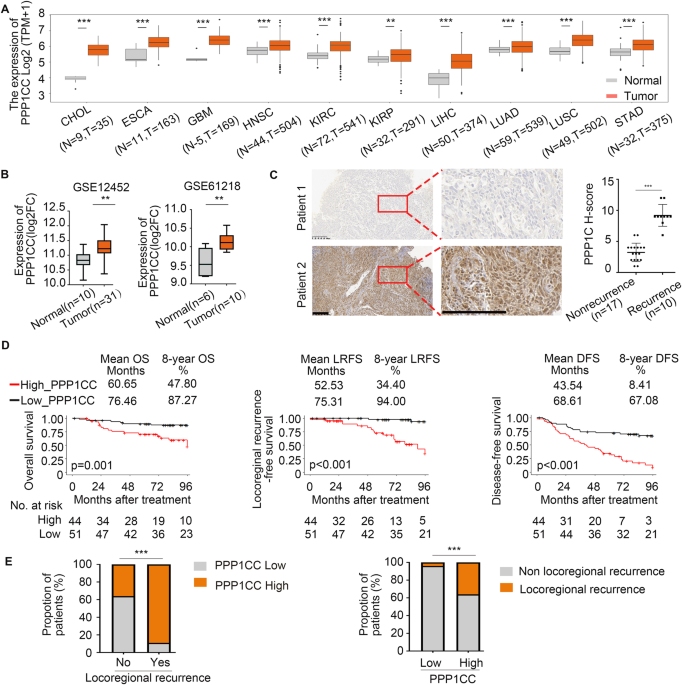
- Select a language for the TTS:
- UK English Female
- UK English Male
- US English Female
- US English Male
- Australian Female
- Australian Male
- Language selected: (auto detect) - EN
Play all audios:
ABSTRACT Protein phosphatase 1 catalytic subunit gamma (PPP1CC) promotes DNA repair and tumor development and progression, however, its underlying mechanisms remain unclear. This study
investigated the molecular mechanism of PPP1CC’s involvement in DNA repair and the potential clinical implications. High expression of PPP1CC was significantly correlated with
radioresistance and poor prognosis in human nasopharyngeal carcinoma (NPC) patients. The mechanistic study revealed that PPP1CC bound to Ku70/Ku80 heterodimers and activated DNA-PKcs by
promoting DNA-PK holoenzyme formation, which enhanced nonhomologous end junction (NHEJ) -mediated DNA repair and led to radioresistance. Importantly, BRCA1-BRCA2-containing complex subunit 3
(BRCC3) interacted with PPP1CC to enhance its stability by removing the K48-linked polyubiquitin chain at Lys234 to prevent PPP1CC degradation. Therefore, BRCC3 helped the overexpressed
PPP1CC to maintain its high protein level, thereby sustaining the elevation of DNA repair capacity and radioresistance. Our study identified the molecular mechanism by which PPP1CC promotes
NHEJ-mediated DNA repair and radioresistance, suggesting that the BRCC3-PPP1CC-Ku70 axis is a potential therapeutic target to improve the efficacy of radiotherapy. You have full access to
this article via your institution. Download PDF SIMILAR CONTENT BEING VIEWED BY OTHERS USP44 REGULATES IRRADIATION-INDUCED DNA DOUBLE-STRAND BREAK REPAIR AND SUPPRESSES TUMORIGENESIS IN
NASOPHARYNGEAL CARCINOMA Article Open access 25 January 2022 LNCRNA LINC00312 SUPPRESSES RADIOTHERAPY RESISTANCE BY TARGETING DNA-PKCS AND IMPAIRING DNA DAMAGE REPAIR IN NASOPHARYNGEAL
CARCINOMA Article Open access 04 January 2021 PRDM15 INTERACTS WITH DNA-PK-KU COMPLEX TO PROMOTE RADIORESISTANCE IN RECTAL CANCER BY FACILITATING DNA DAMAGE REPAIR Article Open access 19
November 2022 INTRODUCTION The most fatal type of DNA damage is double-strand DNA breaks (DSBs), hence DSB repair is critical for cell survival [1]. Most DSBs are repaired by two main
pathways: homologous recombination (HR) and nonhomologous end junction (NHEJ). If cells cannot properly repair DSBs, this usually leads to genomic mutations or treatment failure [2,3,4,5].
HR, an accurate repair mode, ensures the high-fidelity transmission of genetic information. However, NHEJ is a simple and efficient repair method particularly suitable for severe DSB damage
[6]. Defects in NHEJ are associated with developmental abnormalities[7, 8] and severe combined immunodeficiency [9]. NHEJ leads to cancer development via chromosomal translocation and
chromosome fragmentation [10,11,12]. Additionally, some NHEJ factors are overexpressed in tumor tissues, and hyperactive NHEJ leads to resistance to chemotherapy and radiotherapy [13, 14].
LRRC31 and USP44 promote radiosensitization by inhibiting the NHEJ pathway [15, 16]. METTL1 and RFC4 contribute to radioresistance by promoting NHEJ-mediated DSB repair [17, 18]. Together,
NHEJ is one of the main mechanisms underlying radioresistance in radiotherapy for malignant tumors. Phosphoprotein phosphatases (PPPs) are a protein filament/threonine phosphatase family,
composed of seven subfamilies, namely PP1, PP2A, PP2B, PP4, PP5, PP6, and PP7. PPPs are involved in many types of DNA repair by regulating the phosphorylation of related protein molecules in
the DNA repair pathway. PP5 promotes NHEJ by dephosphorylating Abl interactor-1 [19]; PP4 engages in HR-mediated DNA repair via interacting with CNTRB [20] or dephosphorylating RPA2 [21];
PP2A binds to BRCA2 for HR-mediated DNA repair [22]. PPPs contain Protein phosphatase catalytic subunits (PPPCs) and regulatory subunits. The PPPCs interact with different regulatory
subunits to control their functional specificity and play an important role in DNA repair pathways [23]. For example, overexpressed PPP4C mediates dephosphorylation of KAP1 and inhibits NHEJ
repair [24]; PPP6C affects NHEJ by regulating DNA-PKcs and γ-H2AX dephosphorylation [25]; PPP1CA affects the formation of RAD51 nuclear foci by regulating the phosphorylation of BRCA1,
thereby enhancing the HR repair ability of mouse breast cancer cells after DNA damage [26]. PPP1CA can also dephosphorylate multiple sites of ATM and ATR, thereby regulating BRCA1
phosphorylation [27, 28], which is involved in the repair of DNA double-strand breaks (DSBs) through HR, NHEJ [29,30,31], and nucleotide excision repair [32, 33]. PPP1CC binds to DNA-PKcs
and works with regulatory subunit PNUT to fine-tune DNA-PKcs phosphorylation to control the NHEJ pathway in Hela cells [34]. In our previous study on the promotion of radioresistance by
BRCA1-BRCA2-containing complex subunit 3 (BRCC3) in nasopharyngeal carcinoma (NPC) [35], we unexpectedly found that PPP1CC interacts with BRCC3; however, whether this correlation affects
PPP1CC expression and mediates NHEJ to cause radioresistance is unclear. The purpose of this study is to investigate how PPP1CC expression is regulated and acts on the DNA repair pathway
following irradiation (IR) and to explore its relationship with the prognosis of patients with NPC. RESULTS OVEREXPRESSION OF PPP1CC IS SIGNIFICANTLY CORRELATED WITH POOR PROGNOSIS AND TUMOR
RELAPSE Radioresistance is one of the hallmarks of NPC progression [36,37,38,39,40]. _PPP1CC_ expression was elevated in ten different tumor types using The Cancer Genome Atlas (TCGA)
database (Fig. 1A). Two GEO datasets (GSE12452 and GSE61218) also identified _PPP1CC_ as one of the oncogenes in NPC tissues (Fig. 1B). The Clinical Proteomic Tumor Analysis Consortium
(CPTAC) database analysis showed that PPP1CC protein expression was highly expressed in six different tumors using unpaired (Fig. S1A) and paired analysis (Fig. S1B), Consistent with these
findings, PPP1CC protein and mRNA levels were higher in eleven NPC cell lines (6-10B, CNE2, S18, SUNE1, CNE1, HONE1, HNE1, S26, HK1, 5–8F, and C666) than normal nasopharyngeal epithelial
cell line (NP69; Fig. S1C, D). Compared with nonrecurrence NPC, PPP1CC was significantly upregulated in recurrent NPC tissues (Fig. 1C), which was confirmed using 27 NPC tissues (Table S1).
These results suggested that PPP1CC may promote the development of NPC. We conducted immunohistochemical staining on 95 NPC tissue samples to investigate the clinical relevance of PPP1CC
protein levels and assessed its expression using the histochemistry (H)-score. We categorized the samples as low or high based on PPP1CC expression and integrated these findings with the
clinical data. Kaplan−Meier analysis demonstrated significant differences in overall survival (OS), locoregional recurrence-free survival (LRFS), and disease-free survival (DFS) between the
two groups (Fig. 1D). Our analysis further revealed that high PPP1CC staining in tumor samples was associated with locoregional recurrence (Fig. 1E). Compared with patients with low
expression of PPP1CC, the relative risk (RR) [41] of locoregional recurrence in patients with high expression of PPP1CC was 9.333 (0.364/0.039), that is, the risk of locoregional recurrence
in patients with high expression of PPP1CC within 8 years was 9.333 times higher than that in patients with low expression of PPP1CC. Higher PPP1CC expression represented a significantly
higher incidence of TNM_stage, locoregional recurrence, disease, and death (Table S2). Overall, our findings showed that higher PPP1CC expression was a predictor for poor prognosis and
relapse in NPC patients. PPP1CC ENHANCES RADIORESISTANCE VIA PROMOTING NHEJ-MEDIATED DSB REPAIR To validate whether various PPP1CC expression states affect radiosensitivity, we generated NPC
cells with stable knockdown of PPP1CC _(_sh_PPP1CC)_ and overexpressed PPP1CC. Western blotting and RT-qPCR analyses confirmed the efficiency of PPP1CC expression (Fig. S2A, B). We found
that PPP1CC downregulation sensitized NPC cells to irradiation (Fig. 2A, S3A). PPP1CC knockdown NPC cells inhibited proliferation (Fig. 2B, S3B) and migration (Fig. 2C, S3C) following
irradiation. PPP1CC upregulation could enhance radioresistance. Our results showed that PPP1CC overexpression significantly increased irradiation resistance, as demonstrated in cloning,
proliferation and migration assays (Fig. S4A–C, S5A–C), suggesting that high expression of PPP1CC promotes radioresistance. PPP1CC is a positive regulator of DSB repair. We conducted the
DNA-neutral comet assay that detects DSBs at the individual cell level and analyzed comet formation under neutral conditions in NPC cells at 0, 0.5, 4, and 24 h after irradiation. The
results showed that the tail moments in PPP1CC knockdown cells were longer than those in shNC cells (Fig. 2D, S3D). Meanwhile, PPP1CC overexpression shortened tail moments (Fig. S4D, S5D).
Next, we examined γ-H2AX, which is phosphorylated under DSBs induction [42]. The results of western blotting showed that γ-H2AX expression in PPP1CC knockdown cells further increased after
irradiation (Fig. 2E, S3E). The number of γ-H2AX-positive foci was higher in PPP1CC knockdown cells, and the difference persisted throughout 24 h using immunofluorescence assay (Fig. 2F,
S3F). In contrast, PPP1CC overexpression cells had lower γ-H2AX levels and fewer γ-H2AX-positive foci in the post-irradiation recovery phase (Fig. S4E-F, S5E-F). These results suggested that
PPP1CC enhanced DSB repair. We then used the NHEJ and HR reporter system to determine which DSB repair pathway was regulated by PPP1CC. The outcomes demonstrated that while HR activity was
unaffected, PPP1CC knockdown dramatically decreased NHEJ-mediated DSB repair (Fig. 2G, S3G, S6A–D). Conversely, overexpression of PPP1CC increased repair efficiency of NHEJ without affecting
HR (Fig. S4G, S5G, S6E–F). Together, PPP1CC facilitates DSB repair by promoting the NHEJ pathway. To ensure the target specificity of sh_PPP1CC_, we carried out rescue experiments and
indicated that re-expression of PPP1CC in sh_PPP1CC_ HK1 or HONE1 cells restored DNA repair and shortened the tail moments using the neutral comet DNA repair assay (Fig. S7). Consistently,
the immunofluorescent analysis showed that the number of γ-H2AX foci was significantly higher in sh_PPP1CC_ cells than in shNC cells at 0.5, 4, and 24 h post-IR, and this difference could be
abrogated by re-expression of PPP1CC in sh_PPP1CC_ cells (Fig. S8). We next used reporter assay to determine which of these two DNA repair pathways, HR and NHEJ, might be regulated. We
found that the inhibition of PPP1CC knockdown on NHEJ DSB repair efficiency could be reversed by expression of the exogenous PPP1CC (Fig. S9, S10). BRCC3 INTERACTS WITH PPP1CC PROTEIN AND
PREVENTS THE DEGRADATION OF PPP1CC THROUGH ITS DEUBIQUITINATION The interaction between BRCC3 and PPP1CC was found by mass spectrometry analysis (Fig. 3A, S11A, and Table S3). The exogenous
and endogenous interactions of BRCC3 with PPP1CC were confirmed using Coimmunoprecipitation (Co-IP) (Fig. 3B, C, S11B), and this association appeared constitutive and did not be affected at
4 h following IR using endogenous Co-IP (Fig. S11C). In vitro GST pull-down assay indicated the direct interaction between PPP1CC and endogenous BRCC3 (Fig. S11D). Additionally, BRCC3 and
PPP1CC were colocalized in the nucleus following irradiation (Fig. 3D). As both are radioresistant proteins, there may be a positive correlation. BRCC3, a deubiquitination enzyme, functions
as a deubiquitinase (DUB) and deubiquitinate target proteins to stabilize them. Therefore, we hypothesized that PPP1CC is regulated by BRCC3 deubiquitination. To confirm this hypothesis, we
discovered that BRCC3 overexpression increased PPP1CC protein expression but had no effect on mRNA expression (Fig. 3E). Moreover, the positive regulation of BRCC3 on PPP1CC remained at 4 h
after 6 Gy, and did not decrease or increase (Fig. S11E-F), indicating that the regulation of PPP1CC by BRCC3 may be independent of DNA damage. BRCC3 overexpression considerably prevented
PPP1CC degradation after cycloheximide (CHX) treatment, while BRCC3 knockdown enhanced PPP1CC degradation, indicating that BRCC3 could prolong the half-life of the PPP1CC protein (Fig. 3F).
Ubiquitin‒proteasome and lysosomal pathway are two main pathways that affect protein degradation. To study the pathway through which BRCC3 inhibits PPP1CC degradation, we added proteasome
inhibitor MG132 and lysosome inhibitor chloroquine (CQ) to the HK1 cells co-transfected with _BRCC3_ and _PPP1CC_. We found that MG132, but not CQ, contributed to the BRCC3-mediated
stabilization of PPP1CC. (Fig. 3G). We also detected endogenous PPP1CC expression by treating NPC cells that overexpressed or knocked down BRCC3 with MG132. The results showed that BRCC3
positively regulated the protein expression of PPP1CC through the ubiquitin-proteasome pathway (Fig. S11G-H), and this regulation was not affected at 4 h post IR 6 Gy (Fig. S11G).
Furthermore, we investigated proteasome 20 S, which plays a crucial role in the ubiquitin pathway of protein degradation. Its level decreased after BRCC3 upregulation, while BRCC3 was
down-regulated, the levels were accordingly increased (Fig. S11I-J). Collectively, our results indicated that BRCC3 upregulated the PPP1CC protein via the ubiquitin-proteasome pathway. BRCC3
has deubiquitinating activity due to the member of the DUB family. We evaluated its impact on the deubiquitination of PPP1CC and found that BRCC3 overexpression reduced the
polyubiquitination of PPP1CC (Fig. 3H). Moreover, overexpression BRCC3 removed the K48-linked ubiquitin chain of PPP1CC, rather than the K63-linked ubiquitin chain (Fig. 3H). Conversely,
BRCC3 knockdown had the opposite effect (Fig. 3I). Additionally, the deubiquitinase mutant E33A of BRCC3 lost the ability to stabilize and deubiquitinate PPP1CC (Fig. S11K, L). These results
provided supports for the role of BRCC3 in stabilizing PPP1CC through deubiquitination and highlighted the importance of the K48-linked ubiquitination pathway in the regulation of PPP1CC
stability. To investigate the specific site of PPP1CC deubiquitination targeted by BRCC3, four K/R substitution (KR) mutants of PPP1CC were obtained using mass spectrometry analysis (Fig.
S12A). We investigated which mutations affected PPP1CC stabilization induced by BRCC3 deubiquitination, and found that K234R did not increase the level of PPP1CC (Fig. S12B). In the
denaturing-IP assays, without BRCC3 overexpression, PPP1CC mutants (K6R, K98R, K234R) had stronger ubiquitination levels compared with wild-type (WT)-PPP1CC while K260R had weaker
ubiquitination levels, indicating that all PPP1CC KR mutants could affect ubiquitination levels. Comparing the degree of ubiquitination at various mutation sites in cells with overexpression
of BRCC3 showed that PPP1CC ubiquitination was not resistant to BRCC3 deubiquitylation on other lysines except for K234 (Fig. 3J). We also compared the degree of ubiquitination at each
mutation site after knocking down _BRCC3_ and confirmed that the knocking down of _BRCC3_ had the weakest effect on the ubiquitination of the K234R site of PPP1CC (Fig. S12C). Collectively,
BRCC3 targeted the K234 site for deubiquitination of PPP1CC. BRCC3 inhibited the Lys234-mediated K48-linked ubiquitination of PPP1CC and prevented its degradation. PPP1CC BINDS TO KU70/KU80
AND ACTIVATES DNA-PKCS BY ENHANCING DNA-PK HOLOENZYME FORMATION Previous studies have reported that PPP1CC could directly interact with Ku70 and DNA-PKcs in Hela cells [34]. Hitpredict
website (www.hitpredict.org) also predicts that the interacting protein for PPP1CC is Ku70 [43]. To determine whether PPP1CC binds to Ku70, Ku80 and DNA-PKcs in NPC cells, endogenous Co-IP
was used to examine the interactions. PPP1CC interacted with Ku70 and Ku80, but not with DNA-PKcs in NPC cells, and the interactions were significantly enhanced at 4 h post-IR (Fig. 4A),
which may due to the activation of the NHEJ pathway by the interaction of PPP1CC with Ku70 and Ku80 post-IR. As Ku70/Ku80 itself is a heterodimer, we did not study these two proteins, but
focused on Ku70. We also confirmed that PPP1CC directly interacted with endogenous Ku70 in vitro (Fig. S11D). Additionally, both PPP1CC and Ku70 were colocalized in the nucleus following
irradiation (Fig. 4B), as were PPP1CC and Ku80 (Fig. S13A). PPP1CC did not change Ku70 and Ku80 expression post-IR (Fig. 4C, S13B). To further understand the role of the BRCC3-PPP1CC axis in
the NHEJ pathway after irradiation in NPC, we determined that upregulation of BRCC3 promoted the recruitment of Ku70 by PPP1CC (Fig. 4D). We further found that knocking down BRCC3 or
overexpression of PPP1CC in knockdown BRCC3 cells did not affect Ku70 levels with or without IR (Fig. 4E, S13C). Consistently, we confirmed that different expressed BRCC3 did not alter the
expression of Ku70 and Ku80 after IR by western blot analysis. (Fig. 4F, S13D). Autophosphorylation of DNA-PKcs is critical for NHEJ pathway. We surprisedly found that the upregulation of
PPP1CC expression promoted the phosphorylation of DNA-PKcs at Serine 2056 post-IR, as did the upregulation of BRCC3 expression (Figs. 4C, 4F). Conversely, knocking down PPP1CC or BRCC3
inhibited DNA-PKcs activation following IR (Fig. S13B, S13D). Furthermore, overexpression of PPP1CC reversed this reduction of DNA-PKcs phosphorylation caused by knockdown of BRCC3 after
irradiation (Fig. 4G). Combined, the BRCC3-PPP1CC axis enhances DNA-PKcs phosphorylation. We investigated the effect of PPP1CC-Ku70/Ku80 association on DNA-PK complex formation. Up- or
downregulation of PPP1CC did not change the binding of Ku70 and Ku80, rather, it positively regulated the recruitment of DNA–PKcs (Fig. S13E). Chen et al. reported the similar mechanism by
which LRRC31 affects the DNA-PKcs activation [15]. This supported the results that PPP1CC and BRCC3 positively regulated DNA-PKcs phosphorylation (Figs. 4C, 4F, S13B, S13D). Collectively,
PPP1CC bound to Ku70/Ku80, but not to DNA-PKcs, and activated DNA-PKcs by enhancing the formation of DNA-PK holoenzyme. ELEVATED PPP1CC PLAYS A CRITICAL ROLE IN RADIORESISTANCE BY ENHANCING
NHEJ-MEDIATED DNA REPAIR VIA REVERSING THE RADIOSENSITIZING EFFECT OF KNOCKDOWN BRCC3 To confirm the BRCC3-PPP1CC axis effect in vitro and in vivo following irradiation, we generated
shNC+VEC, _shBRCC3#1_ + VEC, and _shBRCC3#1_ + _PPP1CC_ NPC cells (Fig. S14). Overexpression of PPP1CC reversed the inhibitory effects of BRCC3 knockdown on NPC cell cloning, proliferation,
and migration (Fig. 5A–C). In comparison to the _shBRCC3#1_ + VEC group, tumor volume and weight in the _shBRCC3#1_ + _PPP1CC_ group were significantly increased following irradiation,
suggesting that the radiosensitization effect of BRCC3 knockdown was nearly fully reversed by PPP1CC overexpression in vivo (Fig. 5D). These results indicated that the BRCC3-PPP1CC axis
mediates radioresistance in NPC cells. BRCC3-PPP1CC axis promotes the NHEJ pathway. Neutral comet assay was carried out to examine the effect of irradiation on DSBs in shNC+VEC, _shBRCC3#1_
+ VEC, and _shBRCC3#1_ + _PPP1CC_ groups of HK1 and HONE1 cells at 0, 0.5, 4, and 24 h post-IR, and found that the tail moments of _shBRCC3#1_ + VEC cells were much longer than those of
shNC+VEC cells following irradiation. Moreover, PPP1CC overexpression reversed the effect of prolonged tail moments in _shBRCC3#1_ + VEC cells (Fig. 6A). Additionally, BRCC3 knockdown
increased the production of γ-H2AX foci, which was reversed by PPP1CC overexpression (Fig. 6B), suggesting that BRCC3 promotes DSB repair by influencing PPP1CC. The NHEJ and HR reporting
systems were used to determine which DSB repair pathway was affected by the BRCC3-PPP1CC axis. _shBRCC3#1_ + _PPP1CC_ cells expressed much more GFP+ than _shBRCC3#1_ + VEC cells, thereby
promoting NHEJ-mediated DNA repair (Fig. 6C, S15). These results demonstrated that BRCC3 targets PPP1CC to promote NHEJ-mediated DNA repair and radioresistance in NPC cells. HIGH EXPRESSION
OF THE PROTEINS IN THE BRCC3-PPP1CC-KU70 PATHWAY IS ASSOCIATED WITH RECURRENCE OF NPC To evaluate the potential signaling of the BRCC3-PPP1CC-Ku70 axis in NPC patients, BRCC3, PPP1CC, and
Ku70 were stained by immunohistochemical analysis in 95 NPC tissue samples (Fig. 7A). The results showed that PPP1CC positively correlated with the expression of BRCC3 and Ku70 respectively
(Fig. 7B). Also, higher BRCC3 expression was in line with worse prognosis, while Ku70 did not show a significant prognostic correlation (Fig. S16). Therefore, we next only studied the
prognostic significance of PPP1CC and BRCC3 expressions in NPC patients. We found that the patients with high PPP1CC and BRCC3 expressions had the worst OS, RFS and DFS (Fig. 7C, S17A).
Additionally, receiver operating characteristic (ROC) curve analysis was used to analyze the prediction accuracy of OS, LRFS, and DFS by comparing their areas under the curve (AUC). The
results showed that PPP1CC combined with BRCC3 for the prediction accuracy was prior to the single indicator (Fig. 7D, S17B). Together, high expression of BRCC3-PPP1CC-Ku70 pathway proteins
is linked to a poor prognosis and indicates recurrence in NPC patients, suggesting that BRCC3-PPP1CC-Ku70 axis may be a potential therapeutic target. DISCUSSION Our findings demonstrate that
the BRCC3-PPP1CC-Ku70 pathway in NPC cells is involved in NHEJ repair and radioresistance. High expression of PPP1CC considerably enhances the radioresistance in NPC. Mechanically, PPP1CC
binds to Ku70/Ku80 heterodimer and activates DNA-PKcs by promoting the formation of DNA-PK holoenzyme, thereby enhancing NHEJ-mediated DNA repair. Moreover, we confirmed that BRCC3 manitains
PPP1CC protein stability by removing its K48-linked polyubiquitin chain at Lys 234 in NPC cells (Fig. 8). Radioresistance is one of the key reasons leading to poor prognosis in malignant
tumors [44,45,46]. Understanding the molecular mechanism of radioresistance can deliver accurate radiotherapy [47,48,49,50,51]. Our research indicated that highly expressed PPP1CC was
associated with radioresistance and poor prognosis. To date, the role of PPP1CC in different tumors remains controversial. PPP1CC promotes the development of hepatocellular carcinoma by
activating the TRAF6/NF-κB signaling pathway [52]. High expression of PPP1CC predicted poor prognosis of liver cancer, breast cancer, osteogenic tumors, and colorectal cancer [53,54,55,56].
On the other hand, PPP1CC, as a tumor suppressor, can enhance the sensitivity of cancer cells to DNA-damaging drugs, and the mechanism is related to activation of the Schlafen 11 (SLFN11)
and inhibition of the translation of ATR and ATM kinases [57]. Herein, PPP1CC-mediated radioresistance in NPC was attributed to the positive activation of NHEJ repair by the
BRCC3-PPP1CC-Ku70 pathway. DNA-PKcs autophosphorylation is crucial for initiating NHEJ. After Ku70/Ku80 heterodimer recognizes IR-induced DSBs, DNA-PKcs is rapidly recruited to DSBs to form
the DNA-PK holoenzyme with Ku70/Ku80, which leads to the autophosphorylation of DNA-PKcs [2, 58, 59]. In most of the past studies, binding to DNA-PKcs was required for the DNA-PKcs
autophosphorylation. Zhu et al. demonstrated that the PPP1CC/PNUTS complex binds to the DNA-PK complex and activates DNA-PKcs. It is due to PNUTS, as an inhibitory regulator of PP1, inhibits
DNA-PKcs dephosphorylation caused by PPP1CC [34]. However, some studies showed that DNA-PKcs autophosphorylation is affected by the formation of DNA-PK holoenzyme without binding to
DNA-PKcs. Chen et al. indicated that LRRC31 affects DNA-PKcs phosphorylation by influencing the formation of DNA-PK holoenzyme. LRRC31 interacted with Ku70/Ku80 and did not interact with
DNA-PKcs, affecting DNA-PKcs recruitment and activation [15]. Our findings showed that PPP1CC interacted with Ku70/Ku80, but not with DNA-PKcs in NPC cells. The interactions were enhanced
after irradiation, which may be related to the activation of the NHEJ pathway by the interaction of PPP1CC with Ku70 and Ku80. Furthermore, PPP1CC/Ku70/Ku80 complex enhanced DNA-PKcs
recruitment and autophosphorylation by promoting DNA-PK holoenzyme formation in NPC cells. Together, we indicated the precise mechanism by which the PPP1CC/Ku70/Ku80 complex modulates
DNA-PKcs autophosphorylation. The deubiquitinase activity of BRCC3 is essential for PPP1CC protein expression. BRCC3 removed the K48-linked polyubiquitin chain at Lys234 of PPP1CC to
maintain its protein level. BRCC3 is previously found to be highly expressed in breast cancer, and the mechanism by which it mediates radioresistance involves the mediation of BRCA1
phosphorylation to promote HR and regulate the G2/M checkpoint, thereby resulting in cell survival and radioresistance after DNA damage [60, 61]. BRCC3 promotes the development of bladder
cancer through the activation of the NF-κB signaling pathway [62] and can also accelerate the progression of gastric cancer by activating the PI3K/Akt/mTOR pathway [63]. However, the
specific mechanism by which BRCC3, as a DUB, mediates radioresistance remains unclear. Our results suggested that BRCC3 interacted with PPP1CC to enhance its protein stability via
deubiquitination, thereby maintaining high DNA repair activity, leading to radioresistance and poor clinical outcomes. To better understand the relationship between the BRCC3-PPP1CC-Ku70
pathway and the NPC prognosis, we conducted immunohistochemical staining in NPC tissues and found that PPP1CC was positively correlated with the protein expressions of BRCC3 and Ku70. The
combined index of PPP1CC and BRCC3 expression was an effective predictor of radioresistance in patients with NPC. The specificity of antibodies is crucial in research, yet the PPP1CC
antibody’s specificity for staining remains controversial. Therefore, we referred to it as a PPP1C antibody for staining purposes. Additionally, the antibody’s utility was demonstrated in
the western blot assay, where the efficiency was assessed in NPC cells transfected with sh_PPP1CC_ (Fig. S2). Notably, PPP1CC occupies a crucial central position in promoting radioresistance
by the BRCC3-PPP1CC-Ku70 axis. PPP1CC is highly expressed not only at the protein level (CPTAC database), but also at the mRNA level (TCGA and GSE databases) in various tumors, suggesting
that the increase of PPP1CC mRNA also reflects the poor prognosis of patients to a certain extent. We suspect that the mechanism involves the elevation of PPP1CC mRNA levels, which is the
basis for promoting radioresistance, and requires the synergistic help of BRCC3. If only the mRNA of PPP1CC with high expression is not assisted by BRCC3, the PPP1CC protein would be
degraded after transcription and translation. Overall, PPP1CC protein was stabilized and elevated by deubiquitination of BRCC3, indicating that PPP1CC and BRCC3 plays an essential role in
promoting DNA repair and radioresistance. In conclusion, our results showed that PPP1CC bound to Ku70/Ku80 heterodimer and activates DNA-PKcs by enhancing the formation of DNA-PK holoenzyme,
thereby promoting the NHEJ pathway, which ultimately leads to radioresistance. This effect is regulated by BRCC3-mediated deubiquitination of the PPP1CC protein leading to the stabilization
of the DNA repair complex and radioresistance, suggesting that the BRCC3/PPP1CC/Ku70 pathway could be a potential therapeutic target. METHODS AND MATERIALS PATIENTS AND TUMOR SAMPLES A
total of 27 NPC tissues in Fig. 1C were collected from November 2011 to April 2018. The clinical characteristics of these patients are shown in Table S1. Paraffin-embedded tissues of 95 NPC
patients with various clinical features and follow-up data (Figs. 1D, 1E and Fig. 7) were gathered at Sun Yat-sen University Cancer Center (China) from October 2011 to August 2022. None of
the patients were treated for their tumors before the biopsy. Tables S2 and S4 list the clinicopathological parameters. CELL CULTURE Keratinocyte serum-free media (supplemented with bovine
pituitary extract) was used to cultivate the immortalized normal human nasopharyngeal epithelial cell line NP69. The NPC cell lines 6-10B, CNE2, S18, SUNE1, CNE1, HONE1, HNE1, S26, HK1, and
5-8 F were cultivated in RPMI-1640 (Sigma‒Aldrich, USA) containing 10% (w/v) fetal bovine serum (FBS, Excell Bio, China). C666 cell lines were cultured in RPMI-1640 containing 20% (w/v) FBS.
HEK293T cells were grown in DMEM (Corning, USA) supplemented with 10% (w/v) FBS. Cells were cultured in a 37 °C humidified incubator containing 5% CO2. To authenticate all cell lines used
in this work, morphological observations and tests for the lack of mycoplasma contamination were regularly conducted. PLASMID CONSTRUCTION AND TRANSFECTION The plasmids pCDH-CMV-_PPP1CC_
(NM_002710)-HA-EF1-Puro, pCDH-CMV-_PPP1CC_ (NM_002710)-Myc-EF1-Puro, pCDH-CMV-_PPP1CC_ (NM_002710)- 3*Flag-EF1-Puro, pCDH-CMV-_PPP1CC_ (NM_002710)-HA-MCS-EF1-G418, pCDH-CMV-_Ku70_
(NM_001469.5)-HA-EF1-Puro, pCDH-CMV-_BRCC3_ (NM_024332.4)-HA-EF1-Puro, pCDH-CMV-_BRCC3_ (NM_024332.4 WT)-3*Flag-EF1-Puro, pCDH-CMV-_BRCC3_ (NM_024332.4 E33A)-3*Flag-EF1-Puro,
pCDH-CMV-_PPP1CC_ (NM_001244974.1, K6R)-3*Flag-EF1-Puro, pCDH-CMV-_PPP1CC_ (NM_001244974.1, K98R)-3*Flag-EF1-Puro, pCDH-CMV-_PPP1CC_ (NM_001244974.1, K234R)-3*Flag-EF1-Puro,
pCDH-CMV-_PPP1CC_ (NM_001244974.1, K260R)-3*Flag-EF1-Puro, plko-u6-shRNA#1/2 (_PPP1CC_, human) -puro, and pTSB-SH-Fluor-2A-ARGs-_shBRCC3_ were purchased from Kidan Bioscience (China).
Professor Liu Na (Sun Yat-sen University Cancer Center, China) provided the plasmids pCMV-kana-Ub (WT)-HA and PRK-HA-Ub (_K48O or K63O_. Table S5 lists the _shRNA_ sequences. The
overexpression plasmids and sh_RNA_ were transiently transfected in cells with Lipofectamine 3000 (Invitrogen, USA), and harvested 24–48 h later. To achieve stable transfection into NPC
cells, HEK293T cells were cotransfected with the lentivirus packaging plasmids pMD2.G and pSPAX2 using Lipofectamine 3000, and the viral supernatant was collected 48 h later. After the viral
supernatant infected NPC cells, the cells were continuously screened by puromycin (1 g/mL, Sigma‒Aldrich, USA) or G418 (400 µg/mL, Beyotime Biotechnology, China) for 7 days, and used for
RT‒qPCR or western blotting. EXTRACTION OF RNA AND RT‒QPCR TRIzol reagent (Invitrogen, USA) was employed to extract RNA. The reverse transcription PCR kit (TaKaRa, Japan) was used for
complementary DNA synthesis. Quantitative RT‒PCR was conducted using the SYBR Green PCR Kit (TaKaRa, Japan) in a 384-well plate and an ABI PRISM 7900 Sequence Detector (Roche 480,
Switzerland). Using GAPDH as control, the relative gene expression was measured using 2−ΔΔCT method. Table S5 lists the primers utilized in this study. WESTERN BLOTTING When extracting
proteins from the cells, sonication on ice was applied following adding lysate. The protein was isolated and transferred to PVDF membranes (Millipore, USA) using sodium dodecyl
sulfate‒polyacrylamide gel electrophoresis (GenScript, USA). Then, 5% (w/v) bovine serum albumin (Sigma‒Aldrich, USA) was used to block the membranes and incubated with primary antibodies
overnight. The next day, secondary antibodies linked to HRP were added to the membranes. An improved chemiluminescence system (GE Healthcare) was used to identify the target proteins, with
β-actin as a loading control. Table S6 lists the antibodies utilized. CELL PROLIFERATION ASSAY In 96-well plates, 2000 cells per well were laid out and exposed to irradiation or not. 10 μL
Cell Counting Kit-8 reagent (TargetMol, USA) was applied to every well on days 0, 1, 2, 3, and 4. A spectrophotometer was applied to measure the absorbance at 450 nm after incubation at 37
°C for 2 h. CLONOGENIC ASSAY Cells were inoculated into 6 well plates (200–10,000 cells/well), and irradiated with 0–8 Gy after cell adhesion. The plates were washed with phosphate-buffered
saline (PBS, Gibco, USA), fixed with 4% paraformaldehyde (Biosharp, China), and stained with crystal violet following colony development (10–14 days). Over 50 cell colonies were counted. The
linear-quadratic formula surviving fractio_n_ = exp (−_αD_ − _βD_2) was applied to fit cell survival curves. TUMOR MIGRATION The tumor migration experiment was performed with 8 mm culture
inserts (Corning, USA). The FBS-free medium was used to seed the tumor cells into the top inserts; 10% FBS RPMI 1640 medium was placed into the lower chambers. The cells that moved across
the membrane were labeled and counted after 24 or 48 h of incubation. The images were captured by the advanced research microscope (NIKON ECLIPSE 80i, Japan). MASS SPECTROMETRY AND CO-IP
Cells were cracked with a sonicator on ice in immunoprecipitation (IP) lysis buffer. A 3 μg of antibody was used to immunoprecipitate protein overnight at 4 °C. The immune complexes with
pierce TM protein A/G magnetic beads (Thermo Scientific, USA) were washed with IP wash buffer. SDS‒PAGE was used to separate the immune complexes, and a Fast Silver Stain Kit for staining
(Beyotime, China). At Huijun Biotechnology (China), mass spectrometry was performed. Western blotting identified the interested proteins in the Co-IP. Table S6 lists the antibodies utilized.
GST PULL-DOWN ASSAY A total of 500 μL (250 μg) of bacteria expressing GST or GST-PPP1CC (DIA-AN, C3325) were bound to 40 μL of glutathione Sepharose 4B beads (GE Healthcare, #17-0756-01),
the complex was mixed with HK1 whole cell lysate at 4 °C overnight. After incubation, the complexes were washed 3 times, denatured at 95 °C for 10 min and analyzed using western blot with
GST, BRCC3, and Ku70 antibodies. IMMUNOFLUORESCENCE AND CONFOCAL MICROSCOPY The fixed buffer is composed of methanol and acetone at a 1:1 concentration and pre-cooled at −20 °C. After
transfected with the corresponding plasmid for 24 h, the cells were placed in the Milli EZSlide 8-well Glass Slide (Merk millipore, USA), and irradiated when the cells were attached 12 h
later. The slides were collected at the indicated time, washed with PBS 3 times, and then placed in the fixed buffer at −20 °C overnight. The staining was conducted on the second day. The
slides with cells were washed 3 times with TBS, sealed with 5%(w/v) BSA for 30 min, and incubated with the primary antibodies at 4 °C overnight. The corresponding fluorescent secondary
antibodies were added for 60 min at room temperature, and the slides were restained with 4′,6-diamidino-2-phenylindole staining (DAPI, Sigma‒Aldrich, USA). To detect the co-localization in
NPC cells, the protocol was repeated to stain the second primary antibody. A laser confocal scanning microscope (LSM880 with Fast Airyscan, Germany) was applied to obtain fluorescence
images. Table S6 lists the antibodies for immunofluorescence analysis. MG132, CQ, AND CHX TREATMENTS The indicated cells were exposed to either 10 μM MG132 (Sigma‒Aldrich, USA) or 50 μM CQ
(Sigma‒Aldrich, USA) for 6 h. To limit protein synthesis, 100 μg/mL CHX (Sigma‒Aldrich, USA) was added to the cells for 0, 4, 8, and 12 h. ASSAY FOR DENATURE-IP The ubiquitin assays were
conducted under denaturation conditions. Denature-IP experiments conducted previously [64, 65]. The cells were lysed on ice with IP lysis buffer and the protease inhibitor cocktail (Roche,
Switzerland) at 24 h after transfection. Cell lysates were immunoprecipitated using the procedure described for the Co-IP with particular antibodies at 95 °C denaturation after exposure for
10 min to 1% (w/v) SDS. Western blotting analysis of immune complexes lysates was carried out. COMET ASSAY The cells were subjected to irradiation (6 Gy), and harvested at 0, 0.5, 4, and 24
h. Using the SCGE DNA Damage Detection Kit (KeyGen, China), neutral comet experiments were applied (in which cells were treated with neutral electrophoresis), and propidium iodide (PI) for
staining. The comet photos were obtained using an inverted fluorescence microscope (OLYMPUS IX73, Japan) at a magnification of ×20. CaspLab-Comet Assay software was used to quantify the tail
moments; a score from 10 cells was calculated for each sample. NHEJ AND HR REPORTER ASSAY For NHEJ and HR reporter assays [18], GFP-HR and GFP-NHEJ reporters were stably transfected into
NPC cells. G418 (0.5 mg/mL; Beyotime) screened cells for 10 days, no GFP was generated at this time. The required plasmids (indicated _PPPCC_ or sh_RNAs_) and pCMV-NLS-_I-SceI_ were
co-transfected into the cells to produce DSBs. If HR or NHEJ repair occurs, GFP is expressed positively. The increase or decrease of GFP+ represents the level of DSB repair activity. The
cells were extracted 48 h later and the proportion of GFP+ cells was measured using flow cytometry (Beckman Coulter, China). The efficiency of HR or NHEJ-mediated DNA repair (HR or NHEJ
activity) was represented by the fraction of GFP+ cells normalized to the control. NPC XENOGRAFT GROWTH IN MICE BALB/c nude mice obtained from Gempharmatech-GD/GDMLAC (Guangdong, China) were
6–8 weeks-old female (_n_ = 24), and maintained in barrier facilities at 18–22 °C, 50–60% relative humidity and a 12-h light/dark cycle. Mice were randomly injected subcutaneously with 3 ×
105 indicated HK1 cells. The tumor volume was measured every 3 days starting on day 7 post-injection. Mice were irradiated locally with or without 6 Gy when the diameter of the tumors
reached 5 mm. The formula used to obtain the tumor volume was length × width2 × 0.5. All research protocols adhered to the Declaration of Helsinki and were approved by Sun Yat-sen
University’s Institutional Animal Care and Use Committee. Animal pain and distress were reduced to the maximum extent. The maximum tumor diameter allowed by our ethics committee was 20 mm,
which was not exceeded in our trial. IMMUNOHISTOCHEMISTRY (IHC) After the sections were deparaffinized, the antigenic repair was performed with basic (EDTA, pH 9.0) antigen retrieval buffer
(Panovue, China) in the microwave, then washed twice with TBS, pre-incubated with hydrogen peroxide, blocked with goat serum (Beyotime, China), and incubated with primary antibodies for 4 °C
overnight. On the following day, the sections were rewarmed and washed with TBS 3 times. According to the instructions of the Dako REAL™ EnVision™ Detection System kit (Dako, Denmark),
rabbit/mouse secondary antibody was labeled for 30 min, followed by DAB staining and hematoxylin restaining. All sections were scored by two experienced pathologists based on the Immune
Response Score (IRS) system [66]. The staining intensity scores were as follows: 0 (no staining), 1 (weak staining), 2 (moderate staining) and 3 (severe staining). The percentage scores of
positive tumor cells were as follows: 0 (<6%), 1(6–25%), 2(26–50%), 3(51–75%) and 4(>75%). Histochemistry (H)-scores (value 0-12) were calculated as the product of the staining
intensity score and the percentage score of positive cells [49]. We adopted median as the criterion for determining a high or low expression of PPP1CC, BRCC3 and Ku70. H-score of PPP1CC
equal to or lower than 6 was defined as low expression, and the score higher than 6 was deemed high expression (Fig. 1D). The H-scores of BRCC3 and Ku70 were 8 and 6, respectively, as the
standard for high and low expression (Fig. 7C, S16, S17). Images were obtained with a digital pathology section scanner (KF-PRO-020, China). The antibodies used in the IHC analysis are
listed in Table S6. STATISTICS AND REPRODUCIBILITY Statistical analyses were employed using GraphPad Prism version 9.0 and SPSS version 22.0 statistical software. Nonparametric tests were
performed when the variance was different. The unpaired or paired two-tailed Student’s _t_ test or the χ2 test was carried out for comparison between groups. Survival curves were drawn using
Kaplan−Meier method. The Spearman method evaluated the correlation strength. Experiments conducted independently in triplicate. _p_ < 0.05 represented a significant difference, and data
are expressed as the mea_n_ ± SD. DATA AVAILABILITY The data used in this study for protein expression are available in the Clinical Proteome Tumor Analysis Consortium (CPTAC;
https://hupo.org/Clinical-Proteome-Tumor-Analysis-Consortium-(CPTAC)). The microarray data used in this study are available in the Gene Expression Omnibus (GEO;
http://www.ncbi.nlm.nih.gov/geo/) under accession codes GSE12452 and GSE61218. The data used in this study for gene expression profiling interactive analysis (GEPIA;
http://gepia.cancer-pku.cn/index.html) are available in The Cancer Genome Atlas (TCGA; https://tcga-data.nci.nih.gov/). The datasets supporting the conclusions of this article are included
within the article and its supplementary information files. All original western blots are provided as supplementary material. Data that support the findings of this study are available from
the corresponding author upon reasonable request. REFERENCES * Kockler ZW, Osia B, Lee R, Musmaker K, Malkova A. Repair of DNA breaks by break-induced replication. Annu Rev Biochem.
2021;90:165–91. Article CAS PubMed PubMed Central Google Scholar * Groelly FJ, Fawkes M, Dagg RA, Blackford AN, Tarsounas M. Targeting DNA damage response pathways in cancer. Nat Rev
Cancer. 2023;23:78–94. Article CAS PubMed Google Scholar * Awwad SW, Serrano-Benitez A, Thomas JC, Gupta V, Jackson SP. Revolutionizing DNA repair research and cancer therapy with
CRISPR-Cas screens. Nat Rev Mol Cell Biol. 2023;24:477–94. Article CAS PubMed Google Scholar * Pilie PG, Tang C, Mills GB, Yap TA. State-of-the-art strategies for targeting the DNA
damage response in cancer. Nat Rev Clin Oncol. 2019;16:81–104. Article CAS PubMed PubMed Central Google Scholar * Xu Y, et al. The Largest Chinese Cohort Study indicates homologous
recombination pathway gene mutations as another major genetic risk factor for colorectal cancer with heterogeneous clinical phenotypes. Research. 2023;6:0249. Article CAS PubMed PubMed
Central Google Scholar * Murmann-Konda T, Soni A, Stuschke M, Iliakis G. Analysis of chromatid-break-repair detects a homologous recombination to non-homologous end-joining switch with
increasing load of DNA double-strand breaks. Mutat Res Gen Toxicol Environ Mutagen. 2021;867:503372. Article CAS Google Scholar * Frank KM, et al. Late embryonic lethality and impaired
V(D)J recombination in mice lacking DNA ligase IV. Nature. 1998;396:173–7. Article CAS PubMed Google Scholar * Gao Y, et al. A critical role for DNA end-joining proteins in both
lymphogenesis and neurogenesis. Cell. 1998;95:891–902. Article CAS PubMed Google Scholar * Woodbine L, Gennery AR, Jeggo PA. Reprint of “The clinical impact of deficiency in DNA
non-homologous end-joining. DNA Repair. 2014;17:9–20. Article CAS PubMed Google Scholar * Stephens PJ, et al. Massive genomic rearrangement acquired in a single catastrophic event during
cancer development. Cell. 2011;144:27–40. Article CAS PubMed PubMed Central Google Scholar * Ghezraoui H, et al. Chromosomal translocations in human cells are generated by canonical
nonhomologous end-joining. Mol Cell. 2014;55:829–42. Article CAS PubMed PubMed Central Google Scholar * Peycheva M, et al. DNA replication timing directly regulates the frequency of
oncogenic chromosomal translocations. Science. 2022;377:1277. Article Google Scholar * Sishc BJ, Davis AJ. The role of the core non-homologous end joining factors in carcinogenesis and
cancer. Cancers. 2017;9:1–30. * Stavridi AK, Appleby R, Liang SK, Blundell TL, Chaplin AK. Druggable binding sites in the multicomponent assemblies that characterise DNA double-strand-break
repair through non-homologous end joining. Essays Biochem. 2020;64:791–806. Article CAS Google Scholar * Chen YK, et al. LRRC31 inhibits DNA repair and sensitizes breast cancer brain
metastasis to radiation therapy. Nature Cell Biology. 2020;22:1276. Article CAS PubMed PubMed Central Google Scholar * Chen Y, et al. USP44 regulates irradiation-induced DNA
double-strand break repair and suppresses tumorigenesis in nasopharyngeal carcinoma. Nature Communications. 2022;13:501. Article CAS PubMed PubMed Central Google Scholar * Liao JB, et
al. Methyltransferase 1 is required for nonhomologous end-joining repair and renders hepatocellular carcinoma resistant to radiotherapy. Hepatology. 2023;77:1896–1910. * Wang XC, et al.
Genome-wide RNAi screening identifies RFC4 as a factor that mediates radioresistance in colorectal cancer by facilitating nonhomologous end joining repair. Clin Cancer Res. 2019;25:4567–79.
Article CAS PubMed Google Scholar * Zhou W, et al. GTP signaling links metabolism, DNA repair, and responses to genotoxic stress. bioRxiv. 2024;14:158–76. * Réthi-Nagy Z, Abrahám E,
Sinka R, Juhász S, Lipinszki Z. Protein phosphatase 4 is required for centrobin function in DNA damage repair. Cells-Basel. 2023;12:2219. Article Google Scholar * Lee DH, et al. A PP4
phosphatase complex dephosphorylates RPA2 to facilitate DNA repair via homologous recombination. Nat Struct Mol Biol. 2010;17:365–U138. Article CAS PubMed PubMed Central Google Scholar
* Ambjorn SM, et al. A complex of BRCA2 and PP2A-B56 is required for DNA repair by homologous recombination. Nat Commun. 2021;12:5748. Article PubMed PubMed Central Google Scholar * Xie
WJ, et al. Comprehensive analysis of PPPCs family reveals the clinical significance of PPP1CA and PPP4C in breast cancer. Bioengineered. 2022;13:190–205. Article CAS PubMed Google Scholar
* Liu JP, et al. Protein phosphatase PP4 is involved in NHEJ-mediated repair of DNA double-strand breaks. Cell Cycle. 2012;11:2643–9. Article CAS PubMed Google Scholar * Douglas P, et
al. Protein phosphatase 6 interacts with the DNA-dependent protein kinase catalytic subunit and dephosphorylates gamma-H2AX. Mol Cell Biol. 2010;30:1368–81. Article CAS PubMed PubMed
Central Google Scholar * Yu YM, Pace SM, Allen SR, Deng CX, Hsu LC. A PP1-binding motif present in BRCA1 plays a role in its DNA repair function. Int J Biol Sci. 2008;4:352–61. Article
CAS PubMed PubMed Central Google Scholar * Hsu LC. Identification and functional characterization of a PP1-binding site in BRCA1. Biochem Biophys Res Commun. 2007;360:507–12. Article
CAS PubMed PubMed Central Google Scholar * Liu Y, Virshup DM, White RL, Hsu LC. Regulation of BRCA1 phosphorylation by interaction with protein phosphatase 1α. Cancer Res.
2002;62:6357–61. CAS PubMed Google Scholar * Scully R, et al. Association of BRCA1 with Rad51 in mitotic and meiotic cells. Cell. 1997;88:265–75. Article CAS PubMed Google Scholar *
Scully R, et al. Dynamic changes of BRCA1 subnuclear location and phosphorylation state are initiated by DNA damage. Cell. 1997;90:425–435. Article CAS PubMed Google Scholar * Zhong Q,
et al. Association of BRCA1 with the hRad50-hMre11-p95 complex and the DNA damage response. Science. 1999;285:747–50. Article CAS PubMed Google Scholar * Deng CX, Wang RH. Roles of BRCA1
in DNA damage repair: a link between development and cancer. Hum Mol Genet. 2003;12:R113–23. Article CAS PubMed Google Scholar * Hartman AR, Ford JM. BRCA1 induces DNA damage
recognition factors and enhances nucleotide excision repair. Nat Genet. 2002;32:180–4. Article CAS PubMed Google Scholar * Zhu SL, Fisher LA, Bessho T, Peng AM. Protein phosphatase 1 and
phosphatase 1 nuclear targeting subunit-dependent regulation of DNA-dependent protein kinase and non-homologous end joining. Nucleic Acids Res. 2017;45:10583–94. Article CAS PubMed
PubMed Central Google Scholar * Tu ZW, et al. BRCC3 acts as a prognostic marker in nasopharyngeal carcinoma patients treated with radiotherapy and mediates radiation resistance in vitro.
Radiat Oncol. 2015;10:123. Article PubMed PubMed Central Google Scholar * Lee AWM, Ma BBY, Ng WT, Chan ATC. Management of nasopharyngeal carcinoma: current practice and future
perspective. J Clin Oncol. 2015;33:3356. Article PubMed Google Scholar * Wong KCW, et al. Nasopharyngeal carcinoma: an evolving paradigm. Nat Rev Clin Oncol. 2021;18:679–95. Article CAS
PubMed Google Scholar * Qiao H, et al. Association of intratumoral microbiota with prognosis in patients with nasopharyngeal carcinoma from 2 hospitals in China. JAMA Oncol.
2022;8:1301–9. Article PubMed PubMed Central Google Scholar * Zhang PP, et al. Protein C receptor maintains cancer stem cell properties via activating lipid synthesis in nasopharyngeal
carcinoma. Signal Transduct Targeted Ther. 2022;7:46. Article CAS Google Scholar * Li JY, et al. TRIM21 inhibits irradiation-induced mitochondrial DNA release and impairs antitumour
immunity in nasopharyngeal carcinoma tumour models. Nat Commun. 2023;14:865. Article CAS PubMed PubMed Central Google Scholar * Jackson L, et al. Influence of family history on
penetrance of hereditary cancers in a population setting. Eclinicalmedicine. 2023;64:102159. Article PubMed PubMed Central Google Scholar * Ayoub N, Jeyasekharan AD, Bernal JA,
Venkitaraman AR. HP1-beta mobilization promotes chromatin changes that initiate the DNA damage response. Nature. 2008;453:682–6. Article CAS PubMed Google Scholar * Patil A, Nakai K,
Nakamura H. HitPredict: a database of quality assessed protein-protein interactions in nine species. Nucleic Acids Res. 2011;39:D744–9. Article CAS PubMed Google Scholar * Yu YL, Yu J,
Ge SF, Su Y, Fan XQ. Novel insight into metabolic reprogrammming in cancer radioresistance: a promising therapeutic target in radiotherapy. Int J Biol Sci. 2023;19:811–28. Article CAS
PubMed PubMed Central Google Scholar * Jiang K, et al. STC2 activates PRMT5 to induce radioresistance through DNA damage repair and ferroptosis pathways in esophageal squamous cell
carcinoma. Redox Biol. 2023;60:102626. Article CAS PubMed PubMed Central Google Scholar * Price JM, Prabhakaran A, West CML. Predicting tumour radiosensitivity to deliver precision
radiotherapy. Nat Rev Clin Oncol. 2023;20:83–98. Article CAS PubMed Google Scholar * Wu Y, Song Y, Wang R, Wang T. Molecular mechanisms of tumor resistance to radiotherapy. Mol Cancer.
2023;22:96. Article CAS PubMed PubMed Central Google Scholar * Chen QP, et al. SOCS2-enhanced ubiquitination of SLC7A11 promotes ferroptosis and radiosensitization in hepatocellular
carcