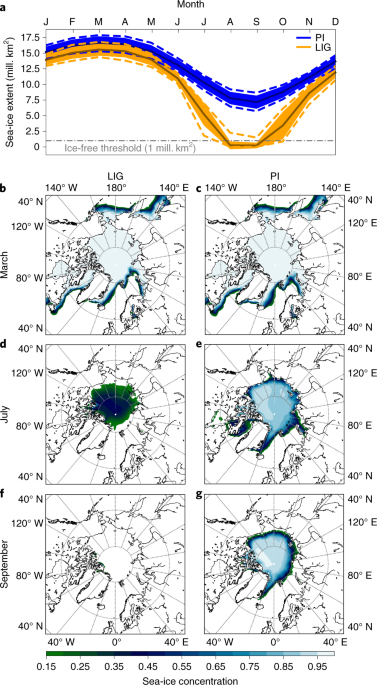
- Select a language for the TTS:
- UK English Female
- UK English Male
- US English Female
- US English Male
- Australian Female
- Australian Male
- Language selected: (auto detect) - EN
Play all audios:
ABSTRACT The Last Interglacial (LIG), a warmer period 130,000–116,000 years before present, is a potential analogue for future climate change. Stronger LIG summertime insolation at high
northern latitudes drove Arctic land summer temperatures 4–5 °C higher than in the pre-industrial era. Climate model simulations have previously failed to capture these elevated
temperatures, possibly because they were unable to correctly capture LIG sea-ice changes. Here, we show that the latest version of the fully coupled UK Hadley Center climate model (HadGEM3)
simulates a more accurate Arctic LIG climate, including elevated temperatures. Improved model physics, including a sophisticated sea-ice melt-pond scheme, result in a complete simulated loss
of Arctic sea ice in summer during the LIG, which has yet to be simulated in past generations of models. This ice-free Arctic yields a compelling solution to the long-standing puzzle of
what drove LIG Arctic warmth and supports a fast retreat of future Arctic summer sea ice. Access through your institution Buy or subscribe This is a preview of subscription content, access
via your institution ACCESS OPTIONS Access through your institution Access Nature and 54 other Nature Portfolio journals Get Nature+, our best-value online-access subscription $29.99 / 30
days cancel any time Learn more Subscribe to this journal Receive 12 print issues and online access $209.00 per year only $17.42 per issue Learn more Buy this article * Purchase on
SpringerLink * Instant access to full article PDF Buy now Prices may be subject to local taxes which are calculated during checkout ADDITIONAL ACCESS OPTIONS: * Log in * Learn about
institutional subscriptions * Read our FAQs * Contact customer support SIMILAR CONTENT BEING VIEWED BY OTHERS LONG- AND SHORT-TERM VARIABILITY OF ARCTIC SEA-ICE COVER DURING THE LAST
INTERGLACIAL AND MARINE ISOTOPE STAGE 11C Article Open access 08 April 2025 EAST ANTARCTIC WARMING FORCED BY ICE LOSS DURING THE LAST INTERGLACIAL Article Open access 03 February 2024
ACCELERATION OF WESTERN ARCTIC SEA ICE LOSS LINKED TO THE PACIFIC NORTH AMERICAN PATTERN Article Open access 09 March 2021 DATA AVAILABILITY The CMIP3-6 model data used in this study to
compute ECS and ice-free years are available from the Earth System Grid Federation (https://esgf-node.llnl.gov/). The HadCM3 and HadGEM3 model outputs used to support the findings of this
study are available from http://gws-access.ceda.ac.uk/public/pmip4/vittoria/CMIP6LIG_HadGEM3_CMIP3_HadCM3/. The HadGEM3 model outputs prepared for CMIP6 can be found at
https://doi.org/10.22033/ESGF/CMIP6.419 (ref. 54). The authors declare that all other data are available in the paper and its Supplementary Information. CODE AVAILABILITY The source code of
the HadCM3 model and the HadGEM3 model’s atmospheric component (Unified Model) is available under licence. To apply for a licence, go to
http://www.metoffice.gov.uk/research/modelling-systems/unified-model. JULES is available under licence free of charge; see https://jules-lsm.github.io/. The NEMO model code is available from
http://www.nemo-ocean.eu. The model code for CICE can be downloaded from https://code.metoffice.gov.uk/trac/cice/browser. CHANGE HISTORY * _ 30 AUGUST 2023 A Correction to this paper has
been published: https://doi.org/10.1038/s41558-023-01821-2 _ REFERENCES * Kaspar, F., Kühl, N., Cubasch, U. & Litt, T. A model–data comparison of European temperatures in the Eemian
interglacial. _Geophys. Res. Lett._ 32, L11703 (2005). Article Google Scholar * CAPE Last Interglacial Project Members. Last interglacial Arctic warmth confirms polar amplification of
climate change. _Quat. Sci. Rev._ 25, 1382–1400 (2006). * Capron, E. et al. Temporal and spatial structure of multi-millennial temperature changes at high latitudes during the last
interglacial. _Quat. Sci. Rev._ 103, 116–133 (2014). Article Google Scholar * Capron, E., Govin, A., Feng, R., Otto-Bliesner, B. L. & Wolff, E. W. Critical evaluation of climate
syntheses to benchmark CMIP6/PMIP4 127 ka last interglacial simulations in the high-latitude regions. _Quat. Sci. Rev._ 168, 137–150 (2017). Article Google Scholar * Hoffman, J. S., Clark,
P. U., Parnell, A. C. & He, F. Regional and global sea-surface temperatures during the last interglaciation. _Science_ 355, 276–279 (2017). Article CAS Google Scholar * Kopp, R. E.,
Simons, F. J., Mitrovica, J. X., Maloof, A. C. & Oppenheimer, M. Probabilistic assessment of sea level during the last interglacial stage. _Nature_ 462, 863–867 (2009). Article CAS
Google Scholar * Dutton, A. et al. Sea-level rise due to polar ice-sheet mass loss during past warm periods. _Science_ 349, aaa4019 (2015). Article CAS Google Scholar * Malmierca-Vallet,
I. et al. Simulating the last interglacial Greenland stable water isotope peak: the role of arctic sea ice changes. _Quat. Sci. Rev._ 198, 1–14 (2018). Article Google Scholar *
Masson-Delmotte, V. et al. Sensitivity of interglacial Greenland temperature and _δ_18_o_: ice core data, orbital and increased CO2 climate simulations. _Climate_ 7, 1041–1059 (2011). Google
Scholar * Otto-Bliesner, B. L. et al. How warm was the last interglacial? New model–data comparisons. _Phil. Trans. R. Soc. A_ 371, 20130097 (2013). Article Google Scholar * Lunt, D. J.
et al. A multi-model assessment of last interglacial temperatures. _Climate_ 9, 699–717 (2013). Google Scholar * Sime, L. C. et al. Warm climate isotopic simulations: what do we learn about
interglacial signals in Greenland ice cores? _Quat. Sci. Rev._ 67, 59–80 (2013). Article Google Scholar * Stein, R., Fahl, K., Gierz, P., Niessen, F. & Lohmann, G. Arctic Ocean sea
ice cover during the penultimate glacial and the last interglacial. _Nat. Commun._ 8, 373 (2017). Article Google Scholar * Brigham-Grette, J. & Hopkins, D. M. Emergent marine record
and paleoclimate of the last interglaciation along the northwest Alaskan coast. _Quat. Res._ 43, 159–173 (1995). Article Google Scholar * Nørgaard-Pedersen, N., Mikkelsen, N., Lassen, S.
J., Kristoffersena, Y. & Sheldon, E. Reduced sea ice concentrations in the Arctic Ocean during the last interglacial period revealed by sediment cores off northern Greenland.
