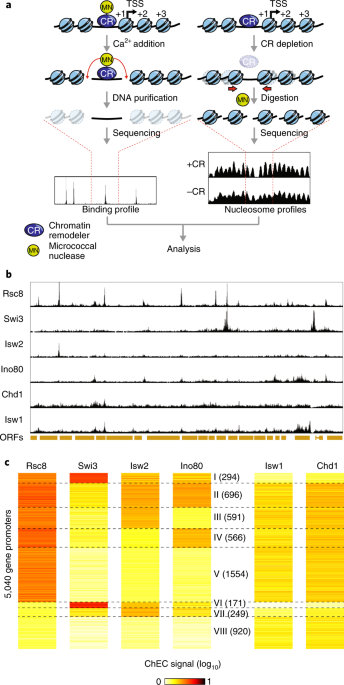
- Select a language for the TTS:
- UK English Female
- UK English Male
- US English Female
- US English Male
- Australian Female
- Australian Male
- Language selected: (auto detect) - EN
Play all audios:
ABSTRACT Precise nucleosome organization at eukaryotic promoters is thought to be generated by multiple chromatin remodeler (CR) enzymes and to affect transcription initiation. Using an
integrated analysis of chromatin remodeler binding and nucleosome occupancy following rapid remodeler depletion, we investigated the interplay between these enzymes and their impact on
transcription in yeast. We show that many promoters are affected by multiple CRs that operate in concert or in opposition to position the key transcription start site (TSS)-associated +1
nucleosome. We also show that nucleosome movement after CR inactivation usually results from the activity of another CR and that in the absence of any remodeling activity, +1 nucleosomes
largely maintain their positions. Finally, we present functional assays suggesting that +1 nucleosome positioning often reflects a trade-off between maximizing RNA polymerase recruitment and
minimizing transcription initiation at incorrect sites. Our results provide a detailed picture of fundamental mechanisms linking promoter nucleosome architecture to transcription
initiation. Access through your institution Buy or subscribe This is a preview of subscription content, access via your institution ACCESS OPTIONS Access through your institution Access
Nature and 54 other Nature Portfolio journals Get Nature+, our best-value online-access subscription $29.99 / 30 days cancel any time Learn more Subscribe to this journal Receive 12 print
issues and online access $209.00 per year only $17.42 per issue Learn more Buy this article * Purchase on SpringerLink * Instant access to full article PDF Buy now Prices may be subject to
local taxes which are calculated during checkout ADDITIONAL ACCESS OPTIONS: * Log in * Learn about institutional subscriptions * Read our FAQs * Contact customer support SIMILAR CONTENT
BEING VIEWED BY OTHERS ENHANCER–PROMOTER CONTACT FORMATION REQUIRES RNAPII AND ANTAGONIZES LOOP EXTRUSION Article 03 April 2023 RULER ELEMENTS IN CHROMATIN REMODELERS SET NUCLEOSOME ARRAY
SPACING AND PHASING Article Open access 28 May 2021 RNA POLYMERASE II DYNAMICS SHAPE ENHANCER–PROMOTER INTERACTIONS Article 10 July 2023 DATA AVAILABILITY All sequencing data generated in
this study were submitted to the GEO database under accession code GSE115412 (for ChEC-seq, MNase-seq and ChIP-seq) and Series GSE114589 (TSS-seq). Source data for Fig. 2c and 2d are
available online. CODE AVAILABILITY Peak-calling software is available at https://gitlab.unige.ch/JLFalcone/peakmatic. REFERENCES * Lai, W. K. M. & Pugh, B. F. Understanding nucleosome
dynamics and their links to gene expression and DNA replication. _Nat. Rev. Mol. Cell Biol._ 18, 548–562 (2017). Article CAS Google Scholar * Brahma, S. & Henikoff, S. RSC-associated
subnucleosomes define MNase-sensitive promoters in yeast. _Mol. Cell_ 73, 238–249.e3 (2019). Article CAS Google Scholar * Henikoff, J. G., Belsky, J. A., Krassovsky, K., MacAlpine, D. M.
& Henikoff, S. Epigenome characterization at single base-pair resolution. _Proc. Natl Acad. Sci. USA_ 108, 18318–18323 (2011). Article CAS Google Scholar * Kent, N. A., Adams, S.,
Moorhouse, A. & Paszkiewicz, K. Chromatin particle spectrum analysis: a method for comparative chromatin structure analysis using paired-end mode next-generation DNA sequencing. _Nucleic
Acids Res._ 39, e26 (2011). Article CAS Google Scholar * Kubik, S. et al. Nucleosome stability distinguishes two different promoter types at all protein-coding genes in yeast. _Mol.
