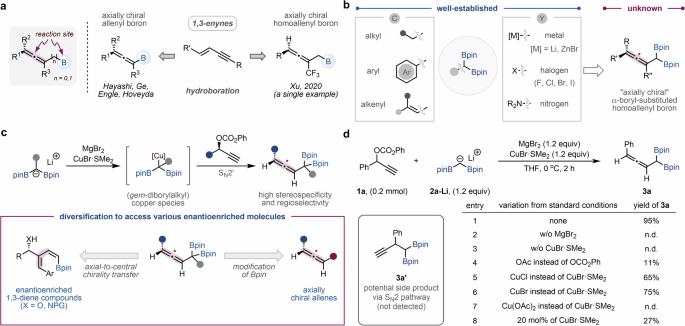
- Select a language for the TTS:
- UK English Female
- UK English Male
- US English Female
- US English Male
- Australian Female
- Australian Male
- Language selected: (auto detect) - EN
Play all audios:
ABSTRACT Axially chiral allenes bearing organoboron groups are highly sought-after building blocks in organic synthesis due to their potential for generating a wide range of axially and
centrally chiral molecules. However, the existing methods for preparing axially chiral allenes containing boron group are primarily limited to the synthesis of allenyl boronic esters, and
strategies for accessing axially chiral homoallenyl boronic esters are still scarce. Here, we report the general method for synthesizing axially chiral α-boryl-homoallenyl boronic esters
through a highly regio- and stereoselective copper-mediated SN2’-addition of newly prepared (diborylalkyl)copper species to chiral propargyl electrophiles. The reaction conditions were
optimized to achieve high yields and excellent stereospecificity. The obtained products were successfully transformed into various axially chiral allenes and other chiral molecules by
transforming diboron units. The potential for axial-to-central chirality transfer of axially chiral α-boryl-homoallenyl boronic esters is also demonstrated through the stereospecific
addition to aldehydes and N-H aldimines, yielding enantioenriched 1,2-oxaborinin-3,5-dienes and 2-aminomethyl-1,3-dienes. SIMILAR CONTENT BEING VIEWED BY OTHERS ENANTIOSELECTIVE CONSTRUCTION
OF C-B AXIALLY CHIRAL ALKENYLBORONS BY NICKEL-CATALYZED RADICAL RELAYED REDUCTIVE COUPLING Article Open access 30 November 2024 SYNTHESIS OF AXIALLY CHIRAL ALKENYLBORONATES THROUGH COMBINED
COPPER- AND PALLADIUM-CATALYSED ATROPOSELECTIVE ARYLBORATION OF ALKYNES Article 05 January 2023 NI-CATALYSED ASSEMBLY OF AXIALLY CHIRAL ALKENES FROM ALKYNYL TETRACOORDINATE BORONS VIA
1,3-METALLATE SHIFT Article 05 January 2024 INTRODUCTION Axially chiral allenes play an important role in synthetic chemistry due to their unique chemical reactivity and inherent axial
chirality1,2,3,4,5. These features drive advancements in asymmetric synthesis and facilitate the development of chiral catalysts, materials, and biologically active compounds6,7,8. Among
these, axially chiral allenes bearing organoboron groups are of particular importance as they enable the synthesis of a wide range of enantioenriched compounds through transformations that
exploit both the boron group and the allene moiety9,10,11,12,13. Recognizing the potential of these valuable synthetic intermediates, several research groups have focused their efforts on
developing methods for synthesizing axially chiral allenes with organoboron groups (Fig. 1a). However, the majority of research efforts have been centered on the preparation of axially
chiral allenyl borons through transition metal-catalyzed enantioselective hydroboration of 1,3-enynes14,15,16,17. In contrast, strategies for accessing axially chiral homoallenyl borons
remain largely unexplored. In 2020, Xu and co-workers developed the sole method for generating axially chiral homoallenyl borons via a copper-catalyzed enantioselective protoborylation of
CF3-substituted 1,3-enynes. However, this approach was limited to the exclusive formation of CF3-substituted axially chiral homoallenyl mono-boronic esters18. _gem_-Diboron compounds have
emerged as valuable intermediates in synthetic chemistry owing to their applicability in transition metal-catalyzed19,20,21,22,23,24,25 or transition metal-free26,27,28,29 carbon−carbon bond
formation reactions. Consequently, significant progress has been made in the synthesis of _gem_-diboron compounds containing various substituents at the α-carbon, such as alkyl19,20,21,
aryl30,31,32,33,34,35, alkenyl36,37,38,39,40,41, metal42,43,44,45,46, and heteroatom47,48,49,50 groups. Despite these substantial advancements, the synthesis of _gem_-diborons bearing
allenyl groups at the α-carbon, particularly axially chiral ones, remains a formidable challenge (Fig. 1b). Herein, we report the method for synthesizing axially chiral α-boryl-homoallenyl
borons through SN2’-addition of (_gem_-diborylalkyl)copper species, generated in situ by transmetalation of (_gem_-diborylalkyl)lithiums and copper(I) halide, to chiral propargylic
carbonates (Fig. 1c). The developed reaction yields axially chiral α-boryl-homoallenyl borons, a new class of diboron compounds with excellent regio- and stereospecificity. We demonstrate
the versatility of these compounds by subjecting them to various stereospecific transformations, affording a wide range of axially and centrally enantioenriched molecules, thereby
highlighting the potential of axially chiral α-boryl-homoallenyl borons as valuable chiral building blocks. RESULTS AND DISCUSSION SYNTHESIS OF Α-BORYL-HOMOALLENYL BORONIC ESTERS Recently,
our group devised a highly efficient technique for isolating (diborylmethyl)lithium from diborylmethane using lithium diisopropylamide (LDA) as a base43. Considering that copper-mediated
SN2’-selective addition of nucleophiles to propargyl electrophiles is well-established for producing various allenes10,11,12,51,52,53,54,55, we hypothesized that the generation of
(diborylmethyl)copper species through the transmetalation of (diborylmethyl)lithium with copper(I) halide could facilitate SN2’-selective addition to propargyl electrophiles, resulting in
α-boryl-homoallenyl borons. After extensive optimization studies (Fig. 1d), we found that the reaction of phenyl-(1-phenylprop-2-yn-1-yl) carbonate (1A) with isolated (diborylmethyl)lithium
(2A-LI) in the presence of 1.2 equivalents of MgBr2 and CuBr•SMe2 in THF at 0 °C afforded the corresponding α-boryl-homoallenyl boronic ester (3A) with an excellent yield (95 %) and a
complete SN2’-selectivity (entry 1). It should be noted that the SN2-selective product 3A’ was not detected under these reaction conditions. Control experiments revealed that product 3A was
not formed in the absence of either MgBr2 or CuBr•SMe2 (entries 2 and 3). These results suggest that (diborylmethyl)magnesium bromide is initially generated through the transmetalation of
