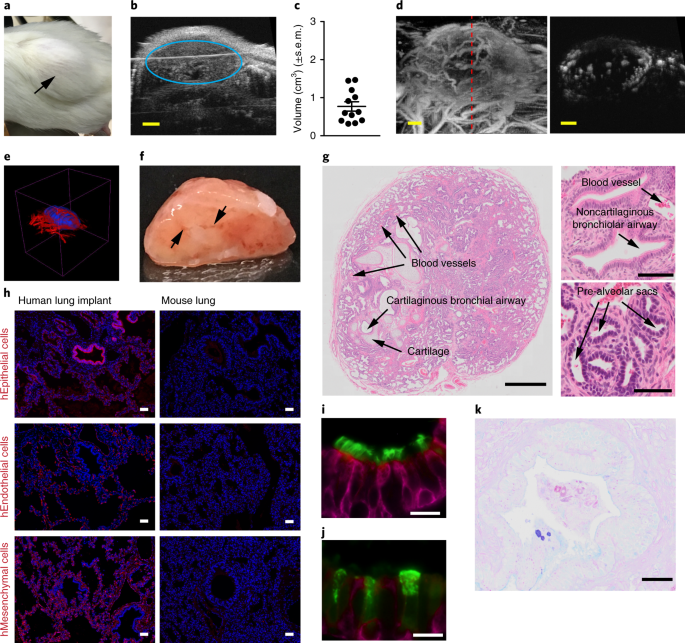
- Select a language for the TTS:
- UK English Female
- UK English Male
- US English Female
- US English Male
- Australian Female
- Australian Male
- Language selected: (auto detect) - EN
Play all audios:
ABSTRACT A major limitation of current humanized mouse models is that they primarily enable the analysis of human-specific pathogens that infect hematopoietic cells. However, most human
pathogens target other cell types, including epithelial, endothelial and mesenchymal cells. Here, we show that implantation of human lung tissue, which contains up to 40 cell types,
including nonhematopoietic cells, into immunodeficient mice (lung-only mice) resulted in the development of a highly vascularized lung implant. We demonstrate that emerging and clinically
relevant human pathogens such as Middle East respiratory syndrome coronavirus, Zika virus, respiratory syncytial virus and cytomegalovirus replicate in vivo in these lung implants. When
incorporated into bone marrow/liver/thymus humanized mice, lung implants are repopulated with autologous human hematopoietic cells. We show robust antigen-specific humoral and T-cell
responses following cytomegalovirus infection that control virus replication. Lung-only mice and bone marrow/liver/thymus-lung humanized mice substantially increase the number of human
pathogens that can be studied in vivo, facilitating the in vivo testing of therapeutics. Access through your institution Buy or subscribe This is a preview of subscription content, access
via your institution ACCESS OPTIONS Access through your institution Access Nature and 54 other Nature Portfolio journals Get Nature+, our best-value online-access subscription $29.99 / 30
days cancel any time Learn more Subscribe to this journal Receive 12 print issues and online access $209.00 per year only $17.42 per issue Learn more Buy this article * Purchase on
SpringerLink * Instant access to full article PDF Buy now Prices may be subject to local taxes which are calculated during checkout ADDITIONAL ACCESS OPTIONS: * Log in * Learn about
institutional subscriptions * Read our FAQs * Contact customer support SIMILAR CONTENT BEING VIEWED BY OTHERS METHODOLOGY OF MURINE LUNG CANCER MIMICS CLINICAL LUNG ADENOCARCINOMA
PROGRESSION AND METASTASIS Article Open access 28 February 2025 A ROBUST MOUSE MODEL OF HPIV-3 INFECTION AND EFFICACY OF GS-441524 AGAINST VIRUS-INDUCED LUNG PATHOLOGY Article Open access 05
September 2024 COVID-19 AND GRAFT-VERSUS-HOST DISEASE: A TALE OF TWO DISEASES (AND WHY AGE MATTERS) Article 09 December 2020 DATA AVAILABILITY The data generated are available from
corresponding authors on reasonable request. REFERENCES * Cockrell, A. S. et al. A mouse model for MERS coronavirus-induced acute respiratory distress syndrome. _Nat. Microbiol._ 2, 16226
(2016). Article CAS Google Scholar * Morrison, T. E. & Diamond, M. S. Animal models of Zika virus infection, pathogenesis, and immunity. _J. Virol._ 91, e00009–17 (2017). CAS PubMed
PubMed Central Google Scholar * Safronetz, D., Geisbert, T. W. & Feldmann, H. Animal models for highly pathogenic emerging viruses. _Curr. Opin. Virol._ 3, 205–209 (2013). Article
Google Scholar * Schmitt, K. et al. Zika viral infection and neutralizing human antibody response in a BLT humanized mouse model. _Virology_ 515, 235–242 (2018). Article CAS Google
Scholar * Crawford, L. B., Streblow, D. N., Hakki, M., Nelson, J. A. & Caposio, P. Humanized mouse models of human cytomegalovirus infection. _Curr. Opin. Virol._ 13, 86–92 (2015).