Cell_ 60, 422–434 (2015). Article CAS Google Scholar * Weiner, A., Hughes, A., Yassour, M., Rando, O. J. & Friedman, N. High-resolution nucleosome mapping reveals
transcription-dependent promoter packaging. _Genome Res._ 20, 90–100 (2010). Article CAS Google Scholar * Xi, Y., Yao, J., Chen, R., Li, W. & He, X. Nucleosome fragility reveals novel
functional states of chromatin and poises genes for activation. _Genome Res._ 21, 718–724 (2011). Article CAS Google Scholar * Rhee, H. S. & Pugh, B. F. Genome-wide structure and
organization of eukaryotic pre-initiation complexes. _Nature_ 483, 295–301 (2012). Article CAS Google Scholar * Kubik, S. et al. Sequence-directed action of RSC remodeler and general
regulatory factors modulates +1 nucleosome position to facilitate transcription. _Mol. Cell_ 71, 89–102.e5 (2018). Article CAS Google Scholar * Clapier, C. R., Iwasa, J., Cairns, B. R.
& Peterson, C. L. Mechanisms of action and regulation of ATP-dependent chromatin-remodelling complexes. _Nat. Rev. Mol. Cell Biol._ 18, 407–422 (2017). Article CAS Google Scholar *
Badis, G. et al. A library of yeast transcription factor motifs reveals a widespread function for Rsc3 in targeting nucleosome exclusion at promoters. _Mol. Cell_ 32, 878–887 (2008). Article
CAS Google Scholar * Ganguli, D., Chereji, R. V., Iben, J. R., Cole, H. A. & Clark, D. J. RSC-dependent constructive and destructive interference between opposing arrays of phased
nucleosomes in yeast. _Genome Res._ 24, 1637–1649 (2014). Article CAS Google Scholar * Krietenstein, N. et al. Genomic nucleosome organization reconstituted with pure proteins. _Cell_
167, 709–721 e12 (2016). Article CAS Google Scholar * Yen, K., Vinayachandran, V., Batta, K., Koerber, R. T. & Pugh, B. F. Genome-wide nucleosome specificity and directionality of
chromatin remodelers. _Cell_ 149, 1461–1473 (2012). Article CAS Google Scholar * Boeger, H., Griesenbeck, J., Strattan, J. S. & Kornberg, R. D. Nucleosomes unfold completely at a
transcriptionally active promoter. _Mol. Cell_ 11, 1587–1598 (2003). Article CAS Google Scholar * Floer, M. et al. ARSC/nucleosome complex determines chromatin architecture and
facilitates activator binding. _Cell_ 141, 407–418 (2010). Article CAS Google Scholar * Klein-Brill, A., Joseph-Strauss, D., Appleboim, A. & Friedman, N. Dynamics of chromatin and
transcription during transient depletion of the rsc chromatin remodeling complex. _Cell Rep._ 26, 279–292.e5 (2019). Article CAS Google Scholar * Gkikopoulos, T. et al. A role for
Snf2-related nucleosome-spacing enzymes in genome-wide nucleosome organization. _Science_ 333, 1758–1760 (2011). Article CAS Google Scholar * Whitehouse, I., Rando, O. J., Delrow, J.