2A-LI with MgBr2. The magnesium species then undergoes an additional transmetalation with CuBr•SMe2 to produce the (diborylmethyl)copper species56,57,58,59, which subsequently reacts with 1A
to yield product 3A. The yield of 3A decreased when the leaving group of the propargyl electrophile was changed from phenyl carbonate to acetate (entry 4). The use of other copper(I) salts
such as CuCl and CuBr proved to be less effective than CuBr•SMe2 (entries 5 and 6). No reaction occurred when Cu(OAc)2 was used instead of CuBr•SMe2 (entry 7). Furthermore, using a catalytic
amount of copper resulted in a significantly reduced the yield of 3A (entry 8). With the optimized conditions in hand, we synthesized a variety of α-boryl-homoallenyl boronic esters to
demonstrate the versatility of the developed method (Fig. 2a). In most cases, the isolated yields were generally lower than the 1H-NMR yields due to the instability of the synthesized
α-boryl-homoallenyl boronic esters. To obtain the desired products in high purity, a two-step chromatographic purification process was necessary. The crude mixture was first passed through a
boric acid-capped silica (B-SiO2)60 column to remove the unreacted electrophile and other impurities, followed by a short silica gel (2.5 cm) column chromatography to isolate the products.
Although this two-step purification process resulted in a decrease in the isolated yields, it was crucial for ensuring the high purity of the obtained α-boryl-homoallenyl boronic esters. It
is worth noting that the crude α-boryl-homoallenyl boronic esters can be directly used for further transformations without isolation (_vide infra_), highlighting the practicality and
efficiency of the developed method. Despite the challenges associated with product isolation, our method enabled the synthesis of a wide range of α-boryl-homoallenyl boronic esters,
demonstrating its broad applicability. Aryl-substituted propargyl carbonates bearing various substituents at different positions of the phenyl ring (C2, C3, or C4) underwent smooth
SN2’-selective addition, affording the desired products (3B-3L) in good yields. Moreover, the reaction tolerated naphthyl and thiophene-containing propargyl carbonates, delivering the
corresponding the products 3M and 3N, respectively. Aliphatic propargyl carbonates also proved to be suitable substrates, furnishing the desired products 3O and 3P in moderate yields.
Furthermore, a cyclohexyl-substituted propargyl carbonate afforded the product 3Q in moderate yield at room temperature. Alkyl substituted _gem_-diborylalkanes could also be employed as
competent nucleophiles, providing access to the corresponding α-boryl-homoallenyl boronic esters 3R-3T. Unfortunately, the use of an internal propargyl carbonate as an electrophile did not
provided the product 3U, showing a limitation of this protocol. Next, we sought to develop a stereospecific SN2’-addition of in situ generated (diborylalkyl)copper species to enantioenriched
propargyl carbonates to synthesize axially chiral α-boryl-homoallenyl boronic esters. Gratifyingly, when (_S_)-phenyl-(1-phenylprop-2-yn-1-yl) carbonate [(_S_)-1A, >99:1 er] and 2A-LI
were treated with MgBr2 and CuBr•SMe2 under the reaction conditions, the desired axially chiral α-boryl-homoallenyl boronic ester (_S_)-3A was obtained in good yield with high
stereospecificity (98:2 er, 96% es). The absolute stereochemistry of (_S_)−3A was determined by converting it to a known allenyl alcohol through a two-step sequence involving the
protodeboronation of one of the Bpin groups, followed by the oxidation of the resulting homoallenyl mono-boronic ester. The specific rotation value of the obtained allenyl alcohol was then
compared with the reported data (See the Supplementary information for details). To further demonstrate the potential of this method, we explored the scope of chiral propargyl carbonates
(Fig. 2b). Chiral propargyl carbonates bearing various substitution patterns on the aryl group were well-tolerated, furnishing products (_S_)−3D, (_S_)-3J, and (_S_)-3L with high efficiency
and excellent stereocontrol. A naphthyl-containing chiral propargyl carbonate was successfully incorporated, delivering (_S_)-3M in good yield and stereospecificity. When aliphatic
substituted chiral propargyl carbonate, such as (_R_)-phenyl (5-phenylpent-1-yn-3-yl) carbonate, was reacted with 2A-LI under the reaction conditions, the product (_S_)-3O was obtained in
good yield, albeit with moderate stereospecificity (58% es). Moreover, the reaction conditions proved compatible with in situ generated (_gem_-diborylethyl)copper species, providing (_S_)-3R
with complete retention of stereochemistry. STEREOSPECIFIC 1,3-DIENYLATION WITH ALDEHYDES AND N-H ALDIMINES Enantioenriched cyclic boronic esters are important structural motifs found in a
wide range of natural products and pharmaceutically relevant compounds (Fig. 3a)61,62. As a result, the development of efficient methods for their synthesis has been a subject of intense
research in recent years. Thus, we directed our attention towards developing a reaction that would enable the addition of the axially chiral α-boryl-homoallenyl boronic ester (_S_)-3A to
various aldehydes through a chemoselective and stereospecific process, yielding the corresponding enantioenriched 1,2-oxaborinin-3,5-dienes63,64,65,66. Due to the low yields obtained during
the isolation of the axially chiral α-boryl-homoallenyl boronic esters, which was attributed to their partial decomposition during column chromatography, we developed a one-pot reaction by
directly subjecting the crude (_S_)-3A to various aldehydes. We were pleased to find that the reaction of crude (_S_)-3A with benzaldehyde in toluene at room temperature afforded the
corresponding enantioenriched 1,2-oxaborinin-3,5-diene (4A) with an excellent yield and stereospecificity. The observed stereochemical outcome of the 1,3-dienylation reaction can be
rationalized by considering the Zimmerman–Traxler transition-state model (Fig. 3b)66,67, which proposes a cyclic six-membered ring transition state for the addition of allylmetal reagents to
aldehydes. Although the addition of (_S_)-α-boryl-homoallenyl boronic esters (_S_)−3A to an aldehyde can potentially proceed through various transition states (See the Supplementary