Article CAS Google Scholar * Taylor, G. Animal models of respiratory syncytial virus infection. _Vaccine_ 35, 469–480 (2017). Article CAS Google Scholar * Gupta, U. D. & Katoch, V.
M. Animal models of tuberculosis. _Tuberculosis (Edinb.)_ 85, 277–293 (2005). Article CAS Google Scholar * Fonseca, K. L., Rodrigues, P. N. S., Olsson, I. A. S. & Saraiva, M.
Experimental study of tuberculosis: from animal models to complex cell systems and organoids. _PLoS Pathog._ 13, e1006421 (2017). Article Google Scholar * Pickles, R. J. & DeVincenzo,
J. P. Respiratory syncytial virus (RSV) and its propensity for causing bronchiolitis. _J. Pathol._ 235, 266–276 (2015). Article Google Scholar * Perlman, R. L. Mouse models of human
disease: an evolutionary perspective. _Evol. Med. Public Health_ 2016, 170–176 (2016). PubMed PubMed Central Google Scholar * Shultz, L. D., Brehm, M. A., Garcia-Martinez, J. V. &
Greiner, D. L. Humanized mice for immune system investigation: progress, promise and challenges. _Nat. Rev. Immunol._ 12, 786–798 (2012). Article CAS Google Scholar * Garcia, J. V.
Humanized mice for HIV and AIDS research. _Curr. Opin. Virol._ 19, 56–64 (2016). Article Google Scholar * Wahl, A. et al. A cluster of virus-encoded microRNAs accelerates acute systemic
Epstein–Barr virus infection but does not significantly enhance virus-induced oncogenesis in vivo. _J. Virol._ 87, 5437–5446 (2013). Article CAS Google Scholar * Melkus, M. W. et al.
Humanized mice mount specific adaptive and innate immune responses to EBV and TSST-1. _Nat. Med._ 12, 1316–1322 (2006). Article CAS Google Scholar * Islas-Ohlmayer, M. et al. Experimental
infection of NOD/SCID mice reconstituted with human CD34+ cells with Epstein–Barr virus. _J. Virol._ 78, 13891–13900 (2004). Article CAS Google Scholar * Bente, D. A., Melkus, M. W.,
Garcia, J. V. & Rico-Hesse, R. Dengue fever in humanized NOD/SCID mice. _J. Virol._ 79, 13797–13799 (2005). Article CAS Google Scholar * Smith, M. S. et al. Granulocyte-colony
stimulating factor reactivates human cytomegalovirus in a latently infected humanized mouse model. _Cell Host Microbe_ 8, 284–291 (2010). Article CAS Google Scholar * Crawford, L. B. et
al. Human cytomegalovirus induces cellular and humoral virus-specific immune responses in humanized BLT mice. _Sci. Rep._ 7, 937 (2017). Article Google Scholar * Wang, L. X. et al.
Humanized-BLT mouse model of Kaposi’s sarcoma-associated herpesvirus infection. _Proc. Natl Acad. Sci. USA_ 111, 3146–3151 (2014). Article CAS Google Scholar * Cockrell, A. S. et al.
Mouse dipeptidyl peptidase 4 is not a functional receptor for Middle East respiratory syndrome coronavirus infection. _J. Virol._ 88, 5195–5199 (2014). Article Google Scholar * Coleman, C.
M., Matthews, K. L., Goicochea, L. & Frieman, M. B. Wild-type and innate immune-deficient mice are not susceptible to the Middle East respiratory syndrome coronavirus. _J. Gen. Virol._
95, 408–412 (2014). Article CAS Google Scholar * Martinez-Torres, F., Nochi, T., Wahl, A., Garcia, J. V. & Denton, P. W. Hypogammaglobulinemia in BLT humanized mice—an animal model of
primary antibody deficiency. _PloS ONE_ 9, e108663 (2014). Article Google Scholar * Nochi, T., Denton, P. W., Wahl, A. & Garcia, J. V. Cryptopatches are essential for the development
of human GALT. _Cell Rep._ 3, 1874–1884 (2013). Article CAS Google Scholar * Dudek, T. E. et al. Rapid evolution of HIV-1 to functional CD8(+) T cell responses in humanized BLT mice.
_Sci. Transl. Med._ 4, 143ra198 (2012). Article Google Scholar * Brainard, D. M. et al. Induction of robust cellular and humoral virus-specific adaptive immune responses in human
immunodeficiency virus-infected humanized BLT mice. _J. Virol._ 83, 7305–7321 (2009). Article CAS Google Scholar * Zhou, J., Chu, H., Chan, J. F. & Yuen, K. Y. Middle East respiratory
syndrome coronavirus infection: virus-host cell interactions and implications on pathogenesis. _Virol. J._ 12, 218 (2015). Article Google Scholar * Frumence, E. et al. The South Pacific
epidemic strain of Zika virus replicates efficiently in human epithelial A549 cells leading to IFN-_β_ production and apoptosis induction. _Virology_ 493, 217–226 (2016). Article CAS
Google Scholar * Liu, S., DeLalio, L. J., Isakson, B. E. & Wang, T. T. AXL-mediated productive infection of human endothelial cells by Zika virus. _Circ. Res._ 119, 1183–1189 (2016).
