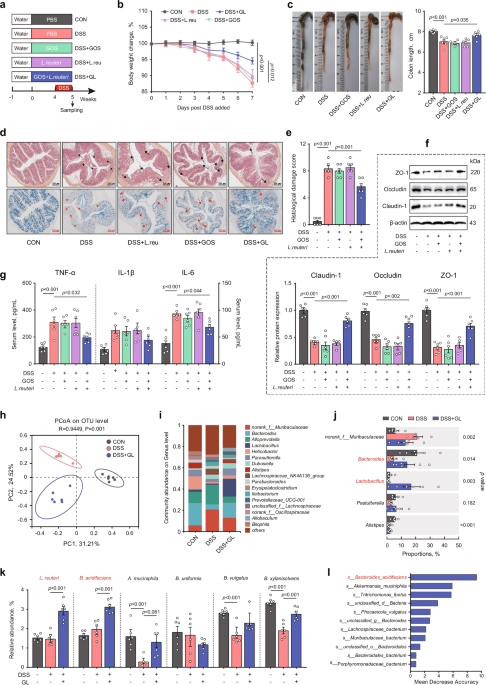
- Select a language for the TTS:
- UK English Female
- UK English Male
- US English Female
- US English Male
- Australian Female
- Australian Male
- Language selected: (auto detect) - EN
Play all audios:
ABSTRACT Ulcerative colitis (UC) is a debilitating inflammatory bowel disease characterized by intestinal inflammation, barrier dysfunction, and dysbiosis, with limited treatment options
available. This study systematically investigates the therapeutic potential of a synbiotic composed of galactooligosaccharides (GOS) and _Limosilactobacillus reuteri_ in a murine model of
colitis, revealing that GOS and _L. reuteri_ synergistically protect against intestinal inflammation and barrier dysfunction by promoting the synthesis of pentadecanoic acid, an odd-chain
fatty acid, from _Bacteroides acidifaciens_. Notably, the synbiotic, _B. acidifaciens_, and pentadecanoic acid are each capable of suppressing intestinal inflammation and enhancing tight
junction by inhibiting NF-κB activation. Furthermore, similar reduction in _B. acidifaciens_ and pentadecanoic acid levels are also observed in the feces from both human UC patients and
lipopolysaccharide-induced intestinal inflammation in pigs. Our findings elucidate the protective mechanism of the synbiotic and highlight its therapeutic potential, along with _B.
acidifaciens_ and pentadecanoic acid, for UC and other intestinal inflammatory disorders. SIMILAR CONTENT BEING VIEWED BY OTHERS NOVEL ANTI-INFLAMMATORY PROPERTIES OF MANNOSE
OLIGOSACCHARIDES IN THE TREATMENT OF INFLAMMATORY BOWEL DISEASE VIA LGALS3 MODULATION Article Open access 08 February 2025 STRONGER GUT MICROBIOME MODULATORY EFFECTS BY POSTBIOTICS THAN
PROBIOTICS IN A MOUSE COLITIS MODEL Article Open access 15 November 2022 _AKKERMANSIA MUCINIPHILA_ ONE EFFECTIVELY AMELIORATES DEXTRAN SULFATE SODIUM (DSS)-INDUCED ULCERATIVE COLITIS IN MICE
Article Open access 19 November 2024 INTRODUCTION Ulcerative colitis (UC) is an inflammatory bowel disease (IBD), but its etiology and pathogenesis remain enigmatic1. Emerging evidence
emphasizes its strong association with intestinal inflammation, barrier dysfunction, and dysbiosis2. However, few preventive and therapeutic options are currently available for UC. Dietary
intervention stands as a primary strategy for alleviating UC symptoms, with prebiotics, probiotics, and synbiotics showing promise due to their reported ability to modify intestinal
microbiota, modulate immune response, and enhance barrier function3,4,5. A comprehensive understanding of the underlying protective mechanisms of these interventions may provide better
options for treating UC. Galactooligosaccharides (GOS) and _Limosilactobacillus reuteri_ are well-known prebiotic and probiotic, respectively, with demonstrated benefits in microbial balance
and intestinal barrier function6,7. We previously showed that dietary GOS enhances intestinal barrier function in _Salmonella_-challenged mice by selectively enriching three lactobacilli,
including _L. reuteri_ 8. Notably, _L. reuteri_ can utilize GOS as a carbon resource, providing the foundation for synbiotic development9. However, the synergistic mechanisms between GOS and
_L. reuteri_ in alleviating inflammation and enhancing barrier function remain unexplored. It is important to identify the intestinal bacteria and metabolites regulated by the synbiotic.
This study presents compelling evidence that the combination of GOS and _L. reuteri_ mitigates intestinal inflammation and barrier dysfunction by enriching _Bacteroides acidifaciens_ and
promoting the synthesis of pentadecanoic acid (C15:0), an odd-chain fatty acid. In a mouse model of dextran sulfate sodium (DSS)-induced colitis, we observed a significant reduction in C15:0
level, which could be restored by the synbiotic-enriched _B. acidifaciens_. Importantly, similar impairments in _B. acidifaciens_ and C15:0 synthesis were also observed in other intestinal
inflammation conditions, such as UC and a porcine model of LPS-induced inflammation. Administration of _B. acidifaciens_ or C15:0 conferred significant protection against DSS-induced colitis
by suppressing inflammatory cytokine production while enhancing tight junction protein expression. Collectively, our findings uncover a major mechanism of action of GOS and _L. reuteri_ and
also offer a promising avenue for mitigating intestinal inflammation and barrier dysfunction in UC and potentially other intestinal inflammatory diseases. RESULTS SYNBIOTIC GOS AND _L.
REUTERI_ ARE HIGHLY EFFECTIVE IN AMELIORATING INTESTINAL INFLAMMATION AND BARRIER DYSFUNCTION WHILE ENRICHING _BACTEROIDES ACIDIFACIENS_ _L. reuteri_ can utilize GOS if provided as the only
carbon resource, implying a synergistic action between _L. reuteri_ and GOS9. To study the impact of a synbiotic consisting of GOS and _L. reuteri_ on intestinal inflammation and barrier
function, we administered GOS and _L. reuteri_ separately or in combination to mice for four weeks, followed by DSS treatment for another week (Fig. 1a). The results indicated that the
synbiotic, but not GOS or _L. reuteri_ alone, markedly reversed body weight loss (Fig. 1b) and shortening of the colon (Fig. 1c), while preserving the integrity of the colon mucosa (Fig.
1d). Furthermore, the synbiotic significantly alleviated intestinal damage (Fig. 1e), reversed DSS-induced suppression of tight junction proteins Claudin-1, Occludin, and ZO-1 (Fig. 1f), and
attenuated intestinal inflammation by decreasing pro-inflammatory cytokines TNF-α, IL-1β, and IL-6 (Fig. 1g). While DSS caused an obvious shift in the colonic microbiota composition, the
synbiotic led to further alterations without restoring it back to normal (Fig. 1h). A closer examination revealed DSS caused a drastic reduction of _Bacteroides_ in the colon of healthy
mice, while the synbiotic restored _Bacteroides_ in DSS-treated mice (Fig. 1i, j). Additionally, _Bacteroides acidifaciens_ and _B. xylanisolvens_ were specifically enriched among
_Bacteroides_ species (Fig. S1a). In contrast, GOS or _L. reuteri_ alone failed to enrich _B. acidifaciens_ in DSS-treated mice (Fig. 1k). Shotgun metagenomic sequencing further validated
the increase in relative abundance of _B. acidifaciens_ following synbiotic intervention (Fig. S1b–f). Moreover, random forest analysis revealed _B. acidifaciens_ to be ranked the most
important species that distinguished the intestinal microbiotas with and without synbiotic intervention (Fig. 1l). To further evaluate whether other strains of _L. reuteri_ exhibit similar
anti-inflammatory and _B. acidifaciens_-enriching effects, we administered two other strains of _L. reuteri_ isolated from the mouse feces by our lab in DSS-treated mice (Fig. S2a). One
strain, _L. reuteri_ #1, demonstrated beneficial effects comparable to _L. reuteri_ BNCC 186135, used above in the synbiotic, on DSS-induced body weight loss (Fig. S2b), intestinal
inflammation (Fig. S2c–e), barrier function (Fig. S2f), and serum cytokine levels (Fig. S2g). In contrast, the other strain had minimal impact. Additionally, _B. acidifaciens_ was
significantly enriched in DSS-treated mice supplemented with _L. reuteri_ #1, but not with strain #2 (Fig. S2h), suggesting a strain-specific effect. Therefore, it is possible to identify
more potent strains to be used in the synbiotic for treating UC if screening additional _L. reuteri_ strains. To further identify the key metabolites produced specifically by the synbiotic
to be responsible for _B. acidifaciens_ enrichment, we examined differentially abundant metabolites in the feces of DSS-treated mice administered with GOS, _L. reuteri_, or the synbiotic.