_Paleoceanography_ 22, PA1218 (2007). Article Google Scholar * Adler, R. E. et al. Sediment record from the western Arctic Ocean with an improved Late Quaternary age resolution: HOTRAX
core HLY0503-8JPC, Mendeleev Ridge. _Glob. Planet. Change_ 68, 18–29 (2009). Article Google Scholar * Cronin, T. et al. Quaternary sea-ice history in the Arctic Ocean based on a new
ostracode sea-ice proxy. _Quat. Sci. Rev._ 29, 3415–3429 (2010). Article Google Scholar * Belt, S. Source-specific biomarkers as proxies for Arctic and Antarctic sea ice. _Org. Geochem._
125, 277–298 (2018). Article CAS Google Scholar * Otto-Bliesner, B. L. et al. Simulating Arctic climate warmth and icefield retreat in the last interglaciation. _Science_ 311, 1751–1753
(2006). Article CAS Google Scholar * Tatebe, H. et al. Description and basic evaluation of simulated mean state, internal variability, and climate sensitivity in MIROC6. _Geosci. Model
Dev._ 12, 2727–2765 (2019). Article CAS Google Scholar * Wu, T. et al. The Beijing Climate Center Climate System Model (BCC-CSM): the main progress from CMIP5 to CMIP6. _Geosci. Model
Dev._ 12, 1573–1600 (2019). Article Google Scholar * Gettelman, A. et al. High climate sensitivity in the Community Earth System Model version 2 (CESM2). _Geophys. Res. Lett._ 46,
8329–8337 (2019). Article Google Scholar * Voldoire, A. et al. Evaluation of CMIP6 deck experiments with CNRM-CM6-1. _J. Adv. Model. Earth Syst._ 11, 2177–2213 (2019). Article Google
Scholar * IPCC _Climate Change 2001: The Scientific Basis_ (eds Houghton, J. T. et al.) (Cambridge Univ. Press, 2001). * IPCC _Climate Change 2007: The Physical Science Basis_ (eds Solomon,
S. et al.) (Cambridge Univ. Press, 2007). * IPCC _Climate Change 2013: The Physical Science Basis_ (eds Stocker, T. F. et al.) (Cambridge Univ. Press, 2013). * Zelinka, M. D. et al. Causes
of higher climate sensitivity in CMIP6 models. _Geophys. Res. Lett._ 47, e2019GL085782 (2020). Article Google Scholar * Williams, K. et al. The Met Office Global Coupled Model 3.0 and 3.1
(GC3.0 and GC3.1) configurations. _J. Adv. Model. Earth Syst._ 10, 357–380 (2018). Article Google Scholar * Otto-Bliesner, B. L. et al. The PMIP4 contribution to CMIP6—part 2: two
interglacials, scientific objective and experimental design for Holocene and last interglacial simulations. _Geosci. Model Dev._ 10, 3979–4003 (2017). Article CAS Google Scholar * Gordon,
C. et al. The simulation of SST, sea ice extents and ocean heat transports in a version of the Hadley Centre coupled model without flux adjustments. _Clim. Dyn._ 16, 147–168 (2000). Article
Google Scholar * Flocco, D., Schroeder, D., Feltham, D. L. & Hunke, E. C. Impact of melt ponds on Arctic sea ice simulations from 1990 to 2007. _J. Geophys. Res. Oceans_ 117, C09032
(2012). Article Google Scholar * Schröder, D., Feltham, D. L., Flocco, D. & Tsamados, M. September Arctic sea-ice minimum predicted by spring melt-pond fraction. _Nat. Clim. Change_ 4,
353–257 (2014). Article Google Scholar * Perovich, D. K. et al. Increasing solar heating of the Arctic Ocean and adjacent seas, 1979–2005: attribution and role in the ice-albedo feedback.
_Geophys. Res. Lett._ 34, L19505 (2007). Article Google Scholar * Rösel, A. & Kaleschke, L. Exceptional melt pond occurrence in the years 2007 and 2011 on the Arctic sea ice revealed
from MODIS satellite data. _J. Geophys. Res. Oceans_ 117, C05018 (2012). Article Google Scholar * Andrews, T. et al. Forcings, feedbacks, and climate sensitivity in HadGEM3-GC3.1 and
UKESM1. _J. Adv. Model. Earth Syst._ 11, 4377–4394 (2019). Article Google Scholar * Rosenblum, E. & Eisenman, I. Sea ice trends in climate models only accurate in runs with biased
global warming. _J. Climate_ 30, 6265–6278 (2017). Article Google Scholar * Mahlstein, I. & Knutti, R. September Arctic sea ice predicted to disappear near 2 C global warming above
present. _J. Geophys. Res. Atmos._ 117, 0026 (2012). Article Google Scholar * Stroeve, J. & Notz, D. Insights on past and future sea-ice evolution from combining observations and
models. _Glob. Planet. Change_ 135, 119–132 (2015). Article Google Scholar * Belt, S. T. & Müller, J. The Arctic sea ice biomarker IP25: a review of current understanding,
recommendations for future research and applications in palaeo sea ice reconstructions. _Quat. Sci. Rev._ 79, 9–25 (2013). Article Google Scholar * Xiao, X., Stein, R. & Fahl, K. MIS 3
to MIS 1 temporal and LGM spatial variability in Arctic Ocean sea ice cover: reconstruction from biomarkers. _Paleoceanography_ 30, 969–983 (2015). Article Google Scholar * Govin, A. et
al. Sequence of events from the onset to the demise of the last interglacial: evaluating strengths and limitations of chronologies used in climatic archives. _Quat. Sci. Rev._ 129, 1–36
(2015). Article Google Scholar * Turney, C. S. & Jones, R. T. Does the Agulhas Current amplify global temperatures during super-interglacials? _J. Quat. Sci._ 25, 839–843 (2010).
