis. The variation of measured responses was similar within treatment
groups. RESULTS BCHE EXPRESSION IN VECTOR-TREATED KO MICE As we and others have consistently observed excess body weight in BChE KO male mice on high-fat diets, we hypothesized that both
central and peripheral BChE must have an impact on fat metabolism. To test this idea, we treated BChE KO mice with AAV vector for mouse BChE in two ways: (1) via tail-vein (intravenous,
i.v.), which led to high levels of circulating BChE and (2) double injections (i.v. and intracerebral (i.c.)), which generated abundant BChE in both peripheral and central tissues (Table 1
and Figure 1). A rich diet (45% calorie fat) was provided to age-matched wild-type and BChE KO mice from 4 weeks of age. Vectors were injected when the mice reached 10 weeks. Vector carrying
the Luc gene served as another treatment control. Sham injection with i.c. saline was carried out in the single i.v. injection group. Giving BChE vector to KO mice led to stably high enzyme
expression in plasma (>50 U ml−1), well above natural levels in wild-type controls (~1.6 U ml−1, Table 1). Accompanying the high BChE expression was a large drop in plasma acyl ghrelin,
and a comparable rise in desacyl-ghrelin, altering the ratio between the two peptides (Table 1). Activity staining of coronal brain sections revealed widespread BChE expression in most brain
areas, with highest levels in the triangular septal nucleus, striatum, thalamus and hypothalamus, but much lower levels in the cortex (Figure 1). BODY COMPOSITION AND FOOD INTAKE We
assessed the effect of vector treatments on body mass and composition over time. In the first week after vector injections, the mice in all groups showed similar and minor weight losses.
This effect, likely owing to surgical stress, dissipated within 6 days (Figure 2a). After 18 weeks, however, the KOs given no BChE vector were 45% heavier than those at the outset. In
contrast, over the same period, weight gain was only 20% in the mice given BChE vector, i.v., or i.v.+i.c., (Figure 2b). The excess weight gain in mice with no BChE was almost exclusively
due to an abnormal increase in fat mass, which was 60% greater than in wild-type controls and BChE vector-treated KO mice (Figure 2c). Terminal tissue harvests revealed that inguinal but not
epididymal fat pads were substantially heavier in untreated KO mice than in wild type, but they were equivalent to the normal controls in the vector-treated KO mice (Figure 2d). Careful
assessment of daily food intake throughout the 2-week study confirmed that untreated KO mice ate more food than wild-type controls and vector-treated KO mice (Figure 3a). Average weekly
caloric intake in the KO group was ~10% more than the other three groups (Figure 3b). Double vector injections (i.c.+i.v.) had no added effect on energy consumption compared with single i.v.
injections. GLUCOSE HOMEOSTASIS Human obesity is strongly associated with type 2 diabetes, but despite the aberrant body weights in our fat mice, their fasting blood glucose remained
closely similar to those in wild-type and vector-treated KO mice (Figure 4a). However, when we challenged fasted animals with intraperitoneal glucose (1.0 g kg−1), the KO mice given double
BChE vector treatments (i.v. and i.c.) cleared injected sugar faster than singly treated cohorts (i.v. only, Figure 4b). This effect was reflected in an ~20% reduction in 'area under
the curve' relative to wild-type control and untreated KO (Figure 4c). In contrast, KO mice singly treated with i.v. AAV–BChE vector exhibited no differences in glucose tolerance as
compared to the KO and wild-type mice that received no vector (Figures 4b and c). Because insulin strongly affects blood glucose, we followed its levels during the glucose tolerance test.
After glucose administration, insulin rose noticeably in the untreated and i.v vector-treated KO mice after glucose administration but not in the double i.v. and i.c. treated KO (Figure 4d).