Article CAS Google Scholar * Hamel, R. et al. Biology of Zika virus infection in human skin cells. _J. Virol._ 89, 8880–8896 (2015). Article CAS Google Scholar * Franks, T. J. et al.
Resident cellular components of the human lung: current knowledge and goals for research on cell phenotyping and function. _Proc. Am. Thorac. Soc._ 5, 763–766 (2008). Article Google Scholar
* Sousa, A. Q. et al. Postmortem findings for 7 neonates with congenital Zika virus infection. _Emerg. Infect. Dis._ 23, 1164–1167 (2017). Article Google Scholar * Cunha, B. A.
Cytomegalovirus pneumonia: community-acquired pneumonia in immunocompetent hosts. _Infect. Dis. Clin. North Am._ 24, 147–158 (2010). Article Google Scholar * Gordon, C. L. et al. Tissue
reservoirs of antiviral T cell immunity in persistent human CMV infection. _J. Exp. Med._ 214, 651–667 (2017). CAS PubMed PubMed Central Google Scholar * Lyon, S. M. & Rossman, M. D.
Pulmonary tuberculosis. _Microbiol. Spectr._ 5, 1–13 (2017). Article Google Scholar * Gessner, R. et al. High-resolution, high-contrast ultrasound imaging using a prototype dual-frequency
transducer: in vitro and in vivo studies. _IEEE Trans. Ultrason. Ferroelectr. Freq. Control_ 57, 1772–1781 (2010). Article Google Scholar * Gessner, R. C., Frederick, C. B., Foster, F. S.
& Dayton, P. A. Acoustic angiography: a new imaging modality for assessing microvasculature architecture. _International J. Biomed. Imaging_ 2013, 936593 (2013). Article Google Scholar
* Davis, L. S. et al. Inflammation, immune reactivity, and angiogenesis in a severe combined immunodeficiency model of rheumatoid arthritis. _Am. J. Pathol._ 160, 357–367 (2002). Article
Google Scholar * Huang, S. Y., Tien, H. F., Su, F. H. & Hsu, S. M. Nonirradiated NOD/SCID-human chimeric animal model for primary human multiple myeloma: a potential in vivo culture
system. _Am. J. Pathol._ 164, 747–756 (2004). Article Google Scholar * Hou, W. et al. Determination of the cell permissiveness spectrum, mode of RNA replication, and RNA-protein
interaction of Zika virus. _BMC Infect. Dis._ 17, 239 (2017). Article Google Scholar * Liesman, R. M. et al. RSV-encoded NS2 promotes epithelial cell shedding and distal airway
obstruction. _J. Clin. Invest._ 124, 2219–2233 (2014). Article CAS Google Scholar * Aherne, W., Bird, T., Court, S. D., Gardner, P. S. & McQuillin, J. Pathological changes in virus
infections of the lower respiratory tract in children. _J. Clin. Pathol._ 23, 7–18 (1970). Article CAS Google Scholar * Arend, K. C., Ziehr, B., Vincent, H. A. & Moorman, N. J.
Multiple transcripts encode full-length human cytomegalovirus IE1 and IE2 proteins during lytic infection. _J. Virol._ 90, 8855–8865 (2016). Article CAS Google Scholar * Azevedo, L. S. et
al. Cytomegalovirus infection in transplant recipients. _Clinics (Sao Paulo)_ 70, 515–523 (2015). Article Google Scholar * Sathaliyawala, T. et al. Distribution and compartmentalization
of human circulating and tissue-resident memory T cell subsets. _Immunity_ 38, 187–197 (2013). Article CAS Google Scholar * Pitt, E. A. et al. The D-form of a novel heparan binding
peptide decreases cytomegalovirus infection in vivo and in vitro. _Antiviral Res._ 135, 15–23 (2016). Article CAS Google Scholar * Sinzger, C. et al. Cloning and sequencing of a highly
productive, endotheliotropic virus strain derived from human cytomegalovirus TB40/E. _J. Gen. Virol._ 89, 359–368 (2008). Article CAS Google Scholar * Ostermann, E., Spohn, M.,
Indenbirken, D. & Brune, W. Complete genome sequence of a human cytomegalovirus strain AD169 bacterial artificial chromosome clone. _Genome Announc._ 4, 1–2 (2016). Article Google
Scholar * Wang, D. & Shenk, T. Human cytomegalovirus UL131 open reading frame is required for epithelial cell tropism. _J. Virol._ 79, 10330–10338 (2005). Article CAS Google Scholar
* Munro, S. C. et al. Diagnosis of and screening for cytomegalovirus infection in pregnant women. _J. Clin. Microbiol._ 43, 4713–4718 (2005). Article CAS Google Scholar * Gamadia, L. E.,
Rentenaar, R. J., van Lier, R. A. & ten Berge, I. J. Properties of CD4(+) T cells in human cytomegalovirus infection. _Hum. Immunol._ 65, 486–492 (2004). Article CAS Google Scholar *
Vaz-Santiago, J. et al. Ex vivo stimulation and expansion of both CD4(+) and CD8(+) T cells from peripheral blood mononuclear cells of human cytomegalovirus-seropositive blood donors by
using a soluble recombinant chimeric protein, IE1-pp65. _J. Virol._ 75, 7840–7847 (2001). Article CAS Google Scholar * Sinclair, E. et al. CMV antigen-specific CD4+ and CD8+ T cell IFNγ
expression and proliferation responses in healthy CMV-seropositive individuals. _Viral Immunol._ 17, 445–454 (2004). Article CAS Google Scholar * Gillespie, G. M. et al. Functional
heterogeneity and high frequencies of cytomegalovirus-specific CD8(+) T lymphocytes in healthy seropositive donors. _J. Virol._ 74, 8140–8150 (2000). Article CAS Google Scholar * Nastke,
M. D. et al. Major contribution of codominant CD8 and CD4 T cell epitopes to the human cytomegalovirus-specific T cell repertoire. _Cell. Mol. Life Sci._ 62, 77–86 (2005). Article CAS
Google Scholar * Kessing, C. F. et al. In vivo suppression of HIV rebound by didehydro-cortistatin a, a “block-and-lock” strategy for HIV-1 treatment. _Cell Rep._ 21, 600–611 (2017).