Article Google Scholar * Berger, A. & Loutre, M.-F. Insolation values for the climate of the last 10 million years. _Quat. Sci. Rev._ 10, 297–317 (1991). Article Google Scholar *
Walters, D. et al. The Met Office Unified Model Global Atmosphere 6.0/6.1 and Jules Global Land 6.0/6.1 configurations. _Geosci. Model Dev._ 10, 1487–1520 (2017). Article Google Scholar *
Madec, G. et al. _Nemo Ocean Engine_ (Institut Pierre-Simon Laplace, 2015). * Ridley, J. K. et al. The sea ice model component of HadGEM3-GC3.1. _Geosci. Model Dev._ 11, 713–723 (2018).
Article Google Scholar * Cox, P. M. _Description of the ‘TRIFFID’ Dynamic Global Vegetation Model_ (Hadley Centre for Climate Prediction and Research, 2001). * Cox, P., Huntingford, C.
& Harding, R. A canopy conductance and photosynthesis model for use in a GCM land surface scheme. _J. Hydrol._ 212, 79–94 (1998). Article Google Scholar * Menary, M. B. et al.
Preindustrial control simulations with HadGEM3-GC3.1 for CMIP6. _J. Adv. Model. Earth Syst._ 10, 3049–3075 (2018). Google Scholar * Guarino, M. V., Sime, L., Schroeder, D., Lister, G. &
Hatcher, R. Machine dependence and reproducibility for coupled climate simulations: the HadGEM3-GC3.1 CMIP preindustrial simulation. _Geosci. Model Dev._ 13, 139–154 (2020). Article Google
Scholar * Gregory, J. M. et al. A new method for diagnosing radiative forcing and climate sensitivity. _Geophys. Res. Lett._ 31, L03205 (2004). Article Google Scholar * Gettelman, A.,
Kay, J. & Shell, K. The evolution of climate sensitivity and climate feedbacks in the community atmosphere model. _J. Climate_ 25, 1453–1469 (2012). Article Google Scholar * O’Neill,
B. C. et al. The Scenario Model Intercomparison Project (ScenarioMIP) for CMIP6. _Geosci. Model Dev._ 9, 3461–3482 (2016). Article Google Scholar * Ridley, J. et al. _MOHC HadGEM3-GC31-LL
Model Output Prepared for CMIP6_ (Earth System Grid Federation, 2018); https://doi.org/10.22033/ESGF/CMIP6.419 Download references ACKNOWLEDGEMENTS M.-V.G. acknowledges support from NERC
research grant no. NE/P013279/1. L.C.S. acknowledges support through grant nos NE/P013279/1, NE/P009271/1 and EU-TiPES. The project has received funding from the European Union’s Horizon
2020 research and innovation programme under grant agreement no. 820970. D.S. acknowledges support from the NERC-UKESM program. I.M.-V. acknowledges support from a NERC PhD studentship and
EU-TiPES. E.W. is supported by a Royal Society Research Professorship. E.J.S. and C.B. acknowledge support by the US National Science Foundation through NSFGEO-NERC award no. 1602435. J.S
acknowledges support from the Canada 150 Research Chairs program, C150 grant no. 50296. This work used the ARCHER UK National Supercomputing Service (http://www.archer.ac.uk) and the JASMIN
data analysis platform (http://jasmin.ac.uk/). In addition, we thank S. Belt for helpful discussions on IP25 LIG sea-ice interpretations, B. Otto-Bliesner for providing the published CMIP5
LIG summer temperature observations and NCAS for supporting the LIG model simulations. AUTHOR INFORMATION Author notes * These authors contributed equally: Maria-Vittoria Guarino, Louise C.