& Tsukiyama, T. Chromatin remodelling at promoters suppresses antisense transcription. _Nature_ 450, 1031–1035 (2007). Article CAS Google Scholar * Zentner, G. E., Tsukiyama, T. &
Henikoff, S. ISWI and CHD chromatin remodelers bind promoters but act in gene bodies. _PLoS Genet_ 9, e1003317 (2013). Article CAS Google Scholar * Kobor, M. S. et al. A protein complex
containing the conserved Swi2/Snf2-related ATPase Swr1p deposits histone variant H2A.Z into euchromatin. _PLoS Biol._ 2, E131 (2004). Article Google Scholar * Krogan, N. J. et al. A Snf2
family ATPase complex required for recruitment of the histone H2A variant Htz1. _Mol. Cell_ 12, 1565–1576 (2003). Article CAS Google Scholar * Mizuguchi, G. et al. ATP-driven exchange of
histone H2AZ variant catalyzed by SWR1 chromatin remodeling complex. _Science_ 303, 343–348 (2004). Article CAS Google Scholar * Mohd-Sarip, A. et al. DOC1-dependent recruitment of NURD
reveals antagonism with SWI/SNF during epithelial-mesenchymal transition in oral cancer cells. _Cell Rep._ 20, 61–75 (2017). Article CAS Google Scholar * Morris, S. A. et al. Overlapping
chromatin-remodeling systems collaborate genome wide at dynamic chromatin transitions. _Nat. Struct. Mol. Biol._ 21, 73–81 (2014). Article CAS Google Scholar * Parnell, T. J., Schlichter,
A., Wilson, B. G. & Cairns, B. R. The chromatin remodelers RSC and ISW1 display functional and chromatin-based promoter antagonism. _eLife_ 4, e06073 (2015). Article Google Scholar *
Tomar, R. S., Psathas, J. N., Zhang, H., Zhang, Z. & Reese, J. C. A novel mechanism of antagonism between ATP-dependent chromatin remodeling complexes regulates RNR3 expression. _Mol.
Cell Biol._ 29, 3255–3265 (2009). Article CAS Google Scholar * El-Brolosy, M. A. & Stainier, D. Y. R. Genetic compensation: A phenomenon in search of mechanisms. _PLoS Genet._ 13,
e1006780 (2017). Article Google Scholar * Zentner, G. E., Kasinathan, S., Xin, B., Rohs, R. & Henikoff, S. ChEC-seq kinetics discriminates transcription factor binding sites by DNA
sequence and shape in vivo. _Nat Commun._ 6, 8733 (2015). Article CAS Google Scholar * Haruki, H., Nishikawa, J. & Laemmli, U. K. The anchor-away technique: rapid, conditional
establishment of yeast mutant phenotypes. _Mol. Cell_ 31, 925–932 (2008). Article CAS Google Scholar * Bruzzone, M. J., Grunberg, S., Kubik, S., Zentner, G. E. & Shore, D. Distinct
patterns of histone acetyltransferase and mediator deployment at yeast protein-coding genes. _Genes Dev._ 32, 1252–1265 (2018). Article CAS Google Scholar * Churchman, L. S. &
Weissman, J. S. Nascent transcript sequencing visualizes transcription at nucleotide resolution. _Nature_ 469, 368–373 (2011). Article CAS Google Scholar * Morawska, M. & Ulrich, H.
D. An expanded tool kit for the auxin-inducible degron system in budding yeast. _Yeast_ 30, 341–351 (2013). Article CAS Google Scholar * van Bakel, H. et al. A compendium of nucleosome
and transcript profiles reveals determinants of chromatin architecture and transcription. _PLoS Genet._ 9, e1003479 (2013). Article Google Scholar * Rawal, Y. et al. SWI/SNF and RSC
cooperate to reposition and evict promoter nucleosomes at highly expressed genes in yeast. _Genes Dev._ 32, 695–710 (2018). Article CAS Google Scholar * Hughes, A. L. & Rando, O. J.
Mechanisms underlying nucleosome positioning in vivo. _Annu Rev. Biophys._ 43, 41–63 (2014). Article CAS Google Scholar * Kornberg, R. D. & Stryer, L. Statistical distributions of
nucleosomes: nonrandom locations by a stochastic mechanism. _Nucleic Acids Res._ 16, 6677–6690 (1988). Article CAS Google Scholar * Shivaswamy, S. & Iyer, V. R. Stress-dependent
dynamics of global chromatin remodeling in yeast: dual role for SWI/SNF in the heat shock stress response. _Mol. Cell Biol._ 28, 2221–2234 (2008). Article CAS Google Scholar * Challal, D.
et al. General regulatory factors control the fidelity of transcription by restricting non-coding and ectopic initiation. _Mol. Cell_ 72, 955–969 e7 (2018). Article CAS Google Scholar *
Dreos, R., Ambrosini, G. & Bucher, P. Influence of rotational nucleosome positioning on transcription start site selection in animal promoters. _PLoS Comput Biol._ 12, e1005144 (2016).