Article CAS Google Scholar * Kovarova, M. et al. A long-acting formulation of the integrase inhibitor raltegravir protects humanized BLT mice from repeated high-dose vaginal HIV
challenges. _J. Antimicrob. Chemother._ 71, 1586–1596 (2016). Article CAS Google Scholar * Tsai, P. et al. CD19xCD3 DART protein mediates human B-cell depletion in vivo in humanized BLT
mice. _Mol. Ther. Oncolytics_ 3, 15024 (2016). Article CAS Google Scholar * Lilleri, D., Zelini, P., Fornara, C., Comolli, G. & Gerna, G. Inconsistent responses of
cytomegalovirus-specific T cells to pp65 and IE-1 versus infected dendritic cells in organ transplant recipients. _Am. J. Transplant._ 7, 1997–2005 (2007). Article CAS Google Scholar *
Jackson, S. E., Mason, G. M., Okecha, G., Sissons, J. G. & Wills, M. R. Diverse specificities, phenotypes, and antiviral activities of cytomegalovirus-specific CD8+ T cells. _J. Virol._
88, 10894–10908 (2014). Article CAS Google Scholar * Braendstrup, P. et al. Identification and HLA-tetramer-validation of human CD4+ and CD8+ T cell responses against HCMV proteins IE1
and IE2. _PloS ONE_ 9, e94892 (2014). Article Google Scholar * Khan, N., Cobbold, M., Keenan, R. & Moss, P. A. Comparative analysis of CD8+ T cell responses against human
cytomegalovirus proteins pp65 and immediate early 1 shows similarities in precursor frequency, oligoclonality, and phenotype. _J. Infect. Dis._ 185, 1025–1034 (2002). Article CAS Google
Scholar * Sacre, K. et al. Expansion of human cytomegalovirus (HCMV) immediate-early 1-specific CD8+ T cells and control of HCMV replication after allogeneic stem cell transplantation. _J.
Virol._ 82, 10143–10152 (2008). Article CAS Google Scholar * Honeycutt, J. B. et al. Macrophages sustain HIV replication in vivo independently of T cells. _J. Clin. Invest._ 126,
1353–1366 (2016). Article Google Scholar * Olesen, R. et al. ART influences HIV persistence in the female reproductive tract and cervicovaginal secretions. _J. Clin. Invest._ 126, 892–904
(2016). Article Google Scholar * Shelton, S. E. et al. Quantification of microvascular tortuosity during tumor evolution using acoustic angiography. _Ultrasound Med. Biol._ 41, 1896–1904
(2015). Article Google Scholar * Honeycutt, J. B. et al. HIV persistence in tissue macrophages of humanized myeloid-only mice during antiretroviral therapy. _Nat. Med._ 23, 638–643 (2017).
Article CAS Google Scholar * Fulcher, M. L., Gabriel, S., Burns, K. A., Yankaskas, J. R. & Randell, S. H. Well-differentiated human airway epithelial cell cultures. _Methods Mol.
Med._ 107, 183–206 (2005). CAS PubMed Google Scholar * Zhang, S. et al. Neutralization mechanism of a highly potent antibody against Zika virus. _Nat. Commun._ 7, 13679 (2016). Article
CAS Google Scholar * Lanciotti, R. S. et al. Genetic and serologic properties of Zika virus associated with an epidemic, Yap State, Micronesia, 2007. _Emerg. Infect. Dis._ 14, 1232–1239
(2008). Article CAS Google Scholar * Hallak, L. K., Spillmann, D., Collins, P. L. & Peeples, M. E. Glycosaminoglycan sulfation requirements for respiratory syncytial virus infection.