Sime. AUTHORS AND AFFILIATIONS * British Antarctic Survey, Cambridge, UK Maria-Vittoria Guarino, Louise C. Sime & Irene Malmierca-Vallet * Department of Meteorology, University of
Reading, Reading, UK David Schröeder & Danny Feltham * Centre of Earth Observation Science, University of Manitoba, Winnipeg, Manitoba, Canada Erica Rosenblum & Julienne Stroeve *
The Met Office, Exeter, UK Mark Ringer, Jeff Ridley & Alistair Sellar * Department of Atmospheric Sciences, University of Washington, Seattle, WA, USA Cecilia Bitz & Eric J. Steig *
Department of Earth and Space Sciences, University of Washington, Seattle, WA, USA Eric J. Steig * Department of Earth Sciences, University of Cambridge, Cambridge, UK Eric Wolff Authors *
Maria-Vittoria Guarino View author publications You can also search for this author inPubMed Google Scholar * Louise C. Sime View author publications You can also search for this author
inPubMed Google Scholar * David Schröeder View author publications You can also search for this author inPubMed Google Scholar * Irene Malmierca-Vallet View author publications You can also
search for this author inPubMed Google Scholar * Erica Rosenblum View author publications You can also search for this author inPubMed Google Scholar * Mark Ringer View author publications
You can also search for this author inPubMed Google Scholar * Jeff Ridley View author publications You can also search for this author inPubMed Google Scholar * Danny Feltham View author
publications You can also search for this author inPubMed Google Scholar * Cecilia Bitz View author publications You can also search for this author inPubMed Google Scholar * Eric J. Steig
View author publications You can also search for this author inPubMed Google Scholar * Eric Wolff View author publications You can also search for this author inPubMed Google Scholar *
Julienne Stroeve View author publications You can also search for this author inPubMed Google Scholar * Alistair Sellar View author publications You can also search for this author inPubMed
Google Scholar CONTRIBUTIONS L.C.S. oversaw the direction and formulation of the research. M.-V.G. carried out the HadGEM3 simulations and analysed all simulation results. D.S. helped guide
the interpretation of the simulation results. I.M.-V. ran the HadCM3 simulations. E.R. helped with the CMIP3-6 projected sea-ice-free analysis. M.R. computed the CMIP6 ECS data. J.R.
assisted with the HadGEM3 data post-processing. All authors read the manuscript and provided comments. L.C.S. and M.-V.G. wrote the manuscript. CORRESPONDING AUTHORS Correspondence to
Maria-Vittoria Guarino or Louise C. Sime. ETHICS DECLARATIONS COMPETING INTERESTS The authors declare no competing interests. ADDITIONAL INFORMATION PUBLISHER’S NOTE Springer Nature remains
neutral with regard to jurisdictional claims in published maps and institutional affiliations. SUPPLEMENTARY INFORMATION SUPPLEMENTARY INFORMATION Supplementary Figs. 1–14 and Tables 1–4.
RIGHTS AND PERMISSIONS Springer Nature or its licensor (e.g. a society or other partner) holds exclusive rights to this article under a publishing agreement with the author(s) or other
rightsholder(s); author self-archiving of the accepted manuscript version of this article is solely governed by the terms of such publishing agreement and applicable law. Reprints and
permissions ABOUT THIS ARTICLE CITE THIS ARTICLE Guarino, MV., Sime, L.C., Schröeder, D. _et al._ Sea-ice-free Arctic during the Last Interglacial supports fast future loss. _Nat. Clim.
Chang._ 10, 928–932 (2020). https://doi.org/10.1038/s41558-020-0865-2 Download citation * Received: 06 December 2019 * Accepted: 07 July 2020 * Published: 10 August 2020 * Issue Date:
October 2020 * DOI: https://doi.org/10.1038/s41558-020-0865-2 SHARE THIS ARTICLE Anyone you share the following link with will be able to read this content: Get shareable link Sorry, a
shareable link is not currently available for this article. Copy to clipboard Provided by the Springer Nature SharedIt content-sharing initiative