Information for details), we have selected four representative transition states (TS-1 to TS-4) to illustrate the key factors influencing the stereoselectivity. Among these representative
transition states, TS-1 is the most favorable as it does not involve any apparent steric interactions. In contrast, TS-2 suffers from destabilizing gauche interactions between the
pseudo-equatorially oriented Bpin group and the pinacol moiety on the boron group. Both TS-3 and TS-4 encounter unfavorable steric interactions between the pseudo-axially oriented phenyl
group of benzaldehyde and the pinacol moiety on the boron group. Consequently, the reaction proceeds predominantly through TS-1, leading to the formation of the observed product 4 with high
stereoselectivity. The reactions of (_S_)−3A with various aromatic aldehydes bearing substituents at the _para_-, _meta_-, and _ortho_-positions of the arene ring afforded the corresponding
products 4B-4G with excellent yields and stereospecificity (Fig. 3c). When 2-naphthaldehyde was subjected to the 1,3-dienylation conditions, the product 4H was successfully produced.
Moreover, an aliphatic aldehyde such as hydrocinnamaldehyde was also a suitable substrate, yielding 4I efficiently. Axially chiral α-boryl-homoallenyl boronic esters (_S_)-3D, (_S_)-3J, and
(_S_)-3M were readily reacted with benzaldehyde to give access to the products 4J-4L. We next focused on developing a chemo- and stereospecific addition of crude axially chiral
α-boryl-homoallenyl boronic esters to N-H aldimines. Enantioenriched 2-aminomethyl-1,3-dienes are valuable building blocks for synthesizing biologically active molecules; however, methods
for their enantioselective synthesis are scarce. Although the addition of homoallenyl boronic esters to imines offers a promising approach to access these compounds, this transformation
remains rarely investigated, and the few reported examples exhibit moderate enantioselectivity, limiting their practical utility67. To address this challenge, we sought to develop a highly
chemo- and stereoselective variant of this reaction, enabling efficient access to enantioenriched 2-aminomethyl-1,3-dienes. The reaction of (_S_)-3A with in-situ generated phenyl N-H
aldimine from the corresponding aldehyde and ammonia in ethanol, followed by benzoyl protection to facilitate isolation, afforded the desired 2-aminomethyl-1,3-diene bearing a (_Z_)-vinyl
Bpin group (5A) in excellent yield and stereospecificity (Fig. 4). Since no other stereoisomers were detected under the reaction conditions, this reaction was believed to proceed in the same
manner as the reaction with aldehydes. Other in-situ generated N-H aldimines, derived from benzaldehyde derivatives, readily participated in the chemo- and stereospecific reaction with
(_S_)−3A, affording 5B-5I in good yields with high stereospecificity. Cinnamaldehyde also smoothly underwent the reaction, giving 5J with moderate stereospecificity. The reaction of phenyl
N-H aldimine with axially chiral α-boryl-homoallenyl boronic esters (_S_)−3D and (_S_)-3L delivered the products 5K and 5L, respectively. SYNTHETIC APPLICATIONS FOR THE SYNTHESIS OF
CENTRALLY AND AXIALLY CHIRAL MOLECULES The obtained axially chiral α-boryl-homoallenyl boronic esters can be transformed into various axially chiral allenes and other chiral compounds
through further modifications of the diboron unit (Fig. 5a–c). For example, oxidation of the diboron groups in (_S_)-3A or (_S_)-3R with 3-chloroperoxybenzoic acid (_m_CPBA) afforded the
corresponding allenyl aldehyde 6 (96:4 er) or allenyl ketone 7 (97:3 er) while retaining axial chirality68. Chemoselective protodeboronation of (_S_)-3A yielded the axially mono-boron
compound with excellent regioselectivity, which was further transformed into alkylboronic ester 8 (97:3 er) through a one-carbon homologation reaction with LiCH2Cl. Oxidation of the
homoallenyl mono-boron compound with H2O2 gave the corresponding alcohol 9 (98:2 er)69, which underwent intramolecular bromocyclization in the presence of bromine to form
3-bromo-2-phenyl-2,5-dihydrofuran 10. Subsequent Pd-catalyzed cross-coupling of 10 with _p_-anisylboronic acid delivered the compound 11 (96:4 er). To showcase the scalability and practical
value of our methodology, we performed a gram-scale synthesis of compound 4A (Fig. 5d). Under the optimized reaction conditions, 2A-LI and (_S_)-1A were converted to (_S_)-3A, which
subsequently underwent a 1,3-dienylation with 5 mmol of benzaldehyde to furnish 4A in 80% yield (1.05 g) with excellent enantioselectivity (98:2 er). The boron group of the enantioenriched
product 4A can be transformed into other functionalities through further modifications. For example, Pd-catalyzed Suzuki- Miyaura cross-coupling of 4A with 4-iodoanisole yielded the product
12 in good yield with high stereoselectivities (98:2 er, 1: > 20 _E_/_Z_). Oxidation of the boron group of 4A resulted in the formation of hemiacetal, which could be further transformed
into the corresponding alcohol 13 by treatment with NaBH4 in 84% yield over two steps with good stereoselectivity (98:2 er). The obtained 2-aminomethyl-1,3-diene 5A could also be transformed
into other enantioenriched product. Oxidation of the Bpin group in 5A by treatment with sodium perborate generated the cyclic N,O-hemiaminal 5A’. Subsequent reduction of 5A’ with sodium
borohydride afforded the enantioenriched 1,4-aminoalcohol 15 in 77% yield with 98:2 er. In summary, we have developed the method for synthesizing axially chiral α-boryl-homoallenyl boronic
esters through a regio- and stereoselective copper-mediated SN2’-type addition of (diborylalkyl)copper species to chiral propargyl electrophiles. The obtained axially chiral
α-boryl-homoallenyl boronic esters exhibit high versatility, as they can be transformed into a wide range of axially chiral allenes and other chiral compounds through further modifications
of the _gem_-diboron unit, highlighting their potential for diverse applications. Moreover, we have successfully achieved axial-to-central chirality transfer through the chemoselective and
stereospecific addition of axially chiral α-boryl-homoallenyl boronic esters to aldehydes and N-H aldimines, yielding enantioenriched 1,2-oxaborinin-3,5-dienes and 2-aminomethyl-1,3-dienes.