_J. Virol._ 74, 10508–10513 (2000). Article CAS Google Scholar * O’Connor, C. M. & Shenk, T. Human cytomegalovirus pUS27 G protein-coupled receptor homologue is required for efficient
spread by the extracellular route but not for direct cell-to-cell spread. _J. Virol._ 85, 3700–3707 (2011). Article Google Scholar * Wang, D., Bresnahan, W. & Shenk, T. Human
cytomegalovirus encodes a highly specific RANTES decoy receptor. _Proc. Natl Acad. Sci. USA_ 101, 16642–16647 (2004). Article CAS Google Scholar * Ziehr, B., Lenarcic, E., Cecil, C. &
Moorman, N. J. The eIF4AIII RNA helicase is a critical determinant of human cytomegalovirus replication. _Virology_ 489, 194–201 (2016). Article CAS Google Scholar * Goonetilleke, N. et
al. Induction of multifunctional human immunodeficiency virus type 1 (HIV-1)-specific T cells capable of proliferation in healthy subjects by using a prime-boost regimen of DNA- and modified
vaccinia virus Ankara-vectored vaccines expressing HIV-1 Gag coupled to CD8+ T-cell epitopes. _J. Virol._ 80, 4717–4728 (2006). Article CAS Google Scholar Download references
ACKNOWLEDGEMENTS This work was supported by NIH grants no. AI103311 (N.J.M.), no. AI123811 (N.J.M.), no. AI110700 (R.S.B.), no. AI100625 (R.S.B.), no. P30 AI027763 (N.G.), no. AI113736
(R.J.P.), no. T32 HL069768 (I.G.N.), no. AI123010 (A.W.), no. AI111899 (J.V.G.), no. AI140799 (J.V.G.), no. MH108179 (J.V.G.), no. CA189479 (P.A.D.) and no. CA170665 (P.A.D) and the North
Carolina University Cancer Research Fund (N.J.M.). This work was also supported by the UNC Center for AIDS Research (CFAR) (grant no. P30 AI050410). We thank K. Arend for the generation of
HCMV virus stocks used in these experiments. We thank P. Collins and M. Peeples for recombinant RSV expressing GFP and C. O’Connor for recombinant HCMV TB40/E expressing luciferase. We thank
G. Clutton for input on the analysis of antigen-specific T-cell responses. The authors thank members of the Garcia laboratory for technical assistance. We thank technicians at the UNC
Animal Histopathology Core, The Marsico Lung Institute Tissue and Procurement Core, and the Department of Comparative Medicine. We also thank J. Schmitz and technicians at the UNC Clinical
Microbiology/Immunology Laboratories. We thank J. Nelson and technicians at the UNC CFAR Virology Core Laboratory and K. Mollan at the UNC CFAR Biostatistics Core. The authors thank M.T.
Heise, L.J. Picker and J.P. Ting for manuscript advice and helpful discussions. AUTHOR INFORMATION Author notes * Christian R. Aguilera-Sandoval Present address: BD Life Sciences, San Jose,
CA, USA * These authors contributed equally: Chandrav De, Maria Abad Fernandez. AUTHORS AND AFFILIATIONS * Division of Infectious Diseases, International Center for the Advancement of
Translational Science, Center for AIDS Research, University of North Carolina, School of Medicine, Chapel Hill, NC, USA Angela Wahl, Chandrav De, Rachel A. Cleary, Claire E. Johnson,
Nathaniel J. Schramm, Christian R. Aguilera-Sandoval & J. Victor Garcia * Department of Microbiology and Immunology, University of North Carolina, Chapel Hill, NC, USA Maria Abad
Fernandez, Erik M. Lenarcic, Yinyan Xu, Laura M. Rank, Heather A. Vincent, Wes Sanders, Allison Boone, Ralph S. Baric, Raymond J. Pickles, Miriam Braunstein, Nathaniel J. Moorman & Nilu
Goonetilleke * Lineberger Comprehensive Cancer Center, University of North Carolina, Chapel Hill, NC, USA Erik M. Lenarcic, Heather A. Vincent, Wes Sanders & Nathaniel J. Moorman *
Department of Epidemiology, University of North Carolina, Chapel Hill, NC, USA Adam S. Cockrell & Ralph S. Baric * Joint Department of Biomedical Engineering, University of North
Carolina and North Carolina State University, Chapel Hill, NC, USA Isabel G. Newsome & Paul A. Dayton * Marsico Lung Institute, University of North Carolina, Chapel Hill, NC, USA Allison
Boone & Raymond J. Pickles * Department of Microbiology and Immunology, University of Oklahoma Health Sciences Center, Oklahoma City, OK, USA William H. Hildebrand * UNC HIV Cure
Center, University of North Carolina, Chapel Hill, NC, USA Nilu Goonetilleke Authors * Angela Wahl View author publications You can also search for this author inPubMed Google Scholar *
Chandrav De View author publications You can also search for this author inPubMed Google Scholar * Maria Abad Fernandez View author publications You can also search for this author inPubMed
Google Scholar * Erik M. Lenarcic View author publications You can also search for this author inPubMed Google Scholar * Yinyan Xu View author publications You can also search for this
author inPubMed Google Scholar * Adam S. Cockrell View author publications You can also search for this author inPubMed Google Scholar * Rachel A. Cleary View author publications You can
also search for this author inPubMed Google Scholar * Claire E. Johnson View author publications You can also search for this author inPubMed Google Scholar * Nathaniel J. Schramm View
author publications You can also search for this author inPubMed Google Scholar * Laura M. Rank View author publications You can also search for this author inPubMed Google Scholar * Isabel
G. Newsome View author publications You can also search for this author inPubMed Google Scholar * Heather A. Vincent View author publications You can also search for this author inPubMed
Google Scholar * Wes Sanders View author publications You can also search for this author inPubMed Google Scholar * Christian R. Aguilera-Sandoval View author publications You can also
search for this author inPubMed Google Scholar * Allison Boone View author publications You can also search for this author inPubMed Google Scholar * William H. Hildebrand View author
publications You can also search for this author inPubMed Google Scholar * Paul A. Dayton View author publications You can also search for this author inPubMed Google Scholar * Ralph S.