Further study showed that BChE KO mice developed a hyperinsulinemia that persisted in both fed and fasting status (Figure 5a). Likewise, KO mice given only i.v. AAV–BChE treatment also
showed elevated insulin levels (Figure 5a) and were less sensitive than wild-type controls in the insulin tolerance test (Figures 5b and c). This outcome implies that, despite healthy body
weight and normal food intake, our singly treated mice still became diabetic. In contrast, doubly treated mice (i.v.+i.c.) retained fed and fasting insulin levels comparable to wild-type
cohorts, and were also similar in their response to insulin (Figures 5b and c). DISCUSSION Our study showed that diet-induced metabolic lesions in BChE KO mice were fully rescued by
simultaneous enzyme overexpression in the brain as well as in the bloodstream. The outcomes of systemic vector alone contrasted strikingly with dual treatment with systemic and cerebral
vector. We think this result points to an unexpected role for brain BChE. Thus, in systemically treated KO mice, high circulating BChE is able to bring down plasma ghrelin levels and
suppress overweight and overeating. Nevertheless, these healthy lean KO mice that lacked brain BChE still developed diabetic symptoms of hyperinsulinemia and impaired insulin sensitivity. In
contrast, coupling systemic with i.c. injection provided widespread BChE expression in the brain and erased all obesity-induced perturbations in glucose and insulin tolerance. Body mass
differences in this study were almost exclusively due to altered fat mass. This outcome aligns well with our previous report that BChE has a sparing effect on lean mass.15 That effect likely
reflected altered ghrelin signaling in peripheral and central systems. Ghrelin originates largely from the stomach, but it signals to the brain through multiple pathways.21 Ghrelin is also
produced in certain brain regions, including the hypothalamus, and it activates NPY/AgRP neurons that stimulate feeding and decrease energy expenditure.22, 23 The hypothalamus is critical in
relaying afferent signals from the gut and brainstem and in processing efferent signals that modulate food intake and energy expenditure.24, 25 There are three possible pathways for BChE to
influence ghrelin's appetite-inducing effect. First, circulating BChE can hydrolyze ghrelin in the bloodstream before it crosses the blood–brain barrier. Second, central BChE can
regulate ghrelin produced locally in the hypothalamus where it may directly affect various hypothalamic nuclei. Third, BChE in the stomach may reduce the ghrelin signaling that reaches the
brain via the afferent vagal nerve to the central motor vagus nuclei. High-fat feeding leads to weight gain and obesity26 and is also associated with insulin resistance.27 Although our
glucose tolerance and insulin tolerance tests showed impaired glucose clearance and reduced insulin sensitivity in BChE KO mice, blood glucose levels were essentially normal. As in many
other diabetic models,28 impaired insulin sensitivity in the BChE KO mice was associated with increased insulin levels and secretion in response to a glucose challenge. We saw much better
glucose homeostasis in the bilaterally treated KO mice, evidenced by increased glucose disposal, decreased insulin secretion and increased sensitivity to insulin injection. The improved
insulin responsiveness in vector-treated BChE KO mice may be due to the altered central ghrelin levels and the sensitivity of its receptor. Ghrelin inhibits insulin release in mice, rat and
humans.29 Ablation of ghrelin or its receptor enhances insulin release and prevents impaired glucose tolerance in high-fat diet-induced obese mice.30, 31 Ghrelin removal also improves
diabetic symptoms in genetically leptin-deficient mice.32, 33 Overexpressing BChE in the brain, especially in the hypothalamus, may re-sensitize ghrelin's receptor and thereby maximize
glucose-induced insulin release, enabling insulin secretion to meet the high demand associated with diet-induced obesity. Neuronal pathways link the central nervous system with the autonomic
innervation of the endocrine pancreas, which controls glucose homeostasis.34 Cholinergic pathways have an important role in maintaining energy and glucose homeostasis.35, 36 The cholinergic
vagus nerve has been shown to stimulate insulin secretion and pancreatic cell proliferation.37, 38 It is plausible that, under a long-term high-fat diet, BChE, despite low efficiency in