Our results revealed that β-alanine, N-acetyl-D-glucosamine, 4-methylhexanoic acid, C15:0, and myristic acid were the top five metabolites significantly enriched in response to the synbiotic
but not to GOS or _L. reuteri_ alone (Fig. S3a, b). To determine which metabolites preferentially support the growth of _B. acidifaciens_, we cultured mouse cecal microbiota anaerobically
with GOS, _L. reuteri_, the symbiotic, or each of the differentially enriched metabolites. The results showed that only N-acetyl-D-glucosamine significantly increased the relative abundance
of _B. acidifaciens_ in the culture medium, approaching the efficacy of the synbiotic (Fig. S3c). Additionally, N-acetyl-D-glucosamine specifically enhanced the growth of _B. acidifaciens_
when glucose was replaced (Fig. S3d). Taken together, N-acetyl-D-glucosamine is the major metabolite derived from the synbiotic GOS and _L. reuteri_ responsible for the enrichment of _B.
acidifaciens_. _B. ACIDIFACIENS_ IS ENRICHED IN RECIPIENT MICE BY FMT WITH SYNBIOTIC-DERIVED MICROBIOTA To assess whether the fecal microbiota from the synbiotic-supplemented mice is capable
of alleviating intestinal inflammation and barrier dysfunction, FMT was performed with intestinal microbiota-depleted recipient mice (Fig. 2a). Synbiotic-derived microbiota, rather than the
control microbiota, effectively attenuated the body weight loss (Fig. 2b), the colon length (Fig. 2c), and the damage to the intestinal mucosal surface (Fig. 2d, e) of DSS-treated mice.
Furthermore, the serum levels of pro-inflammatory cytokines, including TNF-α, IL-1β, and IL-6, were significantly suppressed in DSS-treated mice in response to the synbiotic (Fig. 2f), and
the expressions of tight junction proteins, such as Claudin-1 and Occludin, were substantially restored (Fig. 2g). Additionally, FMT altered the fecal microbiota structure of recipient mice
(Fig. 2h). At the genus level, _Bacteroides_ and _Lactobacillus_ were both increased in mice receiving the synbiotic-derived microbiota (Fig. 2i, j). At the species level, _B. acidifaciens_,
rather than _B. xylanisolvens_, was successfully colonized the intestine following FMT (Fig. 2k), suggesting that _B. acidifaciens_ may be potentially important in protecting against
intestinal inflammation and barrier dysfunction in DSS-treated mice. _B. ACIDIFACIENS_ PROTECTS AGAINST INTESTINAL INFLAMMATION AND BARRIER DYSFUNCTION To directly assess the role of _B.
acidifaciens_ in protecting against intestinal inflammation and barrier dysfunction, _B. acidifaciens_ was inoculated into mice for two weeks, followed by one week of DSS treatment to induce
colitis (Fig. 3a). _B. acidifaciens_ significantly attenuated DSS-induced intestinal damage as shown in body weight loss, the colon length, intestinal morphology, and histological damage
score (Fig. 3b–e). Oral administration of _B. acidifaciens_ also partially restored the protein expressions of tight junctions (Fig. 3f) and suppressed protein expressions of
pro-inflammatory cytokines (Fig. 3g). These results confirmed that _B. acidifaciens_ enriched by GOS and _L. reuteri_ protects against intestinal inflammation and barrier dysfunction. A
valuation of a second strain of _B. acidifaciens_ in DSS-treated mice revealed similar protective effects on reversing body weight loss, intestinal inflammation, barrier dysfunction, and
serum cytokine increase (Fig. S4a–h). Additional _B. acidifaciens_ strains may be tested to determine a strain-specific effect and the possibility of identifying more effective probiotic
candidates. SYNBIOTIC GOS AND _L. REUTERI_ ENHANCE THE SYNTHESIS OF PENTADECANOIC ACID (C15:0) TO ALLEVIATE INTESTINAL INFLAMMATION AND BARRIER DYSFUNCTION To explore the protective role of
the synbiotic-derived metabolites in the restoration of intestinal barrier dysfunction, bacterial cell-free supernatant was prepared from the feces of mice fed standard chow or supplemented
with the synbiotic, followed by oral administration to DSS-treated mice (Fig. 4a). The results showed that the supernatant derived from synbiotic-supplemented mice, but not standard chow-fed
mice, significantly reversed the body weight loss of DSS-treated mice (Fig. 4b). The colon length (Fig. 4c), mucosal integrity (Fig. 4d), histological damage score (Fig. 4e), goblet cell
number (Fig. 4f), tight junction protein expressions (Fig. 4g), and serum pro-inflammatory cytokine levels (Fig. 4h) were also restored by the synbiotic-derived intestinal supernatant,
suggesting that the intestinal metabolites produced in response to synbiotic administration contribute to alleviating intestinal inflammation and barrier dysfunction. To identify the
possible metabolites responsible for disease alleviation, targeted metabolomics was conducted with the fecal bacterial cell-free supernatants of DSS-treated mice supplemented with or without
GOS, _L. reuteri_, or their combination. A total of 35 metabolites from 306 representative metabolites of different classes were found to be differentially enriched in response to GOS
and/or _L. reuteri_ (Fig. 5a). Among them, pentadecanoic acid (C15:0) was the most significantly down-regulated following DSS treatment and up-regulated in response to the synbiotic
administration as evidenced by the lowest adjusted p-value (Fig. 5b, c). Indeed, C15:0 was increased in the feces of DSS-treated mice following synbiotic administration (Fig. 5d).
Interestingly, 9-pentadecanoic acid, a less abundant C15:0 isoform, was also similarly increased in response to the synbiotic (Fig. 5e). Consistently, fecal concentrations of C15:0 were also
increased in DSS-treated mice transplanted with fecal microbiota (Fig. 5f), fecal bacterial cell-free supernatant (Fig. 5g), or _B. acidifaciens_ (Fig. 5h). These results collectively
suggested that C15:0 may be a key metabolite produced by the synbiotic and responsible for alleviating intestinal inflammation and barrier dysfunction. _B. ACIDIFACIENS_ SYNTHESIZES
PENTADECANOIC ACID (C15:0) TO PROTECT AGAINST THE INTESTINAL INFLAMMATION AND BARRIER DYSFUNCTION To identify whether C15:0 can be directly synthesized by _B. acidifaciens_, we cultured _B.
acidifaciens_ in the presence of GOS and _L. reuteri_ individually or in combination. Elevated C15:0 was observed in the culture supernatant of _B. acidifaciens_, and importantly, the
combination of GOS and _L. reuteri_ markedly increased the amount of C15:0 when co-cultured with _B. acidifaciens_, while either alone only had a marginal effect (Fig. 6a). To directly
confirm whether _B. acidifaciens_ has the capacity to synthesize C15:0, we performed whole-genome sequencing of _B. acidifaciens_ and found it to encode 39 genes involved in lipid metabolism
(Fig. S5). Notably, all key genes for biosynthesis of C15:0 were present (Fig. 6b), suggesting that _B. acidifaciens_ is a C15:0-producing bacterium. To further examine how those major
C15:0-synthesizing genes are regulated in the synbiotic-supplemented mice, we performed shotgun metagenomics sequencing and revealed that four genes, namely _fabK, acrC, fabF_ and _FATA_,
were downregulated by DSS, but restored upon the synbiotic supplementation (Fig. 6c), suggesting that the combination of GOS and _L. reuteri_ may accelerate C15:0 biosynthesis through
enriching _B. acidifaciens_. Indeed, among all differentially enriched fatty acids, only C15:0 showed a significantly positive correlation with _B. acidifaciens_ in DSS-treated mice
supplemented with the synbiotic (Fig. 6d). Additionally, the fecal concentrations of C15:0 were positively correlated with _B. acidifaciens_ in recipient mice administered with the fecal
microbiota of the synbiotic-supplemented mice (Fig. 6e) or direct _B. acidifaciens_ (Fig. 6f). These results collectively suggested that C15:0 is a metabolite synthesized by _B.
acidifaciens_ that may be involved in the protection of mice from DSS-induced intestinal damage. PENTADECANOIC ACID (C15:0) AMELIORATES INTESTINAL INFLAMMATION AND BARRIER DYSFUNCTION BY
ACTIVATING FATP4 AND SUPPRESSING NF-ΚB ACTIVATION To directly verify the protection of _B. acidifaciens_-derived C15:0 against the intestinal barrier dysfunction, C15:0 was orally
administered daily to mice for three weeks, followed by DSS treatment for another week (Fig. 7a). The results showed that C15:0 significantly attenuated the body weight loss (Fig. 7b) and
damage to the colon (Fig. 7c–f), upregulated tight junction protein expressions (Fig. 7g) and suppressed mRNA expressions of pro-inflammatory cytokines (Fig. 7h). Furthermore, C15:0 restored
tight junction protein expressions and significantly downregulated LPS-induced pro-inflammatory cytokine expressions in both murine MODE-K cells (Fig. 7i–k) and human Caco-2 cells (Fig.