Article Google Scholar * Malabat, C., Feuerbach, F., Ma, L., Saveanu, C. & Jacquier, A. Quality control of transcription start site selection by nonsense-mediated-mRNA decay. _eLife_
4, e06722 (2015). * Fennessy, R. T. & Owen-Hughes, T. Establishment of a promoter-based chromatin architecture on recently replicated DNA can accommodate variable inter-nucleosome
spacing. _Nucleic Acids Res._ 44, 7189–7203 (2016). CAS PubMed PubMed Central Google Scholar * Vasseur, P. et al. Dynamics of nucleosome positioning maturation following genomic
replication. _Cell Rep._ 16, 2651–2665 (2016). Article CAS Google Scholar * Yadav, T. & Whitehouse, I. Replication-Coupled nucleosome assembly and positioning by ATP-dependent
chromatin-remodeling enzymes. _Cell Rep._ 15, 715–723 (2016). Article CAS Google Scholar * Ramachandran, S., Ahmad, K. & Henikoff, S. Capitalizing on disaster: Establishing chromatin
specificity behind the replication fork. _Bioessays_ https://doi.org/10.1002/bies.201600150 (2017). Article Google Scholar * Agalioti, T. et al. Ordered recruitment of chromatin modifying
and general transcription factors to the IFN-beta promoter. _Cell_ 103, 667–678 (2000). Article CAS Google Scholar * Nocetti, N. & Whitehouse, I. Nucleosome repositioning underlies
dynamic gene expression. _Genes Dev._ 30, 660–672 (2016). Article CAS Google Scholar * Flaus, A. & Owen-Hughes, T. Dynamic properties of nucleosomes during thermal and ATP-driven
mobilization. _Mol. Cell Biol._ 23, 7767–7779 (2003). Article CAS Google Scholar * Kassabov, S. R., Zhang, B., Persinger, J. & Bartholomew, B. SWI/SNF unwraps, slides, and rewraps the
nucleosome. _Mol. Cell_ 11, 391–403 (2003). Article CAS Google Scholar * Chaban, Y. et al. Structure of a RSC-nucleosome complex and insights into chromatin remodeling. _Nat. Struct.
Mol. Biol._ 15, 1272–1277 (2008). Article CAS Google Scholar * Dechassa, M. L. et al. Architecture of the SWI/SNF-nucleosome complex. _Mol. Cell Biol._ 28, 6010–6021 (2008). Article CAS
Google Scholar * Stockdale, C., Flaus, A., Ferreira, H. & Owen-Hughes, T. Analysis of nucleosome repositioning by yeast ISWI and Chd1 chromatin remodeling complexes. _J. Biol. Chem._
281, 16279–16288 (2006). Article CAS Google Scholar * Udugama, M., Sabri, A. & Bartholomew, B. The INO80 ATP-dependent chromatin remodeling complex is a nucleosome spacing factor.
_Mol. Cell Biol._ 31, 662–673 (2011). Article CAS Google Scholar * Bowman, G. D. & McKnight, J. N. Sequence-specific targeting of chromatin remodelers organizes precisely positioned
nucleosomes throughout the genome. _Bioessays_ 39, 1–8 (2017). Article CAS Google Scholar * Goldmark, J. P., Fazzio, T. G., Estep, P. W., Church, G. M. & Tsukiyama, T. The Isw2
chromatin remodeling complex represses early meiotic genes upon recruitment by Ume6p. _Cell_ 103, 423–433 (2000). Article CAS Google Scholar * McKnight, J. N., Tsukiyama, T. & Bowman,
G. D. Sequence-targeted nucleosome sliding in vivo by a hybrid Chd1 chromatin remodeler. _Genome Res._ 26, 693–704 (2016). Article CAS Google Scholar * Wu, A. C. K. et al. Repression of
divergent noncoding transcription by a sequence-specific transcription factor. _Mol. Cell_ 72, 942–954.e7 (2018). Article CAS Google Scholar * Yadon, A. N. et al. Chromatin remodeling
around nucleosome-free regions leads to repression of noncoding RNA transcription. _Mol. Cell Biol._ 30, 5110–5122 (2010). Article CAS Google Scholar * Hainer, S. J. et al. Suppression of
pervasive noncoding transcription in embryonic stem cells by esBAF. _Genes Dev._ 29, 362–378 (2015). Article CAS Google Scholar * David, F. P. et al. HTSstation: a web application and
open-access libraries for high-throughput sequencing data analysis. _PLoS One_ 9, e85879 (2014). Article Google Scholar * Khan, A. et al. JASPAR 2018: update of the open-access database of
transcription factor binding profiles and its web framework. _Nucleic Acids Res._ 46, D260–D266 (2018). Article CAS Google Scholar * Lerdrup, M., Johansen, J. V., Agrawal-Singh, S. &
Hansen, K. An interactive environment for agile analysis and visualization of ChIP-sequencing data. _Nat. Struct. Mol. Biol._ 23, 349–357 (2016). Article CAS Google Scholar Download
references ACKNOWLEDGEMENTS We thank M. Docquier and the iGE3 Genomics Platform (https://ige3.genomics.unige.ch/) at the University of Geneva for high-throughput sequencing services, N.