We anticipate that the developed methodologies will significantly expand the synthetic toolbox for constructing various chiral architectures. Our ongoing studies aim to develop transition
metal-catalyzed chemo- and stereoselective transformations of racemic α-boryl-homoallenyl boronic esters. METHODS GENERAL PROCEDURE FOR THE SYNTHESIS OF AXIALLY CHIRAL Α-BORYL-HOMOALLENYL
BORONIC ESTERS In a nitrogen-filled glove-box, isolated _gem_-(diborylalkyl)lithium 2-LI (0.24 mmol, 1.2 equiv), anhydrous magnesium bromide (44.2 mg, 0.24 mmol, 1.2 equiv), copper(I)
bromide-dimethyl sulfide complex (49.3 mg, 0.24 mmol, 1.2 equiv), and anhydrous THF (0.8 mL) were added to a 4.0 mL dram vial with a Teflon coated magnetic stir bar. The vial was sealed with
a PTFE/silicone-lined septum cap and stirred for 30 min at room temperature. After removing from the glove box, the vial was cooled to 0 oC. To this mixture, propargyl carbonate 1 (0.2
mmol, 1.0 equiv) in anhydrous THF (0.4 mL) was slowly added at 0 oC and stirred at 0 oC for 2 h. The reaction mixture was diluted with CH2Cl2 (15 mL), quenched with saturated aqueous NH4Cl
(15 mL) solution, and the aqueous layer was extracted with CH2Cl2 (15 mL x 3). The combined organic layers were dried over MgSO4, filtered, and concentrated under reduced pressure. The crude
mixture was passed through B-SiO2 (16 cm, 70 mL of _n_-hexane:CH2Cl2 = 1:2) to remove the remaining electrophile and other impurities. After washing that B-SiO2 with diethyl ether, the
filtrate mixture which contains homoallenylic _gem_-diboronic ester was purified by short column chromatography on silica gel (2.5 cm, _n_-hexane:CH2Cl2 = 1:2) to afford the desired product.
GENERAL PROCEDURE FOR THE CHEMOSELECTIVE AND STEREOSPECIFIC 1,3-DIENYLATION OF (_S_)−3 WITH ALDEHYDES In a nitrogen-filled glove-box, crude axially chiral α-boryl-homoallenyl boronic esters
(_S_)−3 (synthesized by GP6 in 0.2 mmol scale) and anhydrous toluene (0.5 mL) were added to a 4.0 mL dram vial with a Teflon coated magnetic stir bar. The vial was sealed with a
PTFE/silicone-lined septum cap and removed from the glove box. To this mixture, the corresponding aldehyde (0.1 mmol) was added and stirred at room temperature for 12 h. The reaction mixture
was filtered through a pad of silica/MgSO4, washed with Et2O (50 mL), and concentrated under reduced pressure. The crude mixture was purified by column chromatography on silica gel to
afford the desired product. GENERAL PROCEDURE FOR THE CHEMOSELECTIVE AND STEREOSPECIFIC 1,3-DIENYLATION OF (_S_)-3 WITH N-H ALDIMINES In a nitrogen-filled glove-box, corresponding aldehyde
(0.1 mmol) and anhydrous THF (0.6 mL) were added to a 4.0 mL dram vial with a Teflon coated magnetic stir bar. The vial was sealed with a PTFE/silicone-lined septum cap and removed from the
glove box. To this mixture, ammonia solution (2.0 M in EtOH, 0.06 mL, 0.12 mmol, 1.2 equiv) was added. After stirring at room temperature for 5 h, crude axially chiral α-boryl-homoallenyl
boronic esters (_S_)-3 (synthesized by GP6 in 0.2 mmol scale) in anhydrous toluene (0.4 mL) was added. The reaction mixture was heated to 50 oC and stirred for 17 h. Upon completion, The
solution was cooled to 0 oC. After adding triethylamine (27.9 μL, 0.2 mmol, 2.0 equiv) and benzoyl chloride (23.2 μL, 0.2 mmol, 2.0 equiv), the reaction mixture was heated to room
temperature and stirred for 1 h. The reaction mixture was filtered through a pad of celite, washed with Et2O (50 mL), and concentrated under reduced pressure. The crude mixture was purified
by column chromatography on silica gel to afford the desired product. DATA AVAILABILITY Experimental procedures, characterization of the compounds are available in the Supplementary
Information. Crystallographic data for the compound 5A is available in the Supplementary Information and from the Cambridge Crystallographic Data Centre (CCDC) under the reference number
2360524. The data can be obtained free of charge via https://www.ccdc.cam.ac.uk/structures/. Data supporting the findings of this manuscript are also available from the authors upon request
REFERENCES * Alcaide, B., Almendros, P. & Aragoncillo, C. Exploiting [2+2] cycloaddition chemistry: achievements with allenes. _Chem. Soc. Rev._ 39, 783–816 (2010). Article CAS PubMed
Google Scholar * Krause, N. & Winter, C. Gold-Catalyzed Nucleophilic Cyclization of Functionalized Allenes: A Powerful Access to Carbo- and Heterocycles. _Chem. Rev._ 111, 1994–2009
(2011). Article CAS PubMed Google Scholar * Ye, J. & Ma, S. Palladium-Catalyzed Cyclization Reactions of Allenes in the Presence of Unsaturated Carbon-Carbon Bonds. _Acc. Chem. Res._
47, 989–1000 (2014). Article CAS PubMed Google Scholar * Neff, R. K. & Frantz, D. E. Recent applications of chiral allenes in axial-to-central chirality transfer reactions.
