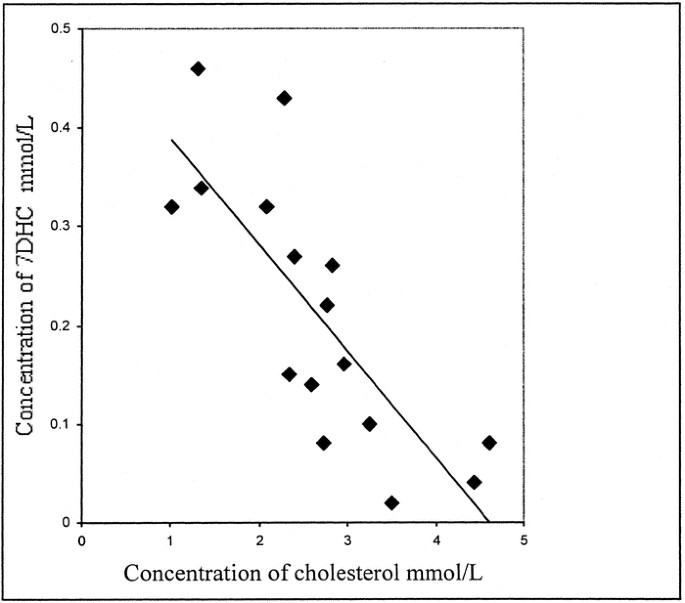
- Select a language for the TTS:
- UK English Female
- UK English Male
- US English Female
- US English Male
- Australian Female
- Australian Male
- Language selected: (auto detect) - EN
Play all audios:
ABSTRACT Smith-Lemli-Opitz syndrome is a condition of impaired cholesterol synthesis that is caused by mutations in _DHCR7_ encoding 7-dehydrocholesterol-Δ7 reductase. Birth defects and
mental retardation are characteristic. Deficient plasma and tissue cholesterol and excess cholesterol precursors 7 and 8 dehydrocholesterol (7DHC and 8DHC) contribute to the pathogenesis.
Cholesterol is transported to tissues _via_ lipoproteins. We measured the effect of dietary cholesterol (egg yolk) on plasma lipoproteins to evaluate this potential treatment. We used the
enzymatic method to measure total sterols in lipoproteins (_n_ = 12) and plasma (_n_ = 16). In addition, we analyzed individual plasma sterols by a gas chromatographic method. Samples were
evaluated after 3 wk of a cholesterol-free diet and after 6–19 mo of dietary cholesterol. We also analyzed the distribution of sterols in lipoproteins and the apolipoprotein E genotype.
Dietary cholesterol significantly increased the total sterols in plasma (2.22 ± 0.13 to 3.10 ± 0.22; mean ± SEM; _p_ < 0.002), in LDL (0.98 ± 0.13 to 1.52 ± 0.17 mM), and in HDL (0.72 ±
0.04 to 0.92 ± 0.07). Plasma cholesterol increased (1.78 ± 0.16 to 2.67 ± 0.25 mM; _p_ < 0.007) and plasma 7DHC decreased in 10 children, but the mean decrease was not significant. The
distribution of individual sterols in each lipoprotein fraction was similar to the distribution in plasma. The baseline cholesterol and the response to dietary cholesterol was the same in
children with 3/3 and 3/4 apolipoprotein E genotypes. Dietary cholesterol increased total sterols in plasma, LDL, and HDL in children with Smith-Lemli-Opitz syndrome. These favorable
increases in the lipoproteins are potentially therapeutic for this condition. SIMILAR CONTENT BEING VIEWED BY OTHERS EFFECTS OF PHYTOSTEROL SUPPLEMENTATION ON LIPOPROTEIN SUBFRACTIONS AND
LDL PARTICLE QUALITY Article Open access 15 May 2024 METABOLISM OF TRIGLYCERIDE-RICH LIPOPROTEINS IN HEALTH AND DYSLIPIDAEMIA Article 22 March 2022 METABOLOMIC PROFILING OF A CHOLESTEROL
LOWERING PLANT-BASED DIET FROM TWO RANDOMIZED CONTROLLED FEEDING TRIALS Article 22 April 2025 MAIN The Smith-Lemli-Opitz syndrome (SLOS) is an autosomal recessive condition with multiple
malformations and mental retardation (OMIM #270400). SLOS is caused by mutations in the gene encoding the final enzyme in the cholesterol biosynthetic pathway 7-dehydrocholesterol
Δ7-reductase (DHCR7; E.C 1.3.1.21). Although the syndrome was initially described in 1964 (1), it was not until 30 y later that deficiency of a cholesterol biosynthetic enzyme was identified
as the cause of the syndrome (2–4). The identification and cloning of the gene defective in SLOS, _DHCR7_, were reported in 1998 (5–7). Currently, >80 different mutations in _DHCR7_ have
been found to be associated with SLOS (8,9). SLOS is characterized clinically by microcephaly, cleft palate, dysmorphic facies, limb abnormalities (especially syndactyly of toes 2 and 3),
genital anomalies, endocrine malfunction, cataracts, malformation in the heart and kidney, mental retardation, feeding difficulties, and growth retardation (10). Clinical features are
variable in severity, presumably reflecting the specific causative mutations and the extent of the decrease in enzyme activity. Some mildly affected patients exhibit only a few of the
described features. The biochemical consequences of the enzyme deficiency include abnormally low or low-normal cholesterol concentrations along with accumulation of the precursor that is the
substrate of DHCR7, 7-dehydrocholesterol (7DHC), and its derivative 8-dehydrocholesterol (8DHC). Treatment is sought to correct the biochemical imbalance and improve physical and
psychomotor development. Several studies have been conducted to evaluate the effects of supplemental and dietary cholesterol as a potential treatment for this condition (11–19). These
studies evaluated the effect of supplemental and/or dietary cholesterol on plasma sterol (cholesterol, 7DHC, 8DHC) concentrations. In the current study, in addition to measuring plasma
sterols, we analyzed lipoproteins (LDL, HDL, VLDL, and triglyceride) to test the hypothesis that dietary cholesterol will increase total sterols in LDL. A second objective was to determine
the distribution of cholesterol, 7DHC, and 8DHC in lipoproteins because this has not been reported previously. We measured lipoprotein sterols at baseline (after 3 wk of an essentially