hydrolyzing acetylcholine, may modulate the cholinergic pathway for insulin secretion and consequently aid glucose homeostasis. Our studies suggest that elevating BChE expression with AAV
vector will have beneficial effects in controlling food intake and obesity-related disorders, and may be of future clinical value. A large excess of BChE in the blood and brain caused no
obvious deficiency of cognition or motor function in our mice. In fact, for other reasons we have conducted extensive toxicology studies on the same viral vector without finding any
physiologic effect from BChE enzyme or its viral host in healthy mice.39 For that reason, a role for BChE in regulating insulin sensitivity was unexpected and has not yet been rigorously
defined. However, this role may well be to serve in modulating ghrelin levels and ghrelin receptor sensitivity to affect lipid metabolism. If so, BChE expression vectors may prove effective
as therapeutics for obesity, type 2 diabetes and related conditions. The present study has provided unexpected evidence that BChE in the central brain may have important roles in clearing
blood glucose and modulating insulin sensitivity. We recognize a few limitations of our work. One is that different viral vector doses were used for the i.v. and i.c. treatments that led to
altered plasma levels of BChE and ghrelin. Second, the specific areas of the brain crucial to BChE’s central role remain to be pinpointed, although the hypothalamus and dorsal motor nuclei
of the vagus nerve are likely involved. Third, the role of ghrelin and its receptor sensitivity in the BChE KO mice model is worth pursuing with genetic approaches. Fourth, new viral vectors
with improved CNS access need study to obviate the invasiveness of intracranial vector delivery. Finally, it should be of great interest to pinpoint other pathways by which BChE may have an
impact on diet-induced obesity. REFERENCES * Lockridge O . Review of human butyrylcholinesterase structure, function, genetic variants, history of use in the clinic, and potential
therapeutic uses. _Pharmacol Ther_ 2015; 148: 34–46. Article CAS Google Scholar * Marsillach J, Costa LG, Furlong CE . Protein adducts as biomarkers of exposure to organophosphorus
compounds. _Toxicology_ 2013; 307: 46–54. Article CAS Google Scholar * De Vriese C, Gregoire F, Lema-Kisoka R, Waelbroeck M, Robberecht P, Delporte C . Ghrelin degradation by serum and
tissue homogenates: identification of the cleavage sites. _Endocrinology_ 2004; 145: 4997–5005. Article CAS Google Scholar * Chen VP, Gao Y, Geng L, Parks RJ, Pang YP, Brimijoin S .
Plasma butyrylcholinesterase regulates ghrelin to control aggression. _Proc Natl Acad Sci USA_ 2015; 112: 2251–2256. Article CAS Google Scholar * Kojima M, Kangawa K . Ghrelin: structure
and function. _Physiol Rev_ 2005; 85: 495–522. Article CAS Google Scholar * Schopfer LM, Lockridge O, Brimijoin S . Pure human butyrylcholinesterase hydrolyzes octanoyl ghrelin to desacyl
ghrelin. _Gen Comp Endocrinol_ 2015; 224: 61–68. Article CAS Google Scholar * Brimijoin S, Chen VP, Pang Y-P, Geng L, Gao Y . Physiological roles for butyrylcholinesterase: a
BChE-ghrelin axis. _Chem-Biol Interact_ 2016; 259: 271–275. Article CAS Google Scholar * Chen VP, Gao Y, Geng L, Brimijoin S . Radiometric assay of ghrelin hydrolase activity and
3H-ghrelin distribution into mouse tissues. _Biochem Pharmacol_ 2015; 98: 732–739. Article Google Scholar * Santarpia L, Grandone I, Contaldo F, Pasanisi F . Butyrylcholinesterase as a
prognostic marker: a review of the literature. _J Cachexia Sarcopenia Muscle_ 2013; 4: 31–39. Article Google Scholar * Lampon N, Hermida-Cadahia EF, Riveiro A, Tutor JC . Association
between butyrylcholinesterase activity and low-grade systemic inflammation. _Ann Hepatol_ 2012; 11: 356–363. CAS PubMed Google Scholar * Randell E, Mathews M, Zhang H, Seraj J, Sun G .
Relationship between serum butyrylcholinesterase and the metabolic syndrome. _Clin Biochem_ 2005; 38: 799–805. Article CAS Google Scholar * Sridhar GR, Rao AA, Srinivas K, Nirmala G,
Lakshmi G, Suryanarayna D _et al_. Butyrylcholinesterase in metabolic syndrome. _Med Hypotheses_ 2010; 75: 648–651. Article CAS Google Scholar * Li B, Duysen EG, Saunders TL, Lockridge O
. Production of the butyrylcholinesterase knockout mouse. _J Mol Neurosci_ 2006; 30: 193–195. Article CAS Google Scholar * Li B, Duysen EG, Lockridge O . The butyrylcholinesterase
knockout mouse is obese on a high-fat diet. _Chem Biol Interact_ 2008; 175: 88–91. Article CAS Google Scholar * Chen VP, Gao Y, Geng L, Stout MB, Jensen MD, Brimijoin S .
Butyrylcholinesterase deficiency promotes adipose tissue growth and hepatic lipid accumulation in male mice on high-fat diet. _Endocrinology_ 2016; 157: 3086–3095. Article Google Scholar *
National Research Council. _Guide for the Care and Use of Laboratory Animals_, 8th edn. National Academies Press: Washington, DC, USA, 2011.. * Geng L, Gao Y, Chen X, Hou S, Zhan C-G, Radic
Z _et al_. Gene transfer of mutant mouse cholinesterase provides high lifetime expression and reduced cocaine responses with no evident toxicity. _PLoS One_ 2013; 8: e67446. Article CAS
Google Scholar * Chen VP, Xie HQ, Chan WK, Leung KW, Chan GK, Choi RC _et al_. The PRiMA-linked cholinesterase tetramers are assembled from homodimers: hybrid molecules composed of
acetylcholinesterase and butyrylcholinesterase dimers are up-regulated during development of chicken brain. _J Biol Chem_ 2010; 285: 27265–27278. Article CAS Google Scholar * Hammond PI,
Jelacic T, Padilla S, Brimijoin S . Quantitative, video-based histochemistry to measure regional effects of anticholinesterase pesticides in rat brain. _Anal Biochem_ 1996; 241: 82–92.
Article CAS Google Scholar * Abdi H. The Bonferonni and Šidák corrections for multiple comparisons. In: Salkind NJ (ed). _Encyclopedia of Measurement and Statistics_, Sage: Thousand Oaks,
CA, USA, 2007, pp 103–107.. * Banks WA, Tschop M, Robinson SM, Heiman ML . Extent and direction of ghrelin transport across the blood-brain barrier is determined by its unique primary
structure. _J Pharmacol Exp Ther_ 2002; 302: 822–827. Article CAS Google Scholar * Cowley MA, Smith RG, Diano S, Tschop M, Pronchuk N, Grove KL _et al_. The distribution and mechanism of
action of ghrelin in the CNS demonstrates a novel hypothalamic circuit regulating energy homeostasis. _Neuron_ 2003; 37: 649–661. Article CAS Google Scholar * Korbonits M, Goldstone AP,
Gueorguiev M, Grossman AB . Ghrelin–a hormone with multiple functions. _Front Neuroendocrinol_ 2004; 25: 27–68. Article CAS Google Scholar * Simpson KA, Martin NM, Bloom SR . Hypothalamic
regulation of food intake and clinical therapeutic applications. _Arq Bras Endocrinol Metabol_ 2009; 53: 120–128. Article Google Scholar * Palkovits M . Hypothalamic regulation of food
intake. _Ideggyogy Sz_ 2003; 56: 288–302. PubMed Google Scholar * Jen KL . Effects of diet composition on food intake and carcass composition in rats. _Physiol Behav_ 1988; 42: 551–556.
Article CAS Google Scholar * Storlien LH, James DE, Burleigh KM, Chisholm DJ, Kraegen EW . Fat feeding causes widespread _in vivo_ insulin resistance, decreased energy expenditure, and
obesity in rats. _Am J Physiol_ 1986; 251 (5 Pt 1): E576–E583. CAS PubMed Google Scholar * Shanik MH, Xu Y, Skrha J, Dankner R, Zick Y, Roth J . Insulin resistance and hyperinsulinemia:
is hyperinsulinemia the cart or the horse? _Diabetes Care_ 2008; 31 (Suppl 2): S262–S268. Article CAS Google Scholar * Dezaki K . Ghrelin function in insulin release and glucose
metabolism. _Endocr Dev_ 2013; 25: 135–143. Article CAS Google Scholar * Dezaki K, Sone H, Koizumi M, Nakata M, Kakei M, Nagai H _et al_. Blockade of pancreatic islet-derived ghrelin
enhances insulin secretion to prevent high-fat diet-induced glucose intolerance. _Diabetes_ 2006; 55: 3486–3493. Article CAS Google Scholar * Lin L, Saha PK, Ma X, Henshaw IO, Shao L,
Chang BH _et al_. Ablation of ghrelin receptor reduces adiposity and improves insulin sensitivity during aging by regulating fat metabolism in white and brown adipose tissues. _Aging Cell_
2011; 10: 996–1010. Article CAS Google Scholar * Sun Y, Asnicar M, Saha PK, Chan L, Smith RG . Ablation of ghrelin improves the diabetic but not obese phenotype of ob/ob mice. _Cell
Metabol_ 2006; 3: 379–386. Article CAS Google Scholar * Yada T, Dezaki K, Sone H, Koizumi M, Damdindorj B, Nakata M _et al_. Ghrelin regulates insulin release and glycemia: physiological
role and therapeutic potential. _Curr Diabetes Rev_ 2008; 4: 18–23. Article CAS Google Scholar * Thorens B . Central control of glucose homeostasis: the brain–endocrine pancreas axis.
_Diabetes Metab_ 2010; 36 (Suppl 3): S45–S49. Article CAS Google Scholar * Berthoud HR . The vagus nerve, food intake and obesity. _Regul Peptides_ 2008; 149: 15–25. Article CAS Google
Scholar * Dockray GJ . The versatility of the vagus. _Physiol Behav_ 2009; 97: 531–536. Article CAS Google Scholar * Kiba T . Relationships between the autonomic nervous system and the
pancreas including regulation of regeneration and apoptosis: recent developments. _Pancreas_ 2004; 29: e51–e58. Article Google Scholar * de Azua IR, Gautam D, Guettier J-M, Wess J . Novel
insights into the function of β-cell M(3) muscarinic acetylcholine receptors: therapeutic implications. _Trends Endocrinol Metab_ 2011; 22: 74–80. Article Google Scholar * Murthy V, Gao Y,
Geng L, LeBrasseur NK, White TA, Parks RJ _et al_. Physiologic and metabolic safety of butyrylcholinesterase gene therapy in mice. _Vaccine_ 2014; 32: 4155–4162. Article CAS Google
Scholar Download references ACKNOWLEDGEMENTS This research was funded by grant MNP13.05 from the Minnesota Partnership for Biotechnology and Medical Genomics, by the Mayo Foundation for
Medical Research and by the National Institute on Drug Abuse (Grant DP1 DA31340-01 and DA42492). AUTHOR INFORMATION AUTHORS AND AFFILIATIONS * Department of Molecular Pharmacology and
Experimental Therapeutics, Mayo Clinic, Rochester, 55905, MN, USA V P Chen, Y Gao, L Geng & S Brimijoin Authors * V P Chen View author publications You can also search for this author
inPubMed Google Scholar * Y Gao View author publications You can also search for this author inPubMed Google Scholar * L Geng View author publications You can also search for this author
inPubMed Google Scholar * S Brimijoin View author publications You can also search for this author inPubMed Google Scholar CORRESPONDING AUTHORS Correspondence to V P Chen or S Brimijoin.
ETHICS DECLARATIONS COMPETING INTERESTS The authors declare no conflicts of interest. RIGHTS AND PERMISSIONS This work is licensed under a Creative Commons
Attribution-NonCommercial-ShareAlike 4.0 International License. The images or other third party material in this article are included in the article’s Creative Commons license, unless
indicated otherwise in the credit line; if the material is not included under the Creative Commons license, users will need to obtain permission from the license holder to reproduce the
material. To view a copy of this license, visit http://creativecommons.org/licenses/by-nc-sa/4.0/ Reprints and permissions ABOUT THIS ARTICLE CITE THIS ARTICLE Chen, V., Gao, Y., Geng, L.
_et al._ Butyrylcholinesterase regulates central ghrelin signaling and has an impact on food intake and glucose homeostasis. _Int J Obes_ 41, 1413–1419 (2017).
https://doi.org/10.1038/ijo.2017.123 Download citation * Received: 16 March 2017 * Revised: 21 April 2017 * Accepted: 03 May 2017 * Published: 22 May 2017 * Issue Date: September 2017 * DOI:
https://doi.org/10.1038/ijo.2017.123 SHARE THIS ARTICLE Anyone you share the following link with will be able to read this content: Get shareable link Sorry, a shareable link is not
currently available for this article. Copy to clipboard Provided by the Springer Nature SharedIt content-sharing initiative