Baric View author publications You can also search for this author inPubMed Google Scholar * Raymond J. Pickles View author publications You can also search for this author inPubMed Google
Scholar * Miriam Braunstein View author publications You can also search for this author inPubMed Google Scholar * Nathaniel J. Moorman View author publications You can also search for this
author inPubMed Google Scholar * Nilu Goonetilleke View author publications You can also search for this author inPubMed Google Scholar * J. Victor Garcia View author publications You can
also search for this author inPubMed Google Scholar CONTRIBUTIONS A.W. inoculated mice with HCMV; collected and processed PB and tissues from HCMV-exposed mice; performed experiments with PB
and tissue cells and analyzed the data; performed the flow cytometric and IHC analysis of naïve LoM and BLT-L mice; performed the human cytokine/chemokine analysis; inoculated mice with BCG
and collected tissues from BCG-exposed mice; conceived and designed experiments; contributed to data interpretation, data presentation and manuscript writing; and conceived, designed and
coordinated the study, the preparation of the manuscript and its revision. C.D. inoculated mice with HCMV; performed the GCV experiment, the in vivo imaging of mice and neutralization assay;
collected and processed PB and tissues from HCMV-exposed mice; performed experiments with PB and tissue cells and analyzed the data; performed the human cytokine/chemokine analysis;
inoculated mice with RSV; and collected and processed tissues and measured RSV-GFP expression by flow cytometry. M.A.F. performed experiments with PB and tissue cells and analyzed the data;
standardized, designed, performed and analyzed immunological assays; and contributed to data interpretation, data presentation and manuscript writing. E.M.L. performed experiments with PB
and tissue cells and analyzed the data and performed the HCMV real-time PCR analyses. Y.X. performed experiments with PB and tissue cells and analyzed the data and standardized, designed,
performed and analyzed immunological assays. A.S.C. produced stocks of MERS-CoV; inoculated mice with MERS-CoV and collected tissues from MERS-CoV infected animals; conceived and designed
experiments; and contributed to data interpretation, data presentation and manuscript writing. R.A.C. performed dual immunofluorescence staining of infected LoM. C.E.J. performed
immunofluorescence staining of animals, collected tissues from BCG-exposed mice and performed the acid-fast staining. N.J.S. produced stocks of ZIKV, inoculated mice with ZIKV, and collected
and processed tissue from ZIKV-infected mice. L.M.R. processed and cultured tissue homogenate to determine the number of the BCG c.f.u. and the analyzed data. I.G.N. performed the
ultrasound and acoustic angiography imaging and analysis. H.A.V. and W.S. performed experiments with PB and tissue cells and analyzed the data and performed the HCMV transcriptome analysis.
C.R.A.S. collected and processed PB and tissues from HCMV-exposed mice. A.B. performed immunofluorescence staining of animals. W.H.H. helped conceive and design experiments. P.A.D., R.S.B.,
N.J.M. and N.G. conceived and designed experiments and contributed to data interpretation, data presentation and manuscript writing. R.J.P. provided stocks of RSV-GFP; performed the
immunofluorescent analysis of tissues sections for RSV infection and AB-PAS staining, and the analysis of human lung implant structures; conceived and designed experiments; and contributed
to data interpretation, data presentation and manuscript writing. M.B. processed and cultured tissue homogenate to determine the number of the BCG c.f.u. and the analyzed data; conceived and
designed experiments; and contributed to data interpretation, data presentation and manuscript writing. J.V.G. conceived and designed experiments and contributed to data interpretation,
data presentation and manuscript writing; and conceived, designed and coordinated the study, the preparation of the manuscript and its revision. CORRESPONDING AUTHORS Correspondence to
Angela Wahl or J. Victor Garcia. ETHICS DECLARATIONS COMPETING INTERESTS P.A.D. is an inventor of the acoustic angiography imaging technique, and a cofounder of SonoVol, Inc., a company that
has licensed this patent. ADDITIONAL INFORMATION PUBLISHER’S NOTE: Springer Nature remains neutral with regard to jurisdictional claims in published maps and institutional affiliations.