cholesterol-free diet) and after 6–19 mo of dietary cholesterol primarily from egg yolk. A third objective was to determine whether apolipoprotein E (apoE) genotype affects the response to
dietary cholesterol. METHODS SUBJECTS AND STUDY DESIGN. Sixteen children with SLOS were enrolled; two of the children were siblings. We have previously reported data on plasma sterol levels,
sterol synthesis, urinary mevalonate, and DNA mutations in as many as 14 of these children (19–22), but we have not previously reported lipoprotein data. All study children had clinical
features of SLOS and elevated 7DHC and 8DHC plasma concentrations diagnostic of SLOS. The children (Table 1) were admitted to the Oregon Health & Science University General Clinical
Research Center for 1-wk periods. Parents were instructed to feed their child an essentially cholesterol-free diet (baseline diet) at home for 3–4 wk before the first admission to establish
baseline conditions. The cholesterol content of these diets ranged from 0.4 to 3.3 mg cholesterol · kg−1 · d−1; one child was breast fed and consumed 14 mg cholesterol · kg−1 · d−1 contained
in breast milk. The baseline diet period was repeated when the initial study was unsuccessful as a result of illness, nonadherence to diet, difficulties with sample collection, or other
reason. During these admissions, the baseline diet was continued under metabolic ward conditions. On discharge, children were prescribed dietary cholesterol as egg yolk and/or butter fat. In
a few cases, crystalline cholesterol in oil or emulsion was prescribed. For all other admissions, the children continued to receive cholesterol. Children returned every 3–12 mo for
monitoring. Blood samples were evaluated during the last week of the baseline diet and after 6–19 mo of dietary cholesterol. Details of the diets are described in previous reports (18,19).
In summary, children aged 0–6 mo were fed one half egg yolk/d; children aged 6–12 mo were fed 1 egg yolk/d; and children aged 12 mo or older were fed 1.5 to 2 egg yolks/d. On the basis of an
estimate of 210 mg of cholesterol per egg yolk, the children received ∼18–60 mg · kg−1 · d−1 of cholesterol. Eggs were hardboiled, and the yolks removed and mixed with formula, breast milk,
or solid food, according to the child's regular diet. At times, egg yolk was cooked in formula or milk. Egg yolks were also provided as powdered egg yolk (subjects 5 and 9) or as whole
eggs (in subject 8 for 46% of the duration of the dietary cholesterol period). Powdered egg yolk was stored at 4°C to prevent oxidation of its cholesterol. Six children received butter fat,
heavy cream, crystalline cholesterol in soybean oil (16), or crystalline cholesterol in an aqueous suspension (23) instead of egg yolk during some or all of the supplemental cholesterol
period. Table 2 describes the source and duration of the supplemental cholesterol (other than egg yolk) for these children. Feeding difficulties are common in SLOS, and seven of the 16
children in our study required tube feeding. The same dietary protocols were used for children who were tube fed or orally fed. These studies were approved by the Oregon Health & Science
University (Portland, OR) Institutional Review Board; the parents gave informed consent for the participation of their child. LIPOPROTEIN AND PLASMA STEROL ANALYSIS. Total sterols in each
lipoprotein fraction and in plasma as well as plasma triglycerides were measured by the standard clinical method (enzymatic method) with a Hitachi 704 Chemistry Analyzer, in compliance with
the standardization and surveillance programs of the Centers for Disease Control and Prevention Laboratory (Atlanta, GA) (24,25). This enzymatic method uses cholesterol esterase and
cholesterol oxidase and overestimates plasma cholesterol concentrations from children with SLOS because dehydrocholesterols are substrates for cholesterol oxidase and contribute to the
resulting value (26). In plasma, the sum of the sterols (measured individually by gas chromatographic method) was similar to the total sterols (measured by enzymatic method) at baseline and
after dietary cholesterol (_R_2 = 0.98). Therefore, we assumed for subsequent analyses by the enzymatic method that each value represented “total sterols,” that is, cholesterol, 7DHC, and
8DHC. We measured the concentrations of individual sterols (cholesterol, 7DHC, and 8DHC) in each lipoprotein fraction (LDL, HDL, and VLDL) after separation by ultracentrifugation (24) (_n_ =