S6), suggesting that C15:0 is broadly anti-inflammatory and protects barrier function by suppressing inflammatory cytokine synthesis. To further uncover the underlying mechanisms of
C15:0-mediated protection of the intestinal barrier integrity, we performed RNA-seq of the colon tissue of DSS-treated mice with or without C15:0 administration (Fig. 8a). The results showed
that C15:0 inhibited multiple inflammation-related pathways (Fig. 8b). RT-qPCR further confirmed C15:0-mediated suppression of the mRNA expression of pro-inflammatory cytokines _IL-1α_,
_CCL2_, _NLRP3_, _CXCL1_ and _IL-18_ in the colon of DSS-treated mice (Fig. S7a–d). Mechanically, C15:0 suppressed NF-κB activation in DSS-treated mice (Fig. 8c) and LPS-challenged MODE-K
cells (Fig. 8d), as evidenced by reduced phosphorylation of NF-κB p65. Consistently, phosphorylation of NF-κB p65 were also decreased in DSS-treated mice administered with the synbiotic
(Fig. 8e), fecal microbiota (Fig. 8f), fecal bacterial cell-free supernatant (Fig. 8g), or _B. acidifaciens_ (Fig. 8h). Additionally, fatty acid transport protein 4 (FATP4/Slc27a4), a major
long-chain fatty acid transporter and fatty acyl-CoA synthase10,11, was suppressed in the colon of DSS-treated mice (Fig. S8a), LPS-challenged human Caco-2 cells (Fig. S8b), and murine
MODE-K cells (Fig. S8c), but were largely restored by C15:0 administration. Furthermore, C15:0-mediated restoration of tight junction protein expression and suppression of pro-inflammatory
cytokines in LPS-treated MODE-K cells were markedly reversed by an FATP4 inhibitor (Fig. S8d–f). These results suggested that the anti-inflammatory and barrier protective properties of C15:0
were mediated through FATP4 and suppression of NF-κB activation. _B. ACIDIFACIENS_ AND PENTADECANOIC ACID ARE DOWNREGULATED IN UC PATIENTS AND LPS-CHALLENGED PIGLETS We observed a
significant decrease in _B. acidifaciens_ and C15:0 levels in DSS-treated mice, which was restored by GOS and _L. reuteri_ administration. To confirm the clinical relevance of our findings
in other intestinal inflammatory diseases, we initially examined the relative abundance of _B. acidifaciens_ in a cohort of 15 healthy donors and 11 UC patients admitted to Jiangyin People’s
Hospital affiliated with Nantong University (Jiangsu, China) in 2023. RT-qPCR revealed a significant reduction of _B. acidifaciens_ in the feces of UC patients compared to healthy
individuals (Fig. 9a). The concentration of C15:0 was also significantly reduced in UC patients (Fig. 9b). Additionally, fecal concentrations of C15:0 positively correlated with the
abundance of _B. acidifaciens_ in the feces of both UC and heathy individuals (Fig. 9c). Similarly, LPS challenge induced obvious gut inflammation in piglets (Fig. S9), associated with
significant reductions of both _B. acidifaciens_ (Fig. 9d) and C15:0 levels (Fig. 9e) in the feces. A strong positive association was also observed between _B. acidifaciens_ and C15:0 in
LPS-challenged pigs (Fig. 9f). To further validate our results, we retrieved and analyzed the C15:0 concentrations from several publicly available metabolomic datasets involving a total of
503 healthy controls and 649 UC patients from China, USA, Netherlands, and Norway in several recent studies12,13,14,15,16. Consistently, the C15:0 concentrations in the feces (Fig. 9g),
serum (Fig. 9h), and colonic biopsy (Fig. 9i) of UC patients were significantly lower than those of healthy volunteers. Furthermore, compared to inactive UC patients, active UC patients
showed significantly reduced C15:0 concentrations in the feces (Fig. 9j), serum (Fig. 9k), and colonic biopsy (Fig. 9l). These results collectively suggested that inflammation and barrier
dysfunction correlate with diminished levels of _B. acidifaciens_ and C15:0 across different intestinal inflammatory disease models in various animal species. Strategies such as combining
GOS and _L. reuteri_ may hold therapeutic potential for UC. Additionally, administration of _B. acidifaciens_ or C15:0 may also be promising for treating UC and other intestinal inflammatory
disorders. DISCUSSION UC has emerged as a significant public health concern with a rising incidence1. Various factors, such as dysregulated immune response, dysbiosis, intestinal barrier
dysfunction, have been implicated in UC pathogenesis17,18. Restoring homeostasis of the intestinal microbiota, inflammation, and barrier function is considered a primary therapeutic
approach19,20. In this study, we demonstrated the protective effects and potential mechanisms of a synbiotic consisting of GOS and _L. reuteri_ on intestinal inflammation and barrier
dysfunction. We showed that this synbiotic improves intestinal health by enriching _B. acidifaciens_, which subsequently leads to increased synthesis of a key metabolite, C15:0. Intestinal
health is improved due to the anti-inflammatory and barrier protective properties of _B. acidifaciens_ and C15:0. These findings suggest that the synbiotic GOS and _L. reuteri_, along with
_B. acidifaciens_ and C15:0, holds promise as a dietary supplement for preventing UC development. We further revealed UC patients, DSS-treated mice, and LPS-challenged pigs all exhibit a
deficiency in _B. acidifaciens_ and C15:0, underscoring the critical role of _B. acidifaciens_ in UC development. _B. acidifaciens_, a dominant commensal bacterium in the intestine, has been
reported to protect against liver injury and metabolic disorders21,22. Consistently, _B. acidifaciens_ has been shown to elevate intestinal immunoglobulin A (IgA) levels and protect against
pathogenic infections in IBD23. Alginate oligosaccharides were reported to improve immune function and dysbiosis by enriching _B. acidifaciens_ in DSS-induced colitis24. Furthermore,
extracellular vesicles of _B. acidifaciens_ are capable of restoring mucosal barrier function and microbiota balance in DSS-induced colitis25. These results confirmed the beneficial roles of
_B. acidifaciens_ in intestinal health, especially in UC. Fatty acids play pivotal roles in the nutrition and protection of enterocytes while acting as mediators in transcriptional and
inflammatory processes26,27. Previous studies have revealed alterations in the metabolism of long-chain fatty acids in the intestine of UC patients12,28,29. Consistently, our study found a
significant reduction in C15:0, an odd-chain fatty acid, in UC patients, as well as in DSS-treated mice and LPS-challenged piglets. Studies with C15:0 have highlighted its anti-inflammatory,
anti-fibrotic, and anticancer activities through various mechanisms, such as AMPK and PPAR-α/δ activation, and inhibition of mTOR, JAK-STAT, and HDAC6 in vitro and in vivo30,31,32,33,34,35.
We also revealed that C15:0 inhibits inflammatory response by suppressing the phosphorylation of NF-κB, which plays a central role in the homeostasis and activation of the immune
system36,37,38. Indeed, epidemiological studies have linked higher circulating C15:0 concentrations to lower cholesterol, triglycerides, liver enzymes, C-reactive protein, adipokines, body
mass index, and improved insulin sensitivity and β cell function in humans39,40,41,42,43,44,45. Furthermore, C15:0 exhibits antimicrobial properties, inhibiting the growth of pathogenic
bacteria and fungi46,47. Due to its role in the alleviation metabolic disorders and chronic inflammation, C15:0 has been proposed as a potential essential fatty acid31,39,44,48,49,50.
However, the mechanism by which C15:0 suppresses inflammation and NF-κB activation remains largely unknown. FATP4 is a major transporter and fatty acid CoA synthase mediating uptake and
downstream biological activities of long-chain fatty acids10,11. Our study indicated that FATP4 was upregulated in DSS-treated mice following C15:0 supplementation. Binding and transporting
of C15:0 to FATP4 ultimately led to the dephosphorylation of NF-κB p65, which reduced inflammation and improved barrier integrity. These findings align with our observation that
pharmacological blockage of FATP4 with a specific inhibitor reverses the anti-inflammatory and barrier protective properties of C15:0. C15:0 was reported to be synthesized by microorganisms,
such as _Yarrowia lipolytica_, _Rhodococcus_, and _Saccharomyces cerevisiae_51,52,53,54. A recent study showed that _Parabacteriodes distasonis_ also synthesize C15:0 from inulin to
ameliorate nonalcoholic steatohepatitis (NASH)55. In this study, we confirmed that _B. acidifaciens_ produces C15:0 in response to GOS and _L. reuteri_ both in vitro and in vivo. For C15:0
synthesis, most current work focuses on the supplement of exogenous three-carbon compounds, like propionyl-CoA and malonyl-acyl carrier protein (ACP), as substrates56. In addition, the
essential FAS enzymes for C15:0 synthesis were all encoded in the genome of _B. acidifaciens_, including β‐ketoacyl‐ACP synthase II (fabF), β‐ketoacyl‐ACP reductase (fabG), β‐hydroxyacyl‐ACP
dehydratase (fabA), enoyl‐ACP reductase (fabK), malonyl-CoA:ACP transacylase (fabD) and fatty acyl-CoA thioesterase (paaL/FATA)51. Thus, _B. acidifaciens_ may be genetically enhanced for
industrial production of C15:0, similar to a recent metabolic engineering attempt with _E. coli_ 57. It is noted that anti-tumor necrosis factor (TNF) agents, thiopurines, and
glucocorticoids are standard treatments for UC patients, reducing the disease activity index (DAI) by approximately 50%58,59,60. In our study, supplementation with the synbiotic (GOS and _L.
reuteri_), _B. acidifaciens_, or C15:0 significantly reduced colonic damage by 25–30%, as indicated by a lower histological damage score in DSS-induced colitis. Although the effect size of
our nutritional strategies appears smaller than current UC treatments, further optimizations, such as microencapsulation, dosage adjustments, and screening for more effective bacterial
strains, will undoubtedly enhance their efficacy against UC. Collectively, our findings demonstrate that a synbiotic combination of GOS and _L. reuteri_ protects against intestinal
inflammation and barrier dysfunction by promoting the synthesis of pentadecanoic acid (C15:0) from _B. acidifaciens_. This critical link between synbiotics, commensal bacteria, and bacterial
metabolites provides evidence and potential therapeutic avenues for UC and other intestinal inflammatory disorders. METHODS ETHICAL STATEMENT The clinical cohort was approved by the Ethics
Committee of Jiangyin People’s Hospital Affiliated to Nantong University (Jiangsu, China) and followed the Declaration of Helsinki under the protocol number of 2023–043. All animal
experiments were approved by the Animal Care and Use Committee of China Agricultural University (Beijing, China) under the protocol number of Aw60213202-1-1. HUMAN CLINICAL PATIENT DATA AND
SAMPLE COLLECTION Included in this study were 15 healthy donors (4 females and 11 males) and 11 UC patients (5 females and 6 males) aged from 25 to 70, which were patients admitted to
Jiangyin People’s Hospital affiliated with Nantong University (Jiangsu, China) in 2023. The characteristics of the study participants were detailed in Supplementary Table S1. UC patients
were diagnosed based on clinical, endoscopic, and surgical specimens. Healthy donors and acute UC patients were free from antibiotics for more than two weeks prior to fecal collection.
Informed consent was obtained from all participants. The samples were stored at –80°C for further analysis. Additionally, to further validate our results, the C15:0 concentrations were
retrieved and reanalyzed from publicly available metabolomic datasets involving a total of 503 healthy controls and 649 UC patients from China, USA, Netherlands, and Norway12,13,14,15,16.
Detailed patient information was provided in Supplementary Table S2. ANIMAL EXPERIMENTS In the mouse model of DSS-induced colitis, 6- to 8-week-old male C57BL/6 J mice were housed in a
specific pathogen-free facility under ambient temperature of 20–22°C, humidity of 40-60%, and 12-h light/12-h dark cycle with free access to water and standard chow. The composition of the
chow diet including: cornstarch (46.57%), maltodextrin (15.50%), casein (14.00%), sucrose (10.00%), cellulose fibers (5.00%), soy oil (4.00%), mineral mix (3.50%), vitamin mix (1.00%),
L-cysteine (0.18%), and choline (0.25%). To induce colitis, DSS (Coolaber, Beijing, China) was added to drinking water to the final concentration of 3% for a week. To administer GOS, _L.
reuteri_ or the synbiotic, GOS (5% w/w; Coolaber) was supplemented to the diet for four weeks with or without daily oral gavage of 2 × 108 CFU _L. reuteri_ (BNCC 186135, BeNa Culture
Collection, China). GOS (5% w/w; Coolaber) was also supplemented to the diet of DSS-treated mice for three weeks with or without daily oral gavage of 2 × 108 CFU of two other _L. reuteri_
strains (#1 and #2, isolated from mouse feces in our lab). For fecal microbiota transplantation (FMT), a cocktail of antibiotics (1 g/L streptomycin, 0.5 g/L ampicillin, 0.5 g/L vancomycin,
and 1 g/L gentamicin) were added to drinking water for 4 weeks to deplete the intestinal microbiota. Mice were then orally administered with 0.2 mL PBS or fecal suspension daily for two
weeks. The fecal suspension was obtained from the mice fed standard chow or administered with GOS and _L. reuteri_, followed by suspension of 100 mg feces in 1.5 mL sterile anaerobic PBS.
Oral administration of _B. acidifaciens_ (BNCC 353574, BeNa Culture Collection, China) or _B. acidifaciens_ JCM10556 (DSMZ, Germany) was performed by gavaging 2 × 108 CFU of the bacteria in
0.2 mL PBS daily for three weeks. For fecal supernatant transplantation (FST), mice were orally administered with 0.2 mL PBS or fecal bacterial cell-free supernatant daily for two weeks. The
bacterial cell-free supernatant was obtained from the feces of mice fed standard chow or administered with GOS and _L. reuteri_, followed by centrifugation at 8000 × _g_ for 5 min and
filtration through a 0.22 μm filter. Oral administration of pentadecanoic acid (C15:0, 35 mg/kg)31 was performed by gavaging in 0.2 mL PBS daily for three weeks. For sampling, a colonic
segment was collected from each mouse and fixed in 4% paraformaldehyde for H&E staining and/or Alcian blue (AB) staining. Histological scoring was performed with each segment on Zeiss
Axio Imager microscope based on the extent of epithelial surface loss, crypt destruction, and inflammatory cell infiltration as previously described61. The acidic goblet cells were counted
with Image J software62. Feces and the remaining colonic segments were collected and stored at –80 °C for further analysis. In the pig model of LPS-induced intestinal inflammation, twelve
24-day-old male weaned Duroc-Landrace-Yorkshire piglets were housed in an environmentally controlled room at 22–25 °C with free access to feed and water. The composition of diet for weaned
piglets including: corn (58.60%), soybean meal (13.00%), whey powder (8.00%), fish meal (3.00%), soy protein concentrate (5.00%), extruded full-fat soybean (5.00%), glucose (2.00%), soybean
oil (2.00%), CaHPO4 (1.00%), limestone (0.80%), NaCl (0.20%), L-lysine-HCl (0.55%), DL-methionine (0.10%), L-threonine (0.20%), L-tryptophan (0.05%), mineral and vitamin premix (0.50%).
Piglets were administered intraperitoneally with 3 mL sterile saline in the presence or absence of LPS (100 μg/kg BW; Sigma-Aldrich) 6 h prior to samples collection. DNA EXTRACTION AND 16S
RRNA GENE SEQUENCING AND ANALYSIS Total genomic DNA of fecal samples were extracted for the amplification using universal primers 338 F/806 R targeting the V3-V4 region of the bacterial 16S
rRNA gene and sequenced on Illumina MiSeq platform. Data analysis was performed on the free online platform of Majorbio Cloud Platform (www.majorbio.com). In brief, raw paired-end reads were
strictly analyzed using QIIME 263. Taxonomic assignment was achieved using RDP classifier and annotated with the SILVA138 reference database. Principal coordinates analysis (PCoA) was based
on unweighted Unifrac distances. Differentially enriched bacteria taxa were based on Kruskal-Wallis test and post-hoc Dunn’s test. Spearman rank correlation coefficient and linear
regression were used for correlation analysis. SHOTGUN METAGENOMIC SEQUENCING AND ANALYSIS Total genomic DNA was used for sequencing and library construction on Illumina NovaSeq platform.
Data analysis was performed on the free online platform of Majorbio Cloud Platform (www.majorbio.com). In brief, raw reads were filtered using Fastp and assembled using MEGAHIT, and the
assembled contig was predicted using MetaGene64,65. After a non-redundant gene catalog was constructed using CD-HIT, gene abundance with 95% identity was calculated using SOAPaligner66,67.
Representative sequences were aligned to NR database for taxonomic annotations using Diamond68. Differential analysis was achieved using Kruskal-Wallis test and post-hoc Dunn’s test. The top
20 species were selected as variables in the random forest model. QUANTIFICATION OF SPECIFIC BACTERIAL SPECIES After total genomic DNA was extracted as mentioned above, RT-qPCR was
conducted using SYBR Premix Ex TaqTM II kit (Takara) on a Light Cycler® System (Roche). Specific primers for total bacteria and specific bacterial species were synthesized by Sangon Biotech
(Shanghai) Co., Ltd. (China) and shown in Supplementary Table S3. Standard curves were obtained by constructing standard plasmids containing 16S rRNA genes. The copy numbers of each target
specific bacterial specie were calculated using the corresponding standard curves. The gene copy numbers were calculated using the following equation: [DNA concentration (mg/mL) × 6.0233 ×
1023 copies/mol] / [DNA size (bp) × 660 × 106]. Each sample was done in duplicate and average before further analysis. The relative abundance of target specific bacterial specie was the copy
number ratio of individual specific bacterial specie to total bacteria. WHOLE-GENOME SEQUENCING AND ANALYSIS Total genomic DNA was extracted from _B. acidifaciens_, and sequencing libraries
were prepared for Illumina and PacBio sequencing platforms and sequenced by Shanghai Majorbio Bio-pharm Technology (Shanghai, China) at the 100 × coverage. Raw reads were quality trimmed
with Fastp and assembled with Unicycler69. Genomic sequences of _B. acidifaciens_ were classified and annotated using eggNOG and KEGG databases. The genome-scale metabolic modeling was
constructed with MetaCyc pathways70. METABOLOMIC PROFILING AND ANALYSIS Targeted metabolomics profiling was conducted using Q300 Metabolite Array from Metabo-Profile Biotechnology (Shanghai,
China). Briefly, 5 mg feces were homogenized in 25 μL water, and the metabolites were extracted with 120 μL ice-cold methanol containing 306 internal standards representing more than 11
classes of metabolites, such as amino acids, bile acids, fatty acids, organic acids, carbohydrates, phenols, indoles, carnitines, pyridines, and benzoic acids. Following centrifugation at
18,000 × _g_ for 20 min, 20 μL supernatant was derivatized with prepared derivative reagents on Eppendorf epMotion Workstation. UPLC-MS/MS system was used to quantitate targeted metabolites
by comparing with internal standards. Metabolite identification was based on Metabo-Profile LIMS system71. Raw data files generated from UPLC-MS/MS were processed using the TMBQ software for
integration, calibration, and quantitation of each metabolite. The statistical analysis was performed on Metabo-Profile iMAP platform72. Differentially enriched metabolites were identified
using Wilcoxon rank-sum test, and adjusted _P_ < 0.05 was deemed significant. Z-scores were calculated after Log2 transformations of relative abundances of individual metabolites.
Heatmaps and volcano plots were generated using Complex Heatmap and ggplot in R language, respectively55. BACTERIAL CULTURE AND PENTADECANOIC ACID BIOSYNTHESIS IN VITRO _L. reuteri_ (BNCC
186135) and two _L. reuteri_ strains (#1 and #2) isolated in the mouse feces by our lab were cultured anaerobically in MRS medium, while _B. acidifaciens_ (BNCC 353574 and JCM10556) were
cultured anaerobically in BHI medium at 37°C. The culture medium used for in vitro pentadecanoic acid biosynthesis is comprised of casitone (10.00 g/L), yeast extract (2.50 g/L), MgSO4·7H2O
(45.00 mg/L), CaCl2·2H2O (90.00 mg/L), M9 salt (11.28 g/L), L-cysteine HCl (0.50 g/L), haemin (5.00 mg/L), and vitamin K (0.50 mg/L). GOS (1%, w/v), _L. reuteri_ (108 CFU/mL, 1%, v/v) or
GL-derived fecal supernatant (1%, v/v) was added when needed. MOUSE CECUM MICROBIOTA CULTIVATION AND _B. ACIDIFACIENS_ DETECTION After 8-week-old male C57BL/6 J mice euthanized, the caecum
was removed aseptically and its content (portions of about 100 μL) was transferred under CO2 flushing to PYG medium (Modified), including: trypticase peptone (5.00 g/L), peptone (5.00 g/L),
yeast extract (10.00 g/L), beef extract (5.00 g/L), glucose (5.00 g/L), K2HPO4 (2.04 g/L), Tween 80 (1.00 mL/L), CaCl2·2H2O (10.00 mg/L), MgSO7·7H2O (20.00 mg/L), KH2PO4 (40.00 mg/L), NaHO3
(0.40 g/L), NaCl (80.00 mg/L), resazurin (1.00 mg/L), vitamin K1 (1.00 μL/L), haemin (5.00 mg/L), L-cysteine-HCl (0.50 g/L), and distilled water (0.95 L)73. For _B. acidifaciens_ enriching
experiment, GOS (1 mM), _L. reuteri_ (106 CFU/mL) or their combination, as well as beta-alanine (1 mM), N-acetyl-D-glucosamine (1 mM), 4-methylhexanoic acid (1 mM) or myristic acid (1 mM)
was supplemented in the culture medium. The relative abundance of _B. acidifaciens_ in the culture medium was detected by RT-qPCR with specific primer as described above. CELLS CULTURE AND
TREATMENT Human Caco-2 cells and murine MODE-K cells were cultured in DMEM/F12 medium supplemented with 10% FBS, 100 U/mL penicillin, and 100 μg/mL streptomycin at 37°C and 5% CO2. After
treated with/without 10 μg/mL LPS, and/or 50 μM C15:0, and/or 1 μM dihydropyrimidinone (DHPM, Sigma) for 24 h, cells were harvested for further analysis. RNA EXTRACTION, REVERSE
TRANSCRIPTION, AND RT-QPCR Total RNA was extracted using TRIzol reagent (Invitrogen, USA) according to the manufacturer’s instructions. Reverse transcription was performed in duplicate using
Prime ScriptTM RT Kit (Takara, Japan), and qPCR was conducted using SYBR Premix Ex TaqTM II kit (Takara, Japan) on a Light Cycler® System (Roche, USA). Primers for RT-qPCR were synthesized
by Sangon Biotech (Shanghai) Co., Ltd. (China), and shown in Supplementary Table S3. The relative gene expressions were calculated using the 2−ΔΔCt method using β-actin as the reference
gene. RNA-SEQ AND DATA ANALYSIS RNA-seq of total RNA samples was performed on Illumina HiSeq X Ten platform. The raw paired end reads were trimmed and quality controlled by Fastp, and then
aligned to _Mus_musculus_ reference genome (GRCm39)74. Data analysis was performed on free online platform of Majorbio Cloud Platform (www.majorbio.com). Differentially expressed genes were
identified through pairwise comparisons using DESeq2 with |log2FC | > 1 and _q_ <0.05. KEGG analysis was performed at _q_ < 0.05 using Goatools and KOBAS75. Differential expression
of selected genes were further validated with RT-qPCR. The primers were synthesized by Sangon Biotech (Shanghai) Co., Ltd. (China) and shown in Supplementary Table S3. PROTEIN EXTRACTION,
QUANTIFICATION, AND WESTERN BLOT Proteins were extracted with RIPA buffer consisting of 50 mM Tris (pH 7.4), 150 mM NaCl, 1% Triton X-100, 1% sodium deoxycholate, 0.1% SDS, 2 mM sodium
pyrophosphate, 25 mM β-glycerophosphate, 1 mM EDTA, 1 mM Na3VO4, 0.5 μg/mL leupeptin, protease inhibitors, and phosphatase inhibitors. Extracted proteins were quantified with the BCA method.
Western blot was performed with the following antibodies: β-actin (Sigma-Aldrich, A5441, 1:1,000), Claudin-1 (Invitrogen, 2H10D10, 1:1,000), Occludin (Abcam, ab216327, 1:1,000), ZO-1
(Abcam, ab221547, 1:1,000), NF-κB p65 (Cell Signaling Technology, 8242, 1:1,000), p-NF-κB p65 (Cell Signaling Technology, 3033, 1:1,000), FATP4 (Proteintech, 11013-1-AP, 1:500), and
HRP-conjugated secondary antibody (Cell Signaling Technology, 91196, 1:10,000). After stripes collection, the signals were quantified using Image J software76. The uncropped and unprocessed
scans have been supplied in the Source Data file. STATISTICS & REPRODUCIBILITY No statistical methods were used to predetermine sample size for in vivo and in vitro experiments, but at
least three samples were used per experimental group and condition. Samples and experimental animals were randomly assigned to experimental groups. The investigators were blinded to
allocation during experiments and outcome assessment. Data were analyzed using SPSS 20.0 (IBM, United States). No data were excluded from the analysis. The Shapiro-Wilk test was performed to
assess the normality of each dataset. If _p_ >0.05, the data was deemed normally distributed, and two-tailed Student’s t test was used to detect statistical significance for two groups,
while one-way ANOVA with Tukey’s test was used for more than two groups. If _p_ <0.05, the two-tailed Mann-Whitney U test was used to detect statistical significance for two groups and
the Kruskal-Wallis test and post-hoc Dunn’s test for more than two groups. REPORTING SUMMARY Further information on research design is available in the Nature Portfolio Reporting Summary
linked to this article. DATA AVAILABILITY The metagenomic sequencing data, 16S rRNA sequencing data, whole-genome sequencing data, and RNA-seq data were available in the NCBI SRA database
under the accession numbers PRJNA1048359 and PRJNA1154812. The output of the metabolomics data and all other data generated during this study were available in Source Data provided with this
paper. Source data are provided with this paper. CODE AVAILABILITY All analyses were conducted using open-source software, which has been explicitly stated in the method section. No custom
code or mathematical algorithm was utilized in this study. REFERENCES * Kobayashi, T. et al. Ulcerative colitis. _Nat. Rev. Dis. Primers_ 6, 74 (2020). Article PubMed Google Scholar *
Zhang, Y. Z. & Li, Y. Y. Inflammatory bowel disease: pathogenesis. _World J. Gastroenterol._ 20, 91–99 (2014). Article ADS PubMed PubMed Central Google Scholar * Andersen, A. D. et
al. Synbiotics combined with glutamine stimulate brain development and the immune system in preterm pigs. _J. Nutr._ 149, 36–45 (2019). Article PubMed Google Scholar * Kolida, S. &
Gibson, G. R. Synbiotics in health and disease. _Annu. Rev. Food Sci. Technol._ 2, 373–393 (2011). Article PubMed Google Scholar * Ma, L. et al. Anti-Inflammatory Effect of clostridium
butyricum-derived extracellular vesicles in Ulcerative Colitis: Impact on host microRNAs expressions and gut microbiome profiles. _Mol. Nutr. Food Res._ 67, e2200884 (2023). Article PubMed
Google Scholar * Mu, Q., Tavella, V. J. & Luo, X. M. Role of _Lactobacillus reuteri_ in human health and diseases. _Front. Microbiol._ 9, 757 (2018). Article PubMed PubMed Central
Google Scholar * Wang, J., Tian, S., Yu, H., Wang, J. & Zhu, W. Response of colonic mucosa-associated microbiota composition, mucosal immune homeostasis, and barrier function to early
life galactooligosaccharides intervention in suckling piglets. _J. Agric. Food Chem._ 67, 578–588 (2019). Article CAS PubMed Google Scholar * Wu, Y. et al. Strain specificity of
lactobacilli with promoted colonization by galactooligosaccharides administration in protecting intestinal barriers during _Salmonella_ infection. _J. Adv. Res._ 56, 1–14 (2024). Article
CAS PubMed Google Scholar * Rattanaprasert, M. et al. Genes involved in galactooligosaccharide metabolism in _Lactobacillus reuteri_ and their ecological role in the gastrointestinal
tract. _Appl. Environ. Microb._ 85, e01788–01719 (2019). Article CAS Google Scholar * Li, Z., Liu, H., Xu, B. & Wang, Y. Enterotoxigenic _Escherichia coli_ interferes FATP4-dependent
long-chain fatty acid uptake of intestinal epithelial enterocytes via phosphorylation of ERK1/2-PPARγ pathway. _Front. Physiol._ 10, 798 (2019). Article PubMed PubMed Central Google
Scholar * Lin, M. H. et al. Fatty acid transport protein 4 is required for incorporation of saturated ultralong-chain fatty acids into epidermal ceramides and monoacylglycerols. _Sci. Rep._
9, 13254 (2019). Article ADS PubMed PubMed Central Google Scholar * Franzosa, E. A. et al. Gut microbiome structure and metabolic activity in inflammatory bowel disease. _Nat.
Microbiol._ 4, 293–305 (2018). Article PubMed PubMed Central Google Scholar * Weng, Y. J. et al. Correlation of diet, microbiota and metabolite networks in inflammatory bowel disease.
_J. Dig. Dis._ 20, 447–459 (2019). Article CAS PubMed Google Scholar * Lloyd-Price, J. et al. Multi-omics of the gut microbial ecosystem in inflammatory bowel diseases. _Nature_ 569,
655–662 (2019). Article ADS CAS PubMed PubMed Central Google Scholar * Di’Narzo, A. F. et al. Integrative analysis of the inflammatory bowel sisease serum metabolome improves our
understanding of genetic etiology and points to novel putative therapeutic targets. _Gastroenterology_ 162, 828–843.e811 (2022). Article PubMed Google Scholar * Diab, J. et al. Lipidomics
in Ulcerative Colitis reveal alteration in mucosal lipid composition associated with the disease state. _Inflamm. Bowel Dis._ 25, 1780–1787 (2019). Article PubMed Google Scholar *
Camilleri, M., Madsen, K., Spiller, R., van Meerveld, B. G. & Verne, G. N. Intestinal barrier function in health and gastrointestinal disease. _Neurogastroenterol. Motil._ 24, 503–512
(2012). Article CAS PubMed PubMed Central Google Scholar * Salim, S. Y. & Soderholm, J. D. Importance of disrupted intestinal barrier in inflammatory bowel diseases. _Inflamm. Bowel
Dis._ 17, 362–381 (2011). Article PubMed Google Scholar * Birchenough, G. & Hansson, G. C. Bacteria tell us how to protect our intestine. _Cell Host Microbe_ 22, 3–4 (2017). Article
CAS PubMed Google Scholar * Fan, L. et al. Gut microbiota bridges dietary nutrients and host immunity. _Sci. China Life Sci._ 66, 2466–2514 (2023). Article PubMed Google Scholar *
Yang, J. Y. et al. Gut commensal _Bacteroides acidifaciens_ prevents obesity and improves insulin sensitivity in mice. _Mucosal Immunol._ 10, 104–116 (2017). Article CAS PubMed Google
Scholar * Wang, H. et al. _Bacteroides acidifaciens_ in the gut plays a protective role against CD95-mediated liver injury. _Gut Microbes_ 14, 2027853 (2022). Article PubMed PubMed
Central Google Scholar * Nakajima, A. et al. A soluble fiber diet increases _Bacteroides fragilis_ group abundance and immunoglobulin A production in the gut. _Appl. Environ. Microbiol._
86, e00405–e00420 (2020). Article CAS PubMed PubMed Central Google Scholar * Peng, J. et al. _Saffron petal_, an edible byproduct of _Saffron_, alleviates dextran sulfate sodium-induced
colitis by inhibiting macrophage activation and regulating gut microbiota. _J. Agric. Food Chem._ 71, 10616–10628 (2023). Article CAS PubMed Google Scholar * Zheng, C. et al.