_Tetrahedron_ 71, 7–18 (2015). Article CAS Google Scholar * Alonso, J. M., Quirós, M. T. & Muñoz, M. P. Chirality transfer in metal-catalysed intermolecular addition reactions
involving allenes. _Org. Chem. Front._ 3, 1186–1204 (2016). Article CAS Google Scholar * Hoffmann-Röder, A. & Krause, N. Synthesis and Properties of Allenic Natural Products and
Pharmaceuticals. _Angew. Chem., Int. Ed._ 43, 1196–1216 (2004). Article Google Scholar * Rivera-Fuentes, P. & Diederich, F. Allenes in Molecular Materials. _Angew. Chem., Int. Ed._ 51,
2818–2828 (2012). Article CAS Google Scholar * Yu, S. & Ma, S. Allenes in Catalytic Asymmetric Synthesis and Natural Product Syntheses. _Angew. Chem., Int. Ed._ 51, 3074–3112 (2012).
Article CAS Google Scholar * Shimizu, M., Kurahashi, T., Kitagawa, H. & Hiyama, T. gem-Silylborylation of an sp Carbon: Novel Synthesis of 1-Boryl-1-silylallenes. _Org. Lett._ 5,
225–227 (2003). Article CAS PubMed Google Scholar * Ito, H., Sasaki, Y. & Sawamura, M. Copper(I)-Catalyzed Substitution of Propargylic Carbonates with Diboron: Selective Synthesis of
Multisubstituted Allenylboronates. _J. Am. Chem. Soc._ 130, 15774–15775 (2008). Article CAS PubMed Google Scholar * Chen, M. & Roush, W. R. Enantioselective Synthesis of anti- and
syn-Homopropargyl Alcohols via Chiral Brønsted Acid Catalyzed Asymmetric Allenylboration Reactions. _J. Am. Chem. Soc._ 134, 10947–10952 (2012). Article CAS PubMed PubMed Central Google
Scholar * Wang, B. et al. Cu-Catalyzed SN2′ Substitution of Propargylic Phosphates with Vinylarene-Derived Chiral Nucleophiles: Synthesis of Chiral Allenes. _Org. Lett._ 21, 3913–3917
(2019). Article CAS PubMed Google Scholar * Manna, S., Das, K. K., Aich, D. & Panda, S. Synthesis and Reactivity of Allenylboron Compounds. _Adv. Synth. Cat._ 363, 2444–2463 (2021).
Article CAS Google Scholar * Matsumoto, Y., Naito, M., Uozumi, Y. & Hayashi, T. Axially chiral allenylboranes: catalytic asymmetric synthesis by palladium-catalysed hydroboration of
but-1-en-3-ynes and their reaction with an aldehyde. _J. Chem. Soc., Chem. Commun_. 1993, 1468−1469. * Gao, D.-W. et al. Catalytic, Enantioselective Synthesis of Allenyl Boronates. _ACS
Catal._ 8, 3650–3654 (2018). Article CAS PubMed PubMed Central Google Scholar * Huang, Y., del Pozo, J., Torker, S. & Hoveyda, A. H. Enantioselective Synthesis of Trisubstituted
Allenyl–B(pin) Compounds by Phosphine–Cu-Catalyzed 1,3-Enyne Hydroboration. Insights Regarding Stereochemical Integrity of Cu–Allenyl Intermediates. _J. Am. Chem. Soc._ 140, 2643–2655
(2018). Article CAS PubMed PubMed Central Google Scholar * Sang, H. L., Yu, S. & Ge, S. Copper-catalyzed asymmetric hydroboration of 1,3-enynes with pinacolborane to access chiral
allenylboronates. _Org. Chem. Front._ 5, 1284–1287 (2018). Article CAS Google Scholar * Yang, C. et al. Catalytic Asymmetric Conjugate Protosilylation and Protoborylation of
2-Trifluoromethyl Enynes for Synthesis of Functionalized Allenes. _Org. Lett._ 22, 1360–1367 (2020). Article CAS PubMed Google Scholar * Miralles, N., Maza, R. J. & Fernández, E.
Synthesis and Reactivity of 1,1-Diborylalkanes towards C–C Bond Formation and Related Mechanisms. _Adv. Synth. Cat._ 360, 1306–1327 (2018). Article CAS Google Scholar * Nallagonda, R.,
Padala, K. & Masarwa, A. gem-Diborylalkanes: recent advances in their preparation, transformation and application. _Org. Biomol. Chem._ 16, 1050–1064 (2018). Article CAS PubMed Google
Scholar * Wu, C. & Wang, J. Geminal bis(boron) compounds: Their preparation and synthetic applications. _Tetrahedron Lett._ 59, 2128–2140 (2018). Article CAS Google Scholar * Corro,
M., Salvado, O., González, S., Dominguez-Molano, P. & Fernández, E. Reactivity Trends with Borylalkyl Copper(I) Species. _Eur. J. Inorg. Chem._ 2021, 2802–2813 (2021). Article CAS
Google Scholar * Lee, Y., Han, S. & Cho, S. H. Catalytic Chemo- and Enantioselective Transformations of gem-Diborylalkanes and (Diborylmethyl)metallic Species. _Acc. Chem. Res._ 54,
3917–3929 (2021). Article CAS PubMed Google Scholar * Zhang, C., Hu, W. & Morken, J. P. α-Boryl Organometallic Reagents in Catalytic Asymmetric Synthesis. _ACS Catal._ 11,
10660–10680 (2021). Article CAS PubMed PubMed Central Google Scholar * Paul, S., Das, K. K., Aich, D., Manna, S. & Panda, S. Recent developments in the asymmetric synthesis and
functionalization of symmetrical and unsymmetrical gem-diborylalkanes. _Org. Chem. Front._ 9, 838–852 (2022). Article CAS Google Scholar * Kim, K. D. & Lee, J. H. Development of
Transition-Metal-Free Carbon–Carbon and Carbon-Boron Bond-Forming Reactions by Utilizing 1,1-Bis[(Pinacolato)Boryl]Alkanes. _Bull. Kor. Chem. Soc._ 39, 5–7 (2018). Article ADS CAS Google
Scholar * Cuenca, A. B. & Fernández, E. Boron-Wittig olefination with gem-bis(boryl)alkanes. _Chem. Soc. Rev._ 50, 72–86 (2021). Article CAS PubMed Google Scholar * Jo, W., Lee, J.
H. & Cho, S. H. Advances in transition metal-free deborylative transformations of gem-diborylalkanes. _Chem. Commun._ 57, 4346–4353 (2021). Article CAS Google Scholar * Salvado, O.
& Fernández, E. A modular olefination reaction between aldehydes and diborylsilylmethide lithium salts. _Chem. Commun._ 57, 6300–6303 (2021). Article CAS Google Scholar * Abu Ali, H.,
Goldberg, I., Kaufmann, D., Burmeister, C. & Srebnik, M. Novel C1-Bridged Bisboronate Derivatives by Insertion of Diazoalkanes into Bis(pinacolato)diborane(4). _Organometallics_ 21,
1870–1876 (2002). Article Google Scholar * Cho, S. H. & Hartwig, J. F. Iridium-catalyzed diborylation of benzylic C–H bonds directed by a hydrosilyl group: synthesis of
1,1-benzyldiboronate esters. _Chem. Sci._ 5, 694–698 (2014). Article CAS Google Scholar * Wommack, A. J. & Kingsbury, J. S. On the scope of the Pt-catalyzed Srebnik diborylation of
diazoalkanes. An efficient approach to chiral tertiary boronic esters and alcohols via B-stabilized carbanions. _Tetrahedron Lett._ 55, 3163–3166 (2014). Article CAS Google Scholar *
Palmer, W. N., Obligacion, J. V., Pappas, I. & Chirik, P. J. Cobalt-Catalyzed Benzylic Borylation: Enabling Polyborylation and Functionalization of Remote, Unactivated C(sp3)–H Bonds.
_J. Am. Chem. Soc._ 138, 766–769 (2016). Article CAS PubMed Google Scholar * Palmer, W. N., Zarate, C. & Chirik, P. J. Benzyltriboronates: Building Blocks for Diastereoselective
Carbon–Carbon Bond Formation. _J. Am. Chem. Soc._ 139, 2589–2592 (2017). Article CAS PubMed PubMed Central Google Scholar * Lee, H., Lee, Y. & Cho, S. H. Palladium-Catalyzed
Chemoselective Negishi Cross-Coupling of Bis[(pinacolato)boryl]methylzinc Halides with Aryl (Pseudo)Halides. _Org. Lett._ 21, 5912–5916 (2019). Article CAS PubMed Google Scholar * Miura,
T., Nakahashi, J. & Murakami, M. Enantioselective Synthesis of (_E_)-δ-Boryl-Substituted anti-Homoallylic Alcohols Using Palladium and a Chiral Phosphoric Acid. _Angew. Chem., Int. Ed._
56, 6989–6993 (2017). Article CAS Google Scholar * Miura, T. et al. Enantioselective Synthesis of anti-1,2-Oxaborinan-3-enes from Aldehydes and 1,1-Di(boryl)alk-3-enes Using Ruthenium
and Chiral Phosphoric Acid Catalysts. _J. Am. Chem. Soc._ 139, 10903–10908 (2017). Article CAS PubMed Google Scholar * Park, J., Choi, S., Lee, Y. & Cho, S. H. Chemo- and
Stereoselective Crotylation of Aldehydes and Cyclic Aldimines with Allylic gem-Diboronate Ester. _Org. Lett._ 19, 4054–4057 (2017). Article CAS PubMed Google Scholar * Gao, S., Chen, J.
& Chen, M. (Z)-α-Boryl-crotylboron reagents via Z-selective alkene isomerization and application to stereoselective syntheses of (E)-α-boryl-syn-homoallylic alcohols. _Chem. Sci._ 10,
3637–3642 (2019). Article CAS PubMed PubMed Central Google Scholar * Gao, S., Duan, M., Shao, Q., Houk, K. N. & Chen, M. Development of α,α-Disubstituted Crotylboronate Reagents and
Stereoselective Crotylation via Brønsted or Lewis Acid Catalysis. _J. Am. Chem. Soc._ 142, 18355–18368 (2020). Article CAS PubMed Google Scholar * Green, J. C., Zanghi, J. M. &
Meek, S. J. Diastereo- and Enantioselective Synthesis of Homoallylic Amines Bearing Quaternary Carbon Centers. _J. Am. Chem. Soc._ 142, 1704–1709 (2020). Article CAS PubMed PubMed Central
Google Scholar * La Cascia, E., Cuenca, A. B. & Fernández, E. Opportune gem-Silylborylation of Carbonyl Compounds: A Modular and Stereocontrolled Entry to Tetrasubstituted Olefins.
_Chem. Eur. J._ 22, 18737–18741 (2016). Article PubMed Google Scholar * Lee, Y., Park, J. & Cho, S. H. Generation and Application of (Diborylmethyl)zinc(II) Species: Access to
Enantioenriched gem-Diborylalkanes by an Asymmetric Allylic Substitution. _Angew. Chem., Int. Ed._ 57, 12930–12934 (2018). Article CAS Google Scholar * Kim, J. & Cho, S. H. Access to
Enantioenriched Benzylic 1,1-Silylboronate Esters by Palladium-Catalyzed Enantiotopic-Group Selective Suzuki–Miyaura Coupling of (Diborylmethyl)silanes with Aryl Iodides. _ACS Catal._ 9,
230–235 (2019). Article CAS Google Scholar * Kim, J., Lee, E. & Cho, S. H. Chemoselective Palladium-Catalyzed Suzuki-Miyaura Cross-Coupling of (Diborylmethyl)silanes with Alkenyl
Bromides. _Asian J. Org. Chem._ 8, 1664–1667 (2019). Article CAS Google Scholar * Shin, M. et al. Facile Synthesis of α-Boryl-Substituted Allylboronate Esters Using Stable
Bis[(pinacolato)boryl]methylzinc Reagents. _Org. Lett._ 22, 2476–2480 (2020). Article CAS PubMed Google Scholar * Han, S., Lee, Y., Jung, Y. & Cho, S. H. Stereoselective Access to
Tetra- and Tri-Substituted Fluoro- and Chloro-Borylalkenes via Boron-Wittig Reaction. _Angew. Chem., Int. Ed._ 61, e202210532 (2022). Article CAS Google Scholar * Hwang, C., Lee, Y., Kim,
M., Seo, Y. & Cho, S. H. Diborylmethyl Group as a Transformable Building Block for the Diversification of Nitrogen-Containing Molecules. _Angew. Chem., Int. Ed._ 61, e202209079 (2022).
Article ADS CAS Google Scholar * Hu, J. et al. Photocatalyzed Borylcyclopropanation of Alkenes with a (Diborylmethyl)iodide Reagent. _Angew. Chem., Int. Ed._ 62, e202305175 (2023).
Article CAS Google Scholar * Fang, T., Wang, L., Wu, M., Qi, X. & Liu, C. Diborodichloromethane as Versatile Reagent for Chemodivergent Synthesis of gem-Diborylalkanes. _Angew. Chem.,
Int. Ed._ 62, e202315227 (2024). Google Scholar * Rona, P. & Crabbe, P. A novel allene synthesis. _J. Am. Chem. Soc._ 90, 4733–4734 (1968). Article CAS Google Scholar * Marshall, J.
A. & Pinney, K. G. Stereoselective synthesis of 2,5-dihydrofurans by sequential SN2’ cleavage of alkynyloxiranes and silver(I)-catalyzed cyclization of the allenylcarbinol products. _J.
Org. Chem._ 58, 7180–7184 (1993). Article CAS Google Scholar * Ohmiya, H., Yokobori, U., Makida, Y. & Sawamura, M. General Approach to Allenes through Copper-Catalyzed γ-Selective
and Stereospecific Coupling between Propargylic Phosphates and Alkylboranes. _Org. Lett._ 13, 6312–6315 (2011). Article CAS PubMed Google Scholar * Uehling, M. R., Marionni, S. T. &
Lalic, G. Asymmetric Synthesis of Trisubstituted Allenes: Copper-Catalyzed Alkylation and Arylation of Propargylic Phosphates. _Org. Lett._ 14, 362–365 (2012). Article CAS PubMed Google
Scholar * Skotnitzki, J. et al. Regio- and diastereoselective reactions of chiral secondary alkylcopper reagents with propargylic phosphates: preparation of chiral allenes. _Chem. Sci._ 11,
5328–5332 (2020). Article CAS PubMed PubMed Central Google Scholar * Harutyunyan, S. R., den Hartog, T., Geurts, K., Minnaard, A. J. & Feringa, B. L. Catalytic Asymmetric Conjugate
Addition and Allylic Alkylation with Grignard Reagents. _Chem. Rev._ 108, 2824–2852 (2008). Article CAS PubMed Google Scholar * Davies, R. P. The structures of lithium and magnesium
organocuprates and related species. _Coord. Chem. Rev._ 255, 1226–1251 (2011). Article CAS Google Scholar * Müller, D. S. & Marek, I. Copper mediated carbometalation reactions. _Chem.
Soc. Rev._ 45, 4552–4566 (2016). Article PubMed PubMed Central Google Scholar * Ziegler, D. S., Wei, B. & Knochel, P. Improving the Halogen–Magnesium Exchange by using New
Turbo-Grignard Reagents. _Chem. Eur. J._ 25, 2695–2703 (2019). Article CAS PubMed Google Scholar * Scaggs, W. R. & Snaddon, T. N. Enantioselective α-Allylation of Acyclic Esters
Using B(pin)-Substituted Electrophiles: Independent Regulation of Stereocontrol Elements through Cooperative Pd/Lewis Base Catalysis. _Chem. Eur. J._ 24, 14378–14381 (2018). Article CAS
PubMed Google Scholar * Luci, G., Mattioli, F., Falcone, M. & Di Paolo, A. Pharmacokinetics of Non-β-Lactam β-Lactamase Inhibitors. _Antibiotics_ 10, 769 (2021). Article CAS PubMed
PubMed Central Google Scholar * Das, B. C. et al. Boron-Containing heterocycles as promising pharmacological agents. _Bioorg. Med. Chem._ 63, 116748 (2022). Article CAS PubMed Google
Scholar * Gridnev, I., Kanai, G., Miyaura, N. & Suzuki, A. Synthesis of pinacol esters of 2,3-alkadienylboronic acid via the copper(I) mediated coupling reaction of Knochel’s
(dialkoxyboryl)methylzinc reagents with propargylic tosylates. _J. Organomet. Chem._ 481, C4–C7 (1994). Article CAS Google Scholar * Zheng, B. & Srebnik, M. Use of gem-Borazirconocene
Alkanes in the Regioselective Synthesis of.alpha.-Allenic Boronic Esters and Conversion of the Latter to Dienes and Trienes. _J. Org. Chem._ 60, 486–487 (1995). Article CAS Google Scholar
* Soundararajan, R., Li, G. & Brown, H. C. Chiral Synthesis via Organoboranes. 44. Racemic and Diastereo- and Enantioselective Homoallenylboration Using Dialkyl
2,3-Butadien-1-ylboronate Reagents. Another Novel Application of the Tandem Homologation−Allylboration Strategy. _J. Org. Chem._ 61, 100–104 (1996). Article CAS Google Scholar * Huang, Y.
et al. Asymmetric Synthesis of 1,3-Butadienyl-2-carbinols by the Homoallenylboration of Aldehydes with a Chiral Phosphoric Acid Catalyst. _Angew. Chem., Int. Ed._ 54, 7299–7302 (2015).
Article CAS Google Scholar * Ma, W.-W., Yang, C., Xie, Q. & Xu, Y.-H. Dienylation of N-benzoylhydrazones with CF3-substituted homoallenylboronates in water. _Org. Biomol. Chem._ 20,
1386–1390 (2022). Article CAS PubMed Google Scholar * Alonso, J. M. & Almendros, P. Highlighting the Rich Chemistry of the Allenone Moiety. _Adv. Synth. Cat._ 365, 1332–1384 (2023).
Article CAS Google Scholar * Alonso, J. M. & Almendros, P. Deciphering the Chameleonic Chemistry of Allenols: Breaking the Taboo of a Onetime Esoteric Functionality. _Chem. Rev._ 121,
4193–4252 (2021). Article CAS PubMed PubMed Central Google Scholar Download references ACKNOWLEDGEMENTS This work is dedicated to Professor Sang-gi Lee (Ewha Womans University) on his
honourable retirement. This work was supported by a National Research Foundation of Korea (NRF) grant funded by the Korea government (MSIT) [NRF-2022R1A2C3004731 and RS-2023-00219859]. This
research was also supported by the Bio&Medical Technology Development Program of the National Research Foundation (NRF) funded by the Korean government (MSIT) [RS-2023-00274113]. This
work was supported in part by the Glocal University 30 Project (Research Center for Molecular Catalysis, POSTECH). S.H. Cho thanks Korea Toray Science Foundation for the financial support.
We thank Vom Kang and Dr. Jinrok Oh (POSTECH) for assistance with X-ray structure analysis. AUTHOR INFORMATION AUTHORS AND AFFILIATIONS * Department of Chemistry, Pohang University of
Science and Technology (POSTECH), Pohang, 37673, Republic of Korea Yonghoon Jin, Junseok Lee, Woohyun Jo, Jeongwoo Yu & Seung Hwan Cho * Institute for Convergence Research and Education
in Advanced Technology (I-CREATE), Yonsei University, Seoul, 03722, Republic of Korea Seung Hwan Cho Authors * Yonghoon Jin View author publications You can also search for this author
inPubMed Google Scholar * Junseok Lee View author publications You can also search for this author inPubMed Google Scholar * Woohyun Jo View author publications You can also search for this
author inPubMed Google Scholar * Jeongwoo Yu View author publications You can also search for this author inPubMed Google Scholar * Seung Hwan Cho View author publications You can also
search for this author inPubMed Google Scholar CONTRIBUTIONS Y. J., W.J. and S.C. conceived and designed the project. Y.J. and S.C. wrote the manuscript. Y.J., J.L., W.J. and J.Y. carried
out the experiments. S.C. organized the research. All authors analysed the data, discussed the results and commented on the manuscript. CORRESPONDING AUTHOR Correspondence to Seung Hwan Cho.
ETHICS DECLARATIONS COMPETING INTERESTS The authors declare no competing interests. PEER REVIEW PEER REVIEW INFORMATION _Nature Communications_ thanks Elena Fernández, and the other,
anonymous, reviewers for their contribution to the peer review of this work. A peer review file is available. ADDITIONAL INFORMATION PUBLISHER’S NOTE Springer Nature remains neutral with
regard to jurisdictional claims in published maps and institutional affiliations. SUPPLEMENTARY INFORMATION SUPPLEMENTARY INFORMATION TRANSPARENT PEER REVIEW FILE RIGHTS AND PERMISSIONS OPEN
ACCESS This article is licensed under a Creative Commons Attribution-NonCommercial-NoDerivatives 4.0 International License, which permits any non-commercial use, sharing, distribution and
reproduction in any medium or format, as long as you give appropriate credit to the original author(s) and the source, provide a link to the Creative Commons licence, and indicate if you
modified the licensed material. You do not have permission under this licence to share adapted material derived from this article or parts of it. The images or other third party material in
this article are included in the article’s Creative Commons licence, unless indicated otherwise in a credit line to the material. If material is not included in the article’s Creative
Commons licence and your intended use is not permitted by statutory regulation or exceeds the permitted use, you will need to obtain permission directly from the copyright holder. To view a
copy of this licence, visit http://creativecommons.org/licenses/by-nc-nd/4.0/. Reprints and permissions ABOUT THIS ARTICLE CITE THIS ARTICLE Jin, Y., Lee, J., Jo, W. _et al._ Axially chiral
α-boryl-homoallenyl boronic esters as versatile toolbox for accessing centrally and axially chiral molecules. _Nat Commun_ 15, 9239 (2024). https://doi.org/10.1038/s41467-024-53606-6
Download citation * Received: 05 June 2024 * Accepted: 14 October 2024 * Published: 25 October 2024 * DOI: https://doi.org/10.1038/s41467-024-53606-6 SHARE THIS ARTICLE Anyone you share the
following link with will be able to read this content: Get shareable link Sorry, a shareable link is not currently available for this article. Copy to clipboard Provided by the Springer
Nature SharedIt content-sharing initiative