INTEGRATED SUPPLEMENTARY INFORMATION SUPPLEMENTARY FIGURE 1 HISTOLOGICAL ANALYSIS OF THE STRUCTURE AND CELLULAR COMPOSITION OF DONOR MATCHED HUMAN LUNG TISSUE PRE- AND POST-IMPLANTATION. The
structure and cellular composition of human lung tissue pre-implantation (n=2 analyzed) and donor matched LoM lung implant harvested 2 months post-implantation (n=4 analyzed) were analyzed
by (a) H&E and (b) immunofluorescent staining. In b, co-staining was performed for epithelial cells (cytokeratin 19, magenta) and cilia (alpha-acetylated tubulin, green) or club cells
(CC10, green). Arrows show cuboidal cells lining alveoli. In a, scale bars are 100 µm (left panel) and 200 µm (right panel). In b, scale bars are 200 µm (left panels) and 100 µm (right
panels). SUPPLEMENTARY FIGURE 2 PRESENCE OF MOUSE CELLS IN THE HUMAN LUNG IMPLANTS OF LOM AND NSG MOUSE LUNG. Immunohistochemical staining for murine epithelial cells, endothelial cells,
hematopoietic cells in LoM human lung implants (n=3 analyzed, top panels) and the mouse lung (n=1 analyzed, bottom panels). Images: 10X, scale bars: 100 µm, positive cells: brown. m, mouse.
SUPPLEMENTARY FIGURE 3 PRESENCE OF HUMAN IMMUNE CELLS IN HUMAN LUNG TISSUE PRE- AND POST-IMPLANTATION. Immunohistochemical staining for human hematopoietic (hCD45) cells including
macrophages (hCD68), dendritic cells (hCD11c), B cells (hCD20) and T cells (hCD3) in human donor matched lung tissue pre-implantation (n=1 analyzed, left panels) and two months
post-implantation (n=1 analyzed, right panels). Images: 10X, scale bars: 100 µm, positive cells: brown. SUPPLEMENTARY FIGURE 4 IN VIVO GENE EXPRESSION PROFILE OF HCMV-INFECTED LOM IS
CONSISTENT WITH LYTIC REPLICATION. Total RNA was extracted from human lung implants harvested from HCMV TB40/E infected LoM 14 days post exposure (n=2 TB40/E infected implants). Double
stranded cDNA ((ds)cDNA) was generated from ribosomal RNA (rRNA) depleted total RNA. HCMV (ds)cDNA was enriched with custom designed biotinylated probes spanning both strands of the entire
HCMV genome and sequenced using next generation sequencing. High quality reads were aligned to the HCMV genome, and viral expression was quantified in read per kilobase per million (rpkm).
Values show read counts per gene normalized to gene length read (rpkm). SUPPLEMENTARY FIGURE 5 RECONSTITUTION OF THE PERIPHERAL BLOOD OF BLT-L MICE WITH HUMAN INNATE AND ADAPTIVE IMMUNE
CELLS. Levels of (a) human hematopoietic cells (hCD45) including (b) human myeloid cells (hCD33), B cells (hCD19) and T cells (hCD3) as well as the (c) levels of CD4+ (hCD4) and CD8+ (hCD8)
T cells and (d) ratio of human CD4:CD8 T cells in the peripheral blood of BLT-L mice (n=11, filled circles). Horizontal lines represent mean ± s.e.m. SUPPLEMENTARY FIGURE 6 LEVELS OF HUMAN
IMMUNE CELLS IN THE HUMAN LUNG IMPLANTS AND MOUSE LUNG OF BLT-L MICE. Levels of (a) human hematopoietic cells (hCD45) including (b) human myeloid cells (hCD33), B cells (hCD19) and T cells
(hCD3) in the human lung implants (circles; hCD45, hCD33, and hCD3 n=18, hCD19 n=15) and mouse lung (squares, n=11) of BLT-L mice. (c) Levels of CD4+ (hCD4) and CD8+ (hCD8) T cells and (d)
ratio of human CD4:CD8 T cells in the human lung implants (circles, n=15) and mouse lungs (squares, n=11) of BLT-L mice. (e) Human CD4+ and CD8+ T cell activation (CD38+HLA-DR+) levels in
the human lung implant (circles, n=7) and mouse lung (squares, n=4) of BLT-L mice. Horizontal lines represent mean ± s.e.m. Human immune cell levels in the human lung implants and mouse lung
were compared with a two-tailed Mann-Whitney test. SUPPLEMENTARY FIGURE 7 SYSTEMIC PRESENCE OF HUMAN IMMUNE CELLS IN BLT-L MICE. (a-c) The memory phenotype of human T cells in the human
lung implants of BLT-L mice (n=4 BLT-L mice, one lung implant per animal). (a) Percent of CD4+ (filled circles) and CD8+ (filled squares) human T cells expressing a memory phenotype
(CD45RO+). (b) Percent of memory (CD45RO+) CD4+ (circles) and CD8+ (squares) human T cells expressing an effector memory (Tem, CCR7neg, closed symbols) or central memory (Tcm, CCR7+, open
symbols) phenotype. (c) Percent of memory (CD45RO+) CD4+ (filled circles) and CD8+ (filled squares) T cells that are tissue-resident (TRM, CD69+). (d) Flow cytometry gating scheme. Regions
identify the following human cell populations: RI (live cells), RII (human hematopoietic cells), RIII (T cells), RIV (CD8+ T cells), RV (CD4+ T cells), RVI (memory CD8+ T cells), RVII (CD8+
Tem), RVIII (CD8+ Tcm), RIX (CD8+ TRM), RX (memory CD4+ T cells), RXI (CD4+ Tem), RXII (CD4+ Tcm) and RXIII (CD4+ TRM). In a-c, horizontal lines represent mean ± s.e.m. (e) Human
hematopoietic (hCD45) cells including dendritic cells (hCD11c), macrophages (hCD68), B cells (hCD20) and T cells (hCD3, hCD4 and hCD8) in lymphoid (spleen and lymph nodes) and non-lymphoid
(liver and mouse lung) of BLT-L mice by immunohistochemical staining (positive cells: brown). Images shown are at 20X magnification and represent three BLT-L mice (scale bars: 100 µm).