_Bacteroides acidifaciens_ and its derived extracellular vesicles improve DSS-induced colitis. _Front Microbiol._ 14, 1304232 (2023). Article PubMed PubMed Central Google Scholar * Yan,
D. et al. Fatty acids and lipid mediators in inflammatory bowel disease: From mechanism to treatment. _Front. Immunol._ 14, 1286667 (2023). Article CAS PubMed PubMed Central Google
Scholar * Brown, E. M., Clardy, J. & Xavier, R. J. Gut microbiome lipid metabolism and its impact on host physiology. _Cell Host Microbe_ 31, 173–186 (2023). Article CAS PubMed
PubMed Central Google Scholar * Heimerl, S. et al. Alterations in intestinal fatty acid metabolism in inflammatory bowel disease. _Biochim. Biophys. Acta_ 1762, 341–350 (2006). Article
CAS PubMed Google Scholar * Ma, C., Vasu, R. & Zhang, H. The role of long-chain fatty acids in inflammatory bowel disease. _Mediators Inflamm._ 2019, 8495913 (2019). Article PubMed
PubMed Central Google Scholar * Ediriweera, M. K., To, N. B., Lim, Y. & Cho, S. K. Odd-chain fatty acids as novel histone deacetylase 6 (HDAC6) inhibitors. _Biochimie_ 186, 147–156
(2021). Article CAS PubMed Google Scholar * Venn-Watson, S., Lumpkin, R. & Dennis, E. A. Efficacy of dietary odd-chain saturated fatty acid pentadecanoic acid parallels broad
associated health benefits in humans: Could it be essential? _Sci. Rep._ 10, 8161 (2020). Article ADS CAS PubMed PubMed Central Google Scholar * Fu, W. et al. Pentadecanoic acid
promotes basal and insulin-stimulated glucose uptake in C2C12 myotubes. _Food Nutr. Res._ 65, 4527 (2021). Article CAS Google Scholar * To, N. B., Truong, V. N., Ediriweera, M. K. &
Cho, S. K. Effects of combined pentadecanoic acid and tamoxifen treatment on tamoxifen resistance in MCF-7/SC breast cancer cells. _Int. J. Mol. Sci._ 23, 11340 (2022). Article CAS PubMed
PubMed Central Google Scholar * Venn-Watson, S. K. & Butterworth, C. N. Broader and safer clinically-relevant activities of pentadecanoic acid compared to omega-3: Evaluation of an
emerging essential fatty acid across twelve primary human cell-based disease systems. _PLoS One_ 17, e0268778 (2022). Article CAS PubMed PubMed Central Google Scholar * To, N. B.,
Nguyen, Y. T., Moon, J. Y., Ediriweera, M. K. & Cho, S. K. Pentadecanoic acid, an odd-chain fatty acid, suppresses the stemness of MCF-7/SC human breast cancer stem-like cells through
JAK2/STAT3 signaling. _Nutrients_ 12, 1663 (2020). Article CAS PubMed PubMed Central Google Scholar * Christian, F., Smith, E. L. & Carmody, R. J. The regulation of NF-κB subunits
by phosphorylation. _Cells_ 5, 12 (2016). Article PubMed PubMed Central Google Scholar * Park, M. H. & Hong, J. T. Roles of NF-κB in cancer and inflammatory diseases and their
therapeutic approaches. _Cells_ 5, 15 (2016). Article PubMed PubMed Central Google Scholar * Taniguchi, K. & Karin, M. NF-κB, inflammation, immunity and cancer: coming of age. _Nat.
Rev. Immunol._ 18, 309–324 (2018). Article CAS PubMed Google Scholar * Prada, M. et al. Association of the odd-chain fatty acid content in lipid groups with type 2 diabetes risk: A
targeted analysis of lipidomics data in the EPIC-Potsdam cohort. _Clin. Nutr._ 40, 4988–4999 (2021). Article CAS PubMed Google Scholar * Polonskaya, Y. V. et al. Balance of fatty acids
and their correlations with parameters of lipid metabolism and markers of inflammation in men with coronary atherosclerosis. _Bull. Exp. Biol. Med._ 164, 33–35 (2017). Article CAS PubMed
Google Scholar * Stallings, V. A. et al. Diagnosing malabsorption with systemic lipid profiling: pharmacokinetics of pentadecanoic acid and triheptadecanoic acid following oral
administration in healthy subjects and subjects with cystic fibrosis. _Int. J. Clin. Pharmacol. Ther._ 51, 263–273 (2013). Article CAS PubMed PubMed Central Google Scholar * Jenkins, B.
et al. The dietary total-fat content affects the in vivo circulating C15:0 and C17:0 fatty acid levels independently. _Nutrients_ 10, 1646 (2018). Article PubMed PubMed Central Google
Scholar * Maruyama, C. et al. Differences in serum phospholipid fatty acid compositions and estimated desaturase activities between Japanese men with and without metabolic syndrome. _J.
Atheroscler. Thromb._ 15, 306–313 (2008). Article CAS PubMed Google Scholar * Yoo, W. et al. Fatty acids in non-alcoholic steatohepatitis: Focus on pentadecanoic acid. _PLoS One_ 12,
e0189965 (2017). Article PubMed PubMed Central Google Scholar * Santaren, I. D. et al. Serum pentadecanoic acid (15:0), a short-term marker of dairy food intake, is inversely associated
with incident type 2 diabetes and its underlying disorders. _Am. J. Clin. Nutr._ 100, 1532–1540 (2014). Article CAS PubMed PubMed Central Google Scholar * Galdiero, E. et al.
Pentadecanoic acid against _Candida albicans_-_Klebsiella pneumoniae_ biofilm: towards the development of an anti-biofilm coating to prevent polymicrobial infections. _Res. Microbiol._ 172,
103880 (2021). Article CAS PubMed Google Scholar * Ricciardelli, A. et al. Pentadecanal and pentadecanoic acid coatings reduce biofilm formation of _Staphylococcus epidermidis_ on PDMS.
_Pathog Dis_ 78, ftaa012 (2020). Article CAS PubMed Google Scholar * Jenkins, B. J. et al. Odd chain fatty acids: New insights of the relationship between the gut microbiota, dietary
intake, biosynthesis and glucose intolerance. _Sci. Rep._ 7, 44845 (2017). Article ADS CAS PubMed PubMed Central Google Scholar * Dornan, K., Gunenc, A., Oomah, B. D. & Hosseinian,
F. Odd chain fatty acids and odd chain phenolic lipids (alkylresorcinols) are essential for diet. _J. Am. Oil Chem. Soc._ 98, 813–824 (2021). Article CAS Google Scholar * Pfeuffer, M.
& Jaudszus, A. Pentadecanoic and heptadecanoic acids: Multifaceted odd-chain fatty acids. _Adv. Nutr._ 7, 730–734 (2016). Article CAS PubMed PubMed Central Google Scholar * Zhang,
L., Liang, S., Zong, M., Yang, J. & Lou, W. Microbial synthesis of functional odd-chain fatty acids: A review. _World J. Microbiol. Biotechnol._ 36, 35 (2020). Article ADS PubMed
Google Scholar * Park, Y. K., Dulermo, T., Ledesma-Amaro, R. & Nicaud, J. M. Optimization of odd chain fatty acid production by _Yarrowia lipolytica_. _Biotechnol. Biofuels_ 11, 158
(2018). Article PubMed PubMed Central Google Scholar * Rezanka, T., Kolouchova, I. & Sigler, K. Precursor directed biosynthesis of odd-numbered fatty acids by different yeasts.
_Folia Microbiol. (Praha)_ 60, 457–464 (2015). Article CAS PubMed Google Scholar * Zhang, L. S. et al. Using 1-propanol to significantly enhance the production of valuable odd-chain
fatty acids by _Rhodococcus opacus_ PD630. _World J. Microbiol. Biotechnol._ 35, 164 (2019). Article PubMed Google Scholar * Wei, W. et al. _Parabacteroides distasonis_ uses dietary
inulin to suppress NASH via its metabolite pentadecanoic acid. _Nat. Microbiol._ 8, 1534–1548 (2023). Article CAS PubMed PubMed Central Google Scholar * Qin, N., Li, L., Wang, Z. &
Shi, S. Microbial production of odd-chain fatty acids. _Biotechnol. Bioeng._ 120, 917–931 (2023). Article CAS PubMed Google Scholar * Wu, H. & San, K. Y. Engineering _Escherichia
coli_ for odd straight medium chain free fatty acid production. _Appl. Microbiol. Biotechnol._ 98, 8145–8154 (2014). Article CAS PubMed Google Scholar * Yamamoto, T., Shimoyama, T.,
Umegae, S. & Matsumoto, K. Tacrolimus vs. anti-tumour necrosis factor agents for moderately to severely active ulcerative colitis: A retrospective observational study. _Aliment.
Pharmacol. Ther._ 43, 705–716 (2016). Article CAS PubMed Google Scholar * Torres, J. et al. Systematic Review of Effects of Withdrawal of Immunomodulators or Biologic Agents From
Patients With Inflammatory Bowel Disease. _Gastroenterology_ 149, 1716–1730 (2015). Article CAS PubMed Google Scholar * Bruscoli, S., Febo, M., Riccardi, C. & Migliorati, G.
Glucocorticoid therapy in inflammatory bowel disease: Mechanisms and clinical practice. _Front. Immunol._ 12, 691480 (2021). Article CAS PubMed PubMed Central Google Scholar * Ji, Y. et
al. Hydroxyproline attenuates dextran sulfate sodium-induced colitis in mice: Involvment of the NF-κB signaling and oxidative stress. _Mol. Nutr. Food Res._ 62, e1800494 (2018). Article
PubMed Google Scholar * Wu, Y. et al. Maternal galactooligosaccharides supplementation programmed immune defense, microbial colonization and intestinal development in piglets. _Food
Funct._ 12, 7260–7270 (2021). Article CAS PubMed Google Scholar * Bolyen, E. et al. Reproducible, interactive, scalable and extensible microbiome data science using QIIME 2. _Nat.