Roggli for expert assistance with data presentation and artwork, B. Albert for help with fluorescence microscopy, and all members of the Shore lab for comments and discussions throughout the
course of this work. M.J.B. was supported in part by an iGE3 PhD student fellowship. D.C. was supported by a fellowship from the Ligue Contre le Cancer. D.L. acknowledges support from the
Centre National de la Recherche Scientifique (CNRS), the Fondation pour la Recherche Medicale (FRM, programme équipes 2013), l’Agence National pour la Recherche (ANR, grant
ANR-16-CE12-0022-01 to D.L.), and the Labex Who Am I? (ANR-11-LABX-0071 et Idex ANR-11-IDEX-0005-02 to D.L.). D.S. acknowledges funding from the Swiss National Science Foundation (grant no.
31003A_170153) and the Republic and Canton of Geneva. AUTHOR INFORMATION Author notes * These authors contributed equally: Maria Jessica Bruzzone, Drice Challal AUTHORS AND AFFILIATIONS *
Department of Molecular Biology and Institute of Genetics and Genomics of Geneva (iGE3), Geneva, Switzerland Slawomir Kubik, Maria Jessica Bruzzone, Stefano Mattarocci & David Shore *
Institut Jacques Monod, CNRS-Université Paris Diderot, Paris, France Drice Challal & Domenico Libri * Ecole Polytechnique Fédérale de Lausanne (EPFL), Lausanne, Switzerland René Dreos
& Philipp Bucher Authors * Slawomir Kubik View author publications You can also search for this author inPubMed Google Scholar * Maria Jessica Bruzzone View author publications You can
also search for this author inPubMed Google Scholar * Drice Challal View author publications You can also search for this author inPubMed Google Scholar * René Dreos View author publications
You can also search for this author inPubMed Google Scholar * Stefano Mattarocci View author publications You can also search for this author inPubMed Google Scholar * Philipp Bucher View
author publications You can also search for this author inPubMed Google Scholar * Domenico Libri View author publications You can also search for this author inPubMed Google Scholar * David
Shore View author publications You can also search for this author inPubMed Google Scholar CONTRIBUTIONS Conceptualization: S.K., D.C., M.J.B., D.L. and D.S.; formal analysis: S.K., D.C.,
M.J.B., R.D., P.B., D.L. and D.S.; investigation: S.K., D.C., M.J.B. and S.M.; data curation: S.K., D.C., M.J.B., R.D., D.L. and P.B.; writing of original draft: S.K. and D.S.; funding
acquisition: D.S., D.L. and P.B.; resources: D.S., D.L. and P.B.; supervision: D.S., D.L. and P.B. CORRESPONDING AUTHOR Correspondence to David Shore. ETHICS DECLARATIONS COMPETING INTERESTS
The authors declare no competing interests. ADDITIONAL INFORMATION PEER REVIEW INFORMATION: Beth Moorefield was the primary editor on this article and managed its editorial process and peer
review in collaboration with the rest of the editorial team. PUBLISHER’S NOTE: Springer Nature remains neutral with regard to jurisdictional claims in published maps and institutional
affiliations. INTEGRATED SUPPLEMENTARY INFORMATION SUPPLEMENTARY FIGURE 1 CHARACTERIZATION OF REMODELER BINDING BY CHEC-SEQ. A, Growth assays (serial dilution “spot assays”) of the indicated
MNase-tagged chromatin remodeler strains on YPAD medium, compared to the wild-type parent strain (WT). Plates were photographed following 24 or 48 hrs incubation at 30oC. B, Distribution of
identified remodeler binding sites between different genomic features. C, Heatmaps displaying ChEC signal for every CR centered on the +1 nucleosome of 5,040 protein-coding genes, sorted
(top to bottom) by decreasing signal calculated in a region -250 to -50 bp from +1 nucleosome dyad. D, Grid representing Pearson correlation coefficients between ChEC signal for different
CRs calculated at all identified remodeler binding sites. E, Plots displaying average normalized ChEC signal for each CR calculated for all clusters. F, Average nucleosome occupancy in each
cluster. G, Boxplots displaying expression of genes belonging to each cluster measured either as RNAPII ChIP-seq signal in the gene body or NET-seq signal. H, Fraction of genes in each
cluster which contain a canonical TATA-box, dashed line indicates genomic average (~17%). SUPPLEMENTARY FIGURE 2 VERIFICATION AND CHARACTERIZATION OF REMODELER DEPLETION AND EFFECTS ON
NUCLEOSOME OCCUPANCY AND STABILITY. A, Fluorescence microscopy of cells bearing FRB-GFP fusions of Sth1, Snf2, Isw2 and Chd1; cells were treated with rapamycin for indicated times, fixed and
stained with DAPI. B, Western blotting (anti-myc antibodies) of cell lysates from an untagged strain and strains bearing Ino80-AID*-myc and Isw1-AID*-myc fusions treated with auxin for
indicated amount of time. C, Growth assays (serial dilution “spot assays”, as in Supplementary Fig. 1a) of the indicated anchor-away or AID depletion strains on YPAD medium. “WT” indicates
the parental anchor-away and/or AID strains background. D, Pearson correlations for all pairwise comparisons of genome-wide nucleosome occupancy change over 100 bp windows for the indicated
CR depletion strains in the absence of depletion (mock-treated). E, Screenshots of sample regions in which nucleosomes become stabilized (marked with a red rectangle) upon depletion of RSC
(top) or SWI-SNF (bottom). F, Average plots of nucleosome occupancy for all nucleosomes becoming stabilized upon depletion of RSC (top) or SWI-SNF (bottom). G, Screenshots of sample regions
in which nucleosomes become destabilized (marked with a red rectangle) upon depletion of ISW2 (top) or INO80 (bottom). H, Average plots of nucleosome occupancy for all nucleosomes
destabilized upon depletion of ISW2 (top) or INO80 (bottom). I,J, Average plots of nucleosome occupancy with (red) or without (blue) depletion of ISW1 (i) or CHD1 (j), plotted separately for
genes with the lowest (top) or highest (bottom) binding by the relevant CR (average binding profiles shown in green). SUPPLEMENTARY FIGURE 3 REMODELER REDUNDANCY IN NUCLEOSOME POSITIONING.
A, Nucleosome occupancy change upon depletion of RSC (left) or SWI-SNF (right) at sites bound by each remodeler and displaying varying binding signal (+, +/-, -) of the other one. B,
Nucleosome occupancy change upon depletion of ISW2 at sites bound by this remodeler and displaying varying binding signal of INO80 (as in (a)). C, Nucleosome occupancy change upon depletion
of INO80 at sites bound by this remodeler and displaying varying binding signal of ISW2 (as in (a)). D, Boxplot of INO80 binding signal at INO80-bound sites displaying varying binding signal
of ISW2. In (A-D), asterisks indicate significant differences (p<0.05, Mann-Whitney test). E, Snapshot of a sample genomic region displaying nucleosome occupancy change upon depletion of
ISW1, CHD1 or both remodelers simultaneously. SUPPLEMENTARY FIGURE 4 MULTIPLE CONCORDANT AND OPPOSING REMODELER ACTIVITIES CONTROL PROMOTER NUCLEOSOME OCCUPANCY. A, Nucleosome occupancy
change upon RSC depletion at sites bound by RSC (left) and change upon SWI-SNF depletion at sites bound by SWI-SNF (right). In both cases comparisons are made between sites co-bound by ISW2
(+) or not (-). Asterisk indicates significant difference (p<0.05, Mann-Whitney test). B, Nucleosome occupancy change upon INO80 depletion at sites bound by INO80 and co-bound by RSC
and/or SWI-SNF or not, as indicated below. C, Average plots of nucleosome occupancy for all nucleosomes destabilized upon depletion of ISW2, comparing wild-type cells and cells depleted of
RSC, ISW2, or both remodelers simultaneously. D, Average plots of nucleosome occupancy for all nucleosomes destabilized upon depletion of INO80, comparing for wild-type cells and cells
depleted of RSC, INO80, or both remodelers simultaneously. E, Average plots of nucleosome occupancy for all nucleosomes stabilized upon depletion of RSC, comparing wild-type cells and cells
depleted of RSC, RSC and ISW2, RSC and INO80, or all three remodelers simultaneously. SUPPLEMENTARY FIGURE 5 LINKS BETWEEN NUCLEOSOME OCCUPANCY AND TRANSCRIPTIONAL REGULATION. A, Scatterplot
showing relationship between TBP binding change at gene promoters and RNAPII binding change in corresponding gene bodies following SWI-SNF depletion, for all genes with a well-defined TSS;
Pearson R value shown. B, Scatterplot showing relationship between TBP binding and nucleosome occupancy changes following SWI-SNF depletion at down-regulated genes. C, Average plots
displaying nucleosome occupancy, RNAPII and TBP ChIP signals, in the presence and absence of SWI-SNF, for those genes down-regulated upon SWI-SNF depletion. D-F, Average plots displaying
nucleosome occupancy together with RNAPII and TBP ChIP-seq signals for genes up-regulated by INO80 depletion (D), down-regulated by INO80 depletion (E), or up-regulated by ISW2 depletion
(F), in each case with or without (+/-) the indicated CR. G, Scatter plot displaying the relationship between TBP binding and nucleosome occupancy changes following double “puller” depletion
at genes where transcription was affected (see Fig. 5b, c). H, Scatterplot displaying the TBP - RNAPII ChIP-seq signal relationship at all genes following double “puller” depletion. I-K,
Average plots displaying nucleosome occupancy together with RNAPII and TBP ChIP-seq signals for genes down-regulated upon ISW1 depletion (I), up-regulated upon ISW1 depletion (J), or
up-regulated upon CHD1 depletion (K), in each case with or without (+/-) the indicated CR. SUPPLEMENTARY FIGURE 6 EFFECTS OF REMODELER DEPLETION ON TSS SELECTION. A, Snapshot of genomic
region showing 5’-RACE signal for the Watson (w) and the Crick (c) strands as well as nucleosome occupancy in the presence and absence of RSC; upon RSC depletion the _RPO49_ gene
transcription initiates more upstream comparing to wild-type conditions. B, As in (A) but for SWI-SNF depletion; upon SWI-SNF depletion the _SNQ2_ gene transcription initiates more
frequently downstream comparing to wild-type conditions; additionally, there is more initiation events in the opposite strand just downstream from the upstream-most _SNQ2_ TSS. C, As in (A)
but for ISW2 and INO80 simultaneous depletion; upon “pullers” depletion the _FRP6_ gene transcription initiates at a downstream position comparing to wild-type conditions. D, Average 5’-RACE
signal at genes upregulated upon simultaneous depletion of ISW2 and INO80. E, As in (D) but for downregulated genes. SUPPLEMENTARY FIGURE 7 EFFECT OF “PULLER” REMODELERS ON NUCLEOSOME
OCCUPANCY. Heatmaps showing nucleosome occupancy, centered at TSS positions determined in cells depleted of ISW2 and INO80, shown in wild type cells and cells depleted of both remodelers.
SUPPLEMENTARY INFORMATION SUPPLEMENTARY INFORMATION Supplementary Figures 1–7 REPORTING SUMMARY SUPPLEMENTARY TABLE 1 _K_-means clustering of ChEC-seq data SUPPLEMENTARY TABLE 2 GO of
clusters SUPPLEMENTARY TABLE 3 Strain list SOURCE DATA SOURCE DATA FIG. 2C SOURCE DATA FIG. 2D RIGHTS AND PERMISSIONS Reprints and permissions ABOUT THIS ARTICLE CITE THIS ARTICLE Kubik, S.,
Bruzzone, M.J., Challal, D. _et al._ Opposing chromatin remodelers control transcription initiation frequency and start site selection. _Nat Struct Mol Biol_ 26, 744–754 (2019).
https://doi.org/10.1038/s41594-019-0273-3 Download citation * Received: 01 February 2019 * Accepted: 28 June 2019 * Published: 05 August 2019 * Issue Date: August 2019 * DOI:
https://doi.org/10.1038/s41594-019-0273-3 SHARE THIS ARTICLE Anyone you share the following link with will be able to read this content: Get shareable link Sorry, a shareable link is not
currently available for this article. Copy to clipboard Provided by the Springer Nature SharedIt content-sharing initiative