SUPPLEMENTARY FIGURE 8 INCREASED PLASMA HUMAN CYTOKINE AND CHEMOKINE LEVELS IN BLT-L MICE FOLLOWING HCMV EXPOSURE. Levels of human GM-CSF IFN-γ, IL-6, IL-8, MDC, IP-10, GRO and MCP-1 in the
PB plasma of BLT-L mice (n=10 mice, filled circles) pre and 4 days after HCMV TB40/E inoculation. A value of 3.2 pg/ml was graphed for measurements below the limit of detection of the assay
(3.2 pg/ml, shown with a dashed line). Human cytokine and chemokine levels pre and post HCMV inoculation were compared with a two-tailed Wilcoxon matched-pairs signed rank test.
SUPPLEMENTARY FIGURE 9 IN VIVO REPLICATION OF HCMV AD169 IN LOM HUMAN LUNG IMPLANTS. (a) HCMV-DNA levels in LoM human lung implants at 4, 7, 14, 21 and 28 days post AD169 exposure (day 4:
n=3 implants, days 7, 14, 21 and 28: n=4 implants, filled squares). Horizontal lines represent mean ± s.e.m. (b) HCMV immediate early (IE), early (E) and late (L) proteins in the human lung
implant of an AD169-infected LoM 21 days post-exposure (n=1 lung implant analyzed, positive cells: brown). Images shown are at 40X magnification (scale bars: 50 µm). Positive cells in the
bottom panel are indicated with black arrows. SUPPLEMENTARY FIGURE 10 PLASMA OF HCMV-EXPOSED BLT-L MICE CONTAINS HCMV NEUTRALIZING ACTIVITY. Heat-inactivated plasma from naïve (n=1, open
blue circles) and repeatedly HCMV TB40/E exposed BLT-L mice (n=6, filled symbols) was incubated with HCMV TB40/E expressing RFP for 1 h prior to the addition of virus to epithelial cells
(ARPE-19). Epithelial cells were incubated at 37 °C with the virus/plasma mixture for 2 h at which time the virus/plasma mixture was removed and fresh media added. Shown is the number of
HCMV TB40/E-RFP+ epithelial cells 72 h post-infection in quadruplicate wells. Horizontal lines represent mean ± s.e.m. The percent reduction in TB40/E RFP+ cells compared to wells infected
with HCMV pre-treated with naïve control plasma is shown in the table. SUPPLEMENTARY FIGURE 11 GATING STRATEGIES FOR THE IDENTIFICATION OF HCMV-SPECIFIC HUMAN T CELL RESPONSES IN BLT-L MICE
BY INTRACELLULAR CYTOKINE STAINING (ICS) AND PENTAMER STAINING. Representative flow cytometry plots indicating the gating used to detect (a) human CD8+ T cells expressing IFN-γ and CD107a
and (b) human CD4+ T cells expressing IFN-γ and TNFα by ICS. (c) Representative flow cytometry plots indicating the gating strategy used to detect HLA class1a-restricted HCMV-specific human
CD8+ T cells by pentamer staining. SUPPLEMENTARY INFORMATION SUPPLEMENTARY INFORMATION Supplementary Figs. 1–11 and Supplementary Tables 1–10 REPORTING SUMMARY SUPPLEMENTARY VIDEO 1:
VASCULARIZATION OF HUMAN LUNG IMPLANTS Three-dimensional rendering of vascularization (red) of a human lung implant (blue). RIGHTS AND PERMISSIONS Reprints and permissions ABOUT THIS ARTICLE
CITE THIS ARTICLE Wahl, A., De, C., Abad Fernandez, M. _et al._ Precision mouse models with expanded tropism for human pathogens. _Nat Biotechnol_ 37, 1163–1173 (2019).
https://doi.org/10.1038/s41587-019-0225-9 Download citation * Received: 04 December 2018 * Accepted: 12 July 2019 * Published: 26 August 2019 * Issue Date: October 2019 * DOI:
https://doi.org/10.1038/s41587-019-0225-9 SHARE THIS ARTICLE Anyone you share the following link with will be able to read this content: Get shareable link Sorry, a shareable link is not
currently available for this article. Copy to clipboard Provided by the Springer Nature SharedIt content-sharing initiative