Biotechnol._ 37, 852–857 (2019). Article CAS PubMed PubMed Central Google Scholar * Noguchi, H., Park, J. & Takagi, T. MetaGene: prokaryotic gene finding from environmental genome
shotgun sequences. _Nucleic Acids Res._ 34, 5623–5630 (2006). Article CAS PubMed PubMed Central Google Scholar * Li, D., Liu, C. M., Luo, R., Sadakane, K. & Lam, T. W. MEGAHIT: an
ultra-fast single-node solution for large and complex metagenomics assembly via succinct de Bruijn graph. _Bioinformatics_ 31, 1674–1676 (2015). Article CAS PubMed Google Scholar * Fu,
L., Niu, B., Zhu, Z., Wu, S. & Li, W. CD-HIT: accelerated for clustering the next-generation sequencing data. _Bioinformatics_ 28, 3150–3152 (2012). Article CAS PubMed PubMed Central
Google Scholar * Li, R., Li, Y., Kristiansen, K. & Wang, J. SOAP: short oligonucleotide alignment program. _Bioinformatics_ 24, 713–714 (2008). Article CAS PubMed Google Scholar *
Buchfink, B., Xie, C. & Huson, D. H. Fast and sensitive protein alignment using DIAMOND. _Nat. Methods_ 12, 59–60 (2015). Article CAS PubMed Google Scholar * Wick, R. R., Judd, L.
M., Gorrie, C. L. & Holt, K. E. Unicycler: Resolving bacterial genome assemblies from short and long sequencing reads. _PLoS Comput. Biol._ 13, e1005595 (2017). Article ADS PubMed
PubMed Central Google Scholar * Weiss, A. S. et al. In vitro interaction network of a synthetic gut bacterial community. _ISME J_ 16, 1095–1109 (2022). Article CAS PubMed Google Scholar
* Ning, L. et al. Microbiome and metabolome features in inflammatory bowel disease via multi-omics integration analyses across cohorts. _Nat. Commun._ 14, 7135 (2023). Article ADS CAS
PubMed PubMed Central Google Scholar * Xie, G. et al. A metabolite array technology for precision medicine. _Anal. Chem._ 93, 5709–5717 (2021). Article CAS PubMed Google Scholar *
Weiss, G. A. et al. Intestinal inflammation alters mucosal carbohydrate foraging and monosaccharide incorporation into microbial glycans. _Cell. Microbiol._ 23, e13269 (2021). Article CAS
PubMed Google Scholar * Chen, S., Zhou, Y., Chen, Y. & Gu, J. Fastp: an ultra-fast all-in-one FASTQ preprocessor. _Bioinformatics_ 34, i884–i890 (2018). Article PubMed PubMed Central
Google Scholar * Xie, C. et al. KOBAS 2.0: a web server for annotation and identification of enriched pathways and diseases. _Nucleic Acids Res._ 39, W316–W322 (2011). Article CAS
PubMed PubMed Central Google Scholar * Li, J. et al. _Limosilactobacillus johnsoni_ and _Limosilactobacillus mucosae_ and their extracellular vesicles alleviate gut inflammatory injury by
mediating macrophage polarization in a lipopolysaccharide-challenged piglet model. _J. Nutr._ 153, 2497–2511 (2023). Article PubMed Google Scholar Download references ACKNOWLEDGEMENTS
This research was funded by National Natural Science Foundation of China (32125036, J.W.; 32172750, D.H.; 32330100, J.W.; and 32302765, Y.W.), Beijing Municipal Natural Science Foundation
(6232024, J.W.), China National Postdoctoral Program for Innovative Talents (BX20230417, Y.W.), China Postdoctoral Science Foundation (2022M723423, Y.W.), China Agricultural Research System
(CARS-35, J.W.), and 111 Project (B16044, J.W.). AUTHOR INFORMATION AUTHORS AND AFFILIATIONS * State Key Laboratory of Animal Nutrition and Feeding, College of Animal Science and Technology,
China Agricultural University, Beijing, 100193, China Yujun Wu, Xiangyu Zhang, Xiaoyi Liu, Qian Xu, Jinbiao Zhao, Zhaolai Dai, Dandan Han & Junjun Wang * Department of General Surgery,
Jiangyin People’s Hospital Affiliated to Nantong University, Jiangsu, 214400, China Zhenguo Zhao * College of Animal Sciences and Technology, Huazhong Agricultural University, Wuhan, 430070,
China Shiyu Tao * Department of Animal and Food Sciences, Oklahoma State University, Stillwater, 74078, USA Guolong Zhang Authors * Yujun Wu View author publications You can also search for
this author inPubMed Google Scholar * Xiangyu Zhang View author publications You can also search for this author inPubMed Google Scholar * Xiaoyi Liu View author publications You can also
search for this author inPubMed Google Scholar * Zhenguo Zhao View author publications You can also search for this author inPubMed Google Scholar * Shiyu Tao View author publications You
can also search for this author inPubMed Google Scholar * Qian Xu View author publications You can also search for this author inPubMed Google Scholar * Jinbiao Zhao View author publications
You can also search for this author inPubMed Google Scholar * Zhaolai Dai View author publications You can also search for this author inPubMed Google Scholar * Guolong Zhang View author
publications You can also search for this author inPubMed Google Scholar * Dandan Han View author publications You can also search for this author inPubMed Google Scholar * Junjun Wang View
author publications You can also search for this author inPubMed Google Scholar CONTRIBUTIONS Y.W. performed most of the experiments and drafted the manuscript. X.Z. and X.L. analyzed the
data of shotgun metagenome sequencing, 16S rRNA sequencing, RNA-seq, whole-genome sequencing and metabolomics. Q.X. and S.T. assisted with the in vitro bacterial culture experiments and
animal experiments. Z.Z. provided the clinical samples. J.Z., Z.D., and D.H. commented on the study. G.Z. revised the manuscript. J.W. supervised the study and revised the manuscript.
CORRESPONDING AUTHOR Correspondence to Junjun Wang. ETHICS DECLARATIONS COMPETING INTERESTS The authors declare no competing interests. PEER REVIEW PEER REVIEW INFORMATION _Nature
Communications_ thanks Lusheng Huang, Mi-Na Kweon and Andre Marette for their contribution to the peer review of this work. A peer review file is available. ADDITIONAL INFORMATION
PUBLISHER’S NOTE Springer Nature remains neutral with regard to jurisdictional claims in published maps and institutional affiliations. SUPPLEMENTARY INFORMATION SUPPLEMENTARY INFORMATION
PEER REVIEW FILE REPORTING-SUMMARY SOURCE DATA SOURCE DATA RIGHTS AND PERMISSIONS OPEN ACCESS This article is licensed under a Creative Commons Attribution-NonCommercial-NoDerivatives 4.0
International License, which permits any non-commercial use, sharing, distribution and reproduction in any medium or format, as long as you give appropriate credit to the original author(s)
and the source, provide a link to the Creative Commons licence, and indicate if you modified the licensed material. You do not have permission under this licence to share adapted material
derived from this article or parts of it. The images or other third party material in this article are included in the article’s Creative Commons licence, unless indicated otherwise in a
credit line to the material. If material is not included in the article’s Creative Commons licence and your intended use is not permitted by statutory regulation or exceeds the permitted
use, you will need to obtain permission directly from the copyright holder. To view a copy of this licence, visit http://creativecommons.org/licenses/by-nc-nd/4.0/. Reprints and permissions
ABOUT THIS ARTICLE CITE THIS ARTICLE Wu, Y., Zhang, X., Liu, X. _et al._ Galactooligosaccharides and _Limosilactobacillus reuteri_ synergistically alleviate gut inflammation and barrier
dysfunction by enriching _Bacteroides acidifaciens_ for pentadecanoic acid biosynthesis. _Nat Commun_ 15, 9291 (2024). https://doi.org/10.1038/s41467-024-53144-1 Download citation *
Received: 16 February 2024 * Accepted: 01 October 2024 * Published: 28 October 2024 * DOI: https://doi.org/10.1038/s41467-024-53144-1 SHARE THIS ARTICLE Anyone you share the following link
with will be able to read this content: Get shareable link Sorry, a shareable link is not currently available for this article. Copy to clipboard Provided by the Springer Nature SharedIt
content-sharing initiative