2). This ultracentrifugation step requires more blood than is routinely obtained in these small children, so we were able to do this only with two children. Both children were receiving
dietary cholesterol at the time of measurement. In all of the children, we measured the concentrations of individual sterols in plasma. These individual plasma sterol concentrations were
measured by capillary-column gas chromatography with a CP-Wax 57 column (25 M, 0.32 mm ID; Chrompack Co., Raritan, NJ) (5,20). Internal standard (5α-cholestane) and an authentic standard of
the cholesterol were used for calibration. In the two children, the distribution of individual sterols in each lipoprotein was the same as the distribution in plasma. Assuming that this was
the case for all of the children, we calculated the concentrations of cholesterol, 7DHC, and 8DHC in each lipoprotein fraction. For example, the concentration of cholesterol in LDL equals
the ratio of cholesterol to total sterols in plasma sample (gas chromatographic method) multiplied by the total sterol concentration in LDL (enzymatic method). Data are expressed as means ±
SEM. Statistical analysis of differences between means was determined using a two-tailed _t_ test for paired samples. APOE GENOTYPING. The apoE gene has three alleles, ε2, ε3, and ε4, coding
for the three major isoforms (E2, E3, and E4) of this protein. The alleles were identified by PCR amplification of nucleotides 2823–3093 followed by restriction endonuclease digestion with
_Hha_I. The six possible genotypes were determined from the sizes of the DNA fragments (27). RESULTS EFFECT OF DIETARY CHOLESTEROL ON LIPOPROTEINS AND TOTAL STEROLS. By the enzymatic method,
we measured total sterols in plasma (16 children) and total sterols in each lipoprotein fraction (12 children). Measurements that were taken at baseline (after 3 wk of an essentially
cholesterol-free diet) were compared with measurements after 6–19 mo of dietary cholesterol. The total sterols in plasma increased from 2.22 to 3.10 mM (_p_ < 0.002; Table 3). Six
children received alternative sources of cholesterol instead of egg yolk during some or all of the supplemental cholesterol period. The total sterols in this subgroup increased from 2.38 ±
0.28 to 3.26 ± 0.28 mM. Total sterols in LDL (enzymatic method) increased significantly (from 0.98 to 1.52 mM; _p_ < 0.02) in parallel with the increase in total plasma sterols. HDL
increased similarly from 0.72 to 0.92 mM (_p_ < 0.03). There were no changes in total sterols in VLDL (0.49 to 0.58 mM; _p_ = 0.48) or in plasma triglyceride concentrations (1.11 to 1.24
mM; _p_ = 0.49) in response to the dietary cholesterol. Under normal conditions, changes in dietary cholesterol do not affect VLDL cholesterol or plasma triglyceride concentrations (28). In
children 5–9 y of age, the normal triglyceride concentration in fasting plasma ranges from 31 to 104 mg/dL (29). Our values for triglycerides are slightly higher, likely because fasting
samples could not be obtained from infants. EFFECT OF DIETARY CHOLESTEROL ON PLASMA CONCENTRATIONS OF CHOLESTEROL, 7DHC, AND 8DHC. To determine whether the increase in total sterols resulted
specifically from an increase in the cholesterol component of plasma, we measured plasma cholesterol, 7DHC, and 8DHC concentrations in 16 SLOS children at baseline (after 3 wk of
essentially cholesterol-free diet) and after 6–19 mo of dietary cholesterol. Dietary cholesterol raised plasma cholesterol from 1.78 to 2.67 mM (_p_ < 0.007; Table 4). Not only did the
concentration of plasma cholesterol increase, but also the ratio of cholesterol relative to the sum of all sterols increased from 0.79 to 0.84. Dietary cholesterol did not significantly
decrease the mean plasma 7DHC (Table 4) or 8DHC (0.23–0.21 mM and 0.19–0.20 mM, respectively). At baseline, there was no correlation between the concentrations of cholesterol and 7DHC. After
dietary cholesterol, however, there was a significant negative correlation (_R_2 = 0.62, _p_ < 0.01; Fig. 1). Children who responded to the dietary cholesterol with the greatest increase
in plasma cholesterol concentrations tended to obtain the lowest 7DHC concentrations. DISTRIBUTION OF STEROLS WITHIN EACH LIPOPROTEIN FRACTION. The increase in total sterols in LDL and HDL
could result from an increase either in cholesterol or in the precursor sterols 7DHC or 8DHC. The plasma samples from two children had an adequate volume to separate the VLDL, LDL, and HDL
by ultracentrifugation. We then measured the individual sterol concentrations (cholesterol, 7DHC, and 8DHC) by gas chromatographic method in each fraction. We compared the distribution of
individual sterols in each lipoprotein with the distribution of individual sterols measured in plasma (Fig. 2). The concentrations of total sterols in each of these two children (subjects 7
and 9) were different from one another (2.43 and 1.24 mM, respectively). In addition, the distribution of cholesterol relative to the total sterols in plasma was different in these two
children (90 and 70%, respectively). For each child individually, however, the distribution of cholesterol, 7DHC, and 8DHC (relative to total sterols) in each lipoprotein fraction was the
same as the distribution in plasma (Fig. 2). Assuming that this is true for all cases, we calculated the concentration of cholesterol in LDL and HDL for each child (_n_ = 12). Results showed
that LDL cholesterol increased from 0.73 ± 0 0.13 to 1.31 ± 0.18 mM (_p_ < 0.02) and HDL cholesterol increased from 0.57 ± 0.05 to 0.79 ± 0.08 mM (_p_ < 0.03). The concentrations of
7DHC in LDL (0.10 mM and 0.11 mM) or 8DHC in LDL (0.09 and 0.10 mM) did not change. LACK OF EFFECT OF APOE GENOTYPE ON PLASMA CHOLESTEROL AND ON EFFICACY OF DIETARY CHOLESTEROL. Plasma
sterols in SLOS children varied widely at baseline and after dietary cholesterol. Of the three isoforms of apoE, the E4 isoform is associated with increased concentration of plasma
cholesterol (30). We determined whether apoE genotype could account for some of this variability. Three of the SLOS children had the 3/4 apoE genotype, whereas nine had the 3/3 genotype.
Plasma cholesterol concentrations, however, were similar in SLOS children with either genotype at baseline (1.74 _versus_ 1.80 mM; _p_ = 0.90) and after 6–19 mo of dietary cholesterol (2.62
_versus_ 2.81 mM; _p_ = 0.80; 3/4 and 3/3, respectively). DISCUSSION Cholesterol deficiency is characteristic of SLOS and may be a major contributor to the characteristic clinical features.
Cholesterol is an essential component of cell membranes and nervous system neurons and myelin, as well as serving as the substrate for important biologic compounds, including bile acids and
steroid hormones. Recently, cholesterol was shown to be an essential component of the lipid rafts involved in intracellular trafficking of proteins and lipids (31) and cell surface signaling
proteins (32). In addition, cholesterol has been shown to promote CNS synaptogenesis (33). Cholesterol also plays a critical role during fetal development. The cholesterol molecule is a
covalently bound component of active hedgehog proteins, a family of embryonic signaling proteins (34). Cholesterol deficiency could alter the function of the hedgehog signaling pathway. In
SLOS, some of the pathologic characteristics may also be due to accumulation of cholesterol precursors. For example, accumulation of 7DHC in the brain has been associated with impaired
learning in rats (35). 7DHC has also been shown to be teratogenic in rat embryos (36). Therefore, treatment for SLOS that normalizes plasma and tissue cholesterol levels while decreasing
accumulation of potentially toxic cholesterol intermediates and metabolites is sought. Dietary cholesterol has been considered a potential treatment. Lipoproteins are essential for transport
of dietary cholesterol from the intestines to the other tissues of the body as well as for cellular uptake of cholesterol. We measured total sterols in each lipoprotein fraction in 12
children. The total sterols significantly increased in LDL and HDL in response to dietary cholesterol. The ratios of noncholesterol sterols to cholesterol in serum do not always predict the
ratios in the different lipoproteins (37). Therefore, in two children, the concentration of each sterol within each lipoprotein was measured to determine the distribution of the sterols in
lipoproteins. In these two children, the distribution of individual sterols within each lipoprotein fraction was shown to be the same as the distribution in plasma. We calculated the
concentration of each sterol in each lipoprotein fraction in 12 children using the assumption that plasma sterol distribution equaled lipoprotein sterol distribution. These results showed
that after dietary cholesterol, _1_) the cholesterol concentrations in LDL and HDL increased, and _2_) there was not a preferential increase of 7DHC or 8DHC in either LDL or HDL. The
increases in LDL and HDL cholesterol in these children in response to dietary cholesterol could provide a larger pool of cholesterol available for tissue uptake. It has been shown that
125I-HDL2 from plasma of patients with abetalipoproteinemia was bound, taken up, and degraded by cultured human fibroblasts (37a). This suggests that HDL in addition to LDL can transport
cholesterol to tissues. Kinetic studies will be needed to determine whether there is a corresponding increase in LDL and HDL cholesterol uptake by cholesterol-deficient tissues in SLOS. Only
two published studies have reported lipoprotein data in SLOS patients. Pierquin _et al._ (38) described one severely affected child. This child had low concentrations of total sterols in
LDL (13 mg/dL) and HDL (8 mg/dL) and a low concentration of apoA-I (67 mg/dL), the major protein of HDL, as compared with normal ranges. Behulova _et al._ (39) performed similar measurements
in eight children who had SLOS and were not receiving supplemental or dietary cholesterol. Five of the children were severely affected, and three had less severe clinical features. All
eight children studied had low concentrations of total sterols in LDL (range: 0–1.18 mM) and HDL (0.13–0.84 mM) and low concentrations of apoA-I (<0.12–0.94 g/dL). The concentrations of
total sterols in VLDL were normal or above normal, and the concentrations of apoB were normal or borderline low. Neither the effect of dietary cholesterol on lipoproteins nor the
distribution of the individual sterols (in lipoprotein fractions or in plasma) was measured in these two studies. The effect of dietary cholesterol on plasma sterols was similar to our
previous report (19). After 6–19 mo of dietary cholesterol with egg yolk (in most cases), the mean plasma cholesterol in children with SLOS significantly increased when compared with the
mean concentration at baseline. The increase in cholesterol (57%, _n_ = 16) found in the current study was similar to the increase in cholesterol (55%, _n_ = 7) after 1–3 mo of dietary
cholesterol in our earlier study (19). These percentage increases were greater than those increases seen in adults who were given high-cholesterol diets (40). After dietary cholesterol,
plasma cholesterol rose to above the fifth percentile in four children of the current study, 3.18 mM for normal children aged 5–9 y (29). Although 10 children showed a decrease in plasma
7DHC (range: 8–77%), six children had an increase or no change. After dietary cholesterol, the lowest concentration of 7DHC in the SLOS children, 0.04 mM, was still higher than normal,
0.00026 mM (41). The bioavailability of egg yolk cholesterol _versus_ crystalline cholesterol has not been reported recently. We chose to use egg yolk as the source of cholesterol in most
subjects because there is some evidence that it is more effective in raising plasma cholesterol concentrations. In normal adults, dietary egg yolk increased plasma cholesterol concentrations
more readily than crystalline cholesterol supplementation (69 _versus_ 19 mg/dL), even though the subjects consumed lower amounts of cholesterol in the form of dietary egg yolks (475–1425
mg/d) than the amounts consumed as cholesterol in the crystalline form (1200–3600 mg/d) (42). In this present study, the increases in plasma concentrations were similar in both groups.
However, the number of children is too small to determine whether the source of cholesterol made a difference in the plasma sterol response. The absorption of cholesterol from the intestine
is complex (43) and may be dependent on many factors, including emulsification, micelle formation (44), and cholesterol transporters (45,46). Dietary cholesterol presumably would lower
plasma 7DHC and 8DHC in SLOS by inhibiting hepatic hydroxymethyl glutaryl (HMG) CoA reductase through feedback inhibition of this rate-limiting enzyme. We have shown that dietary cholesterol
(for 2 mo or 2 y) decreased HMG CoA reductase activity as measured by the amount of mevalonate in 24-h urine samples. Only after 2 y, however, was there a decrease in plasma 7DHC (_n_ = 4)
(19). These results and the wide range of responses of plasma 7DHC in our current study reflect the complex regulation of the cholesterol biosynthetic pathway. CONCLUSION This is the first
report of the effect of dietary cholesterol on sterols in lipoproteins in children with SLOS and of the distribution of cholesterol, 7DHC, and 8DHC in lipoproteins. Increase of total sterols
in plasma in response to dietary cholesterol was paralleled by increases of total sterols in LDL and HDL. Calculated concentrations of cholesterol in LDL and HDL also increased. This bodes
well for potential therapy of SLOS, because the increased LDL and HDL cholesterol provides a larger pool of cholesterol for tissue uptake. The plasma 7DHC concentrations did not return to
normal in the current study. Although dietary cholesterol inhibits HMG CoA reductase in SLOS (19), further inhibition, perhaps by statin drugs, may be necessary to normalize the 7DHC.
ABBREVIATIONS * apoE: apolipoprotein E * DHCR7: 7-dehydrocholesterol Δ7-reductase * HMG: hepatic hydroxymethyl glutaryl * SLOS: Smith-Lemli-Opitz syndrome * 7DHC: 7-dehydrocholesterol *
8DHC: 8-dehydrocholesterol REFERENCES * Smith DW, Lemli L, Opitz JM 1964 A newly recognized syndrome of multiple congenital anomalies. _J Pediatr_ 64: 210–217 Article CAS Google Scholar *
Irons M, Elias ER, Salen G, Tint GS, Batta AK 1993 Defective cholesterol biosynthesis in Smith-Lemli-Opitz syndrome. _Lancet_ 341: 1414 Article CAS Google Scholar * Tint GS, Irons M,
Elias ER, Batta AK, Frieden R, Chen TS, Salen G 1994 Defective cholesterol biosynthesis associated with the Smith-Lemli-Opitz syndrome. _N Engl J Med_ 330: 107–113 Article CAS Google
Scholar * Shefer S, Salen G, Batta AK, Honda A, Tint GS, Irons M, Elias ER, Chen TC, Holick MF 1995 Markedly inhibited 7-dehydrocholesterol-delta 7-reductase activity in liver microsomes
from Smith-Lemli-Opitz homozygotes. _J Clin Invest_ 96: 1779–1785 Article CAS Google Scholar * Wassif CA, Maslen C, Kachilele-Linjewile S, Lin D, Linck LM, Connor WE, Steiner RD, Porter
FD 1998 Mutations in the human sterol delta7-reductase gene at 11q12–13 cause Smith-Lemli-Opitz syndrome. _Am J Hum Genet_ 63: 55–62 Article CAS Google Scholar * Waterham HR, Wijburg FA,
Hennekam RC, Vreken P, Poll-The BT, Dorland L, Duran M, Jira PE, Smeitink JA, Wevers RA, Wanders RJ 1998 Smith-Lemli-Opitz syndrome is caused by mutations in the 7-dehydrocholesterol
reductase gene. _Am J Hum Genet_ 63: 329–338 Article CAS Google Scholar * Fitzky BU, Witsch-Baumgartner M, Erdel M, Lee JN, Paik YK, Glossmann H, Utermann G, Moebius FF 1998 Mutations in
the Delta7-sterol reductase gene in patients with the Smith-Lemli-Opitz syndrome. _Proc Natl Acad Sci USA_ 95: 8181–8186 Article CAS Google Scholar * Waterham HR, Wanders RJ 2000
Biochemical and genetic aspects of 7-dehydrocholesterol reductase and Smith-Lemli-Opitz syndrome. _Biochim Biophys Acta_ 1529: 340–356 Article CAS Google Scholar * Nowaczyk MJ, Nakamura
LM, Waye JS 2001 DHCR7 and Smith-Lemli-Opitz syndrome. _Clin Invest Med_ 24: 311–317 CAS PubMed Google Scholar * Battaile KP, Steiner RD 2000 Smith-Lemli-Opitz syndrome: the first
malformation syndrome associated with defective cholesterol synthesis. _Mol Genet Metab_ 71: 154–162 Article CAS Google Scholar * Irons M, Elias ER, Tint GS, Salen G, Frieden R, Buie TM,
Ampola M 1994 Abnormal cholesterol metabolism in the Smith-Lemli-Opitz syndrome: report of clinical and biochemical findings in four patients and treatment in one patient. _Am J Med Genet_
50: 347–352 Article CAS Google Scholar * Nwokoro NA, Hyde B, Mulvihill JJ 1994 Smith-Lemli-Opitz syndrome: biochemical before clinical diagnosis; early dietary management. _Am J Med
Genet_ 50: 375–376 Article CAS Google Scholar * Ullrich K, Koch HG, Meschede D, Flotmann U, Seedorf U 1996 Smith-Lemli-Opitz syndrome: treatment with cholesterol and bile acids.
_Neuropediatrics_ 27: 111–112 Article CAS Google Scholar * van Rooij A, Nijenhuis AA, Wijburg FA, Schutgens RB 1997 Highly increased CSF concentrations of cholesterol precursors in
Smith-Lemli-Opitz syndrome. _J Inherit Metab Dis_ 20: 578–580 Article CAS Google Scholar * Nwokoro NA, Mulvihill JJ 1997 Cholesterol and bile acid replacement therapy in children and
adults with Smith-Lemli-Opitz (SLO/RSH) syndrome. _Am J Med Genet_ 68: 315–321 Article CAS Google Scholar * Irons M, Elias ER, Abuelo D, Bull MJ, Greene CL, Johnson VP, Keppen L, Schanen
C, Tint GS, Salen G 1997 Treatment of Smith-Lemli-Opitz syndrome: results of a multicenter trial. _Am J Med Genet_ 68: 311–314 Article CAS Google Scholar * Elias ER, Irons MB, Hurley AD,
Tint GS, Salen G 1997 Clinical effects of cholesterol supplementation in six patients with the Smith-Lemli-Opitz syndrome (SLOS). _Am J Med Genet_ 68: 305–310 Article CAS Google Scholar *
Linck LM, Lin DS, Flavell D, Connor WE, Steiner RD 2000 Cholesterol supplementation with egg yolk increases plasma cholesterol and decreases plasma 7-dehydrocholesterol in Smith-Lemli-Opitz
syndrome. _Am J Med Genet_ 93: 360–365 Article CAS Google Scholar * Pappu AS, Steiner RD, Connor SL, Flavell DP, Lin DS, Hatcher L, Illingworth DR, Connor WE 2002 Feedback inhibition of
the cholesterol biosynthetic pathway in patients with Smith-Lemli-Opitz syndrome as demonstrated by urinary mevalonate excretion. _J Lipid Res_ 43: 1661–1669 Article CAS Google Scholar *
Steiner RD, Linck LM, Flavell DP, Lin DS, Connor WE 2000 Sterol balance in the Smith-Lemli-Opitz syndrome. Reduction in whole body cholesterol synthesis and normal bile acid production. _J
Lipid Res_ 41: 1437–1447 CAS PubMed Google Scholar * Linck LM, Hayflick SJ, Lin DS, Battaile KP, Ginat S, Burlingame T, Gibson KM, Honda M, Honda A, Salen G, Tint GS, Connor WE, Steiner
RD 2000 Fetal demise with Smith-Lemli-Opitz syndrome confirmed by tissue sterol analysis and the absence of measurable 7-dehydrocholesterol Delta(7)-reductase activity in chorionic villi.
_Prenat Diagn_ 20: 238–240 Article CAS Google Scholar * Krakowiak PA, Nwokoro NA, Wassif CA, Battaile KP, Nowaczyk MJ, Connor WE, Maslen C, Steiner RD, Porter FD 2000 Mutation analysis
and description of sixteen RSH/Smith-Lemli-Opitz syndrome patients: polymerase chain reaction-based assays to simplify genotyping. _Am J Med Genet_ 94: 214–227 Article CAS Google Scholar
* Martin A, Koenig K, Scahill L, Tierney E, Porter FD, Nwokoro NA 2001 Smith-Lemli-Opitz syndrome. _J Am Acad Child Adolesc Psychiatry_ 40: 506–507 Article CAS Google Scholar * Lipid
Research Clinics Program, 1982 Manual of Laboratory Operations, Lipid and Lipoprotein Analysis, 2nd Ed. _Department of Health and Human Services (NIH)_, Bethesda * Wiebe DA, Bernert JT Jr
1984 Influence of incomplete cholesteryl ester hydrolysis on enzymic measurements of cholesterol. _Clin Chem_ 30: 352–356 CAS PubMed Google Scholar * Jira PE, de Jong JG, Janssen-Zijlstra
FS, Wendel U, Wevers RA 1997 Pitfalls in measuring plasma cholesterol in the Smith-Lemli-Opitz syndrome. _Clin Chem_ 43: 129–133 CAS PubMed Google Scholar * Tsukamoto K, Watanabe T,
Matsushima T, Kinoshita M, Kato H, Hashimoto Y, Kurokawa K, Teramoto T 1993 Determination by PCR-RFLP of apo E genotype in a Japanese population. _J Lab Clin Med_ 121: 598–602 CAS PubMed
Google Scholar * Raymond TL, Connor WE, Lin DS, Warner S, Fry MM, Connor SL 1977 The interaction of dietary fibers and cholesterol upon the plasma lipids and lipoproteins, sterol balance,
and bowel function in human subjects. _J Clin Invest_ 60: 1429–1437 Article CAS Google Scholar * Kwiterovich PO Jr 1991 Plasma lipid and lipoprotein levels in childhood. _Ann NY Acad Sci_
623: 90–107 Article CAS Google Scholar * Utermann G, Kindermann I, Kaffarnik H, Steinmetz A 1984 Apolipoprotein E phenotypes and hyperlipidemia. _Hum Genet_ 65: 232–236 Article CAS
Google Scholar * Ikonen E 2001 Roles of lipid rafts in membrane transport. _Curr Opin Cell Biol_ 13: 470–477 Article CAS Google Scholar * Kabouridis PS, Janzen J, Magee AL, Ley SC 2000
Cholesterol depletion disrupts lipid rafts and modulates the activity of multiple signaling pathways in T lymphocytes. _Eur J Immunol_ 30: 954–963 Article CAS Google Scholar * Mauch DH,
Nagler K, Schumacher S, Goritz C, Muller EC, Otto A, Pfrieger FW 2001 CNS synaptogenesis promoted by glia-derived cholesterol. _Science_ 294: 1354–1357 Article CAS Google Scholar * Kelley
RI, Hennekam RC 2000 The Smith-Lemli-Opitz syndrome. _J Med Genet_ 37: 321–335 Article CAS Google Scholar * Xu G, Servatius RJ, Shefer S, Tint GS, O'Brien WT, Batta AK, Salen G 1998
Relationship between abnormal cholesterol synthesis and retarded learning in rats. _Metabolism_ 47: 878–882 Article CAS Google Scholar * Roux C, Wolf C, Mulliez N, Gaoua W, Cormier V,
Chevy F, Citadelle D 2000 Role of cholesterol in embryonic development. _Am J Clin Nutr_ 71: 1270S–1279S Article CAS Google Scholar * Ketomaki A, Gylling H, Siimes MA, Vuorio A, Miettinen
TA 2003 Squalene and noncholesterol sterols in serum and lipoproteins of children with and without familial hypercholesterolemia. _Pediatr Res_ 53: 648–653 Article Google Scholar *
Illingworth DR, Alam NA, Sundberg EE, Hagemenas FC, Layman DL 1983 Regulation of low density lipoprotein receptors by plasma lipoproteins from patients with abetalipoproteineinemia. _Proc
Natl Acad Sci USA_ 80: 3475–3479 Article CAS Google Scholar * Pierquin G, Peeters P, Roels F, Vamos E, Brucher JM, Tint GS, Honda A, Van Regemorter N 1995 Severe Smith-Lemli-Opitz
syndrome with prolonged survival and lipid abnormalities. _Am J Med Genet_ 56: 276–280 Article CAS Google Scholar * Behulova D, Bzduch V, Skodova J, Dello Russo A, Corso G, Ponec J,
Kasanicka A 2000 Serum lipids and apolipoproteins in children with the Smith-Lemli-Opitz syndrome. _J Inherit Metab Dis_ 23: 413–415 Article CAS Google Scholar * Connor WE, Connor SL 2002
Dietary cholesterol and coronary heart disease. _Curr Atheroscler Rep_ 4: 425–432 Article Google Scholar * Cunniff C, Kratz LE, Moser A, Natowicz MR, Kelley RI 1997 Clinical and
biochemical spectrum of patients with RSH/Smith-Lemli-Opitz syndrome and abnormal cholesterol metabolism. _Am J Med Genet_ 68: 263–269 Article CAS Google Scholar * Connor WE, Hodges RE,
Bleiler RE 1961 The serum lipids in men receiving high cholesterol and cholesterol-free diets. _J Clin Invest_ 40: 894–901 Article CAS Google Scholar * Ros E 2000 Intestinal absorption of
triglyceride and cholesterol. Dietary and pharmacological inhibition to reduce cardiovascular risk. _Atherosclerosis_ 151: 357–379 Article CAS Google Scholar * Yao L, Heubi JE, Buckley
DD, Fierra H, Setchell KD, Granholm NA, Tso P, Hui DY, Woollett LA 2002 Separation of micelles and vesicles within lumenal aspirates from healthy humans: solubilization of cholesterol after
a meal. _J Lipid Res_ 43: 654–660 CAS PubMed Google Scholar * Altmann SW, Davis HR Jr, Zhu LJ, Yao X, Hoos LM, Tetzloff G, Iyer SP, Maguire M, Golovko A, Zeng M, Wang L, Murgolo N,
Graziano MP 2004 Niemann-Pick C1 Like 1 protein is critical for intestinal cholesterol absorption. _Science_ 303: 1201–1204 Article CAS Google Scholar * Plat J, Mensink RP 2002 Increased
intestinal ABCA1 expression contributes to the decrease in cholesterol absorption after plant stanol consumption. _FASEB J_ 16: 1248–1253 Article CAS Google Scholar Download references
ACKNOWLEDGEMENTS We thank Gary Sexton, Ph.D., for assistance with statistical analysis and Pam Smith and Carol Marsh for lipoprotein separation. We thank the children and families for
participation in the study and the staff of the Oregon Health & Science University General Clinical Research Center. We thank Sandra Banta-Wright, R.N., M.S.N., N.N.P., Daniel Marks,
M.D., and numerous other health care providers who assisted in the care of these children. We thank the many health care providers who kindly referred these children to us. AUTHOR
INFORMATION AUTHORS AND AFFILIATIONS * Department of Pediatrics, Department of Medicine, OR Health & Science University, Portland, 97239, OR Louise S Merkens & Robert D Steiner *
Division of Endocrinology, Department of Medicine, Metabolism and Nutrition, OR Health & Science University, Portland, 97239, OR William E Connor, Don S Lin & Donna P Flavell *
Department of Genetics, Kaiser Permanente Northwest, Portland, 97227, OR Leesa M Linck * Department of Molecular and Medical Genetics, Child Development and Rehabilitation Center,
Doernbecher Children's Hospital, OR Health & Science University, Portland, 97239, OR Leesa M Linck & Robert D Steiner Authors * Louise S Merkens View author publications You can
also search for this author inPubMed Google Scholar * William E Connor View author publications You can also search for this author inPubMed Google Scholar * Leesa M Linck View author
publications You can also search for this author inPubMed Google Scholar * Don S Lin View author publications You can also search for this author inPubMed Google Scholar * Donna P Flavell
View author publications You can also search for this author inPubMed Google Scholar * Robert D Steiner View author publications You can also search for this author inPubMed Google Scholar
CORRESPONDING AUTHOR Correspondence to Robert D Steiner. ADDITIONAL INFORMATION Supported by grants from the National Heart, Lung, and Blood Institute (PHS HL-64618 and HL-073980); National
Center for Research Resources (PHS 5 M01 RR000334 and PHS 3 M01 RR003344-33S3); National Institute for Child Health and Human Development, OR Child Health Research Center (PHS 5P30
HD33703-04); the American Academy of Pediatrics Section on Genetics and Birth Defects; the Collins Foundation; and the Smith-Lemli-Opitz Advocacy and Exchange. R.D.S. is a Clinical Associate
Physician Investigator of the Oregon Health & Science University General Clinical Research Center. RIGHTS AND PERMISSIONS Reprints and permissions ABOUT THIS ARTICLE CITE THIS ARTICLE
Merkens, L., Connor, W., Linck, L. _et al._ Effects of Dietary Cholesterol on Plasma Lipoproteins in Smith-Lemli-Opitz Syndrome. _Pediatr Res_ 56, 726–732 (2004).
https://doi.org/10.1203/01.PDR.0000141522.14177.4F Download citation * Received: 20 January 2004 * Accepted: 07 July 2004 * Issue Date: 01 November 2004 * DOI:
https://doi.org/10.1203/01.PDR.0000141522.14177.4F SHARE THIS ARTICLE Anyone you share the following link with will be able to read this content: Get shareable link Sorry, a shareable link
is not currently available for this article. Copy to clipboard Provided by the Springer Nature SharedIt content-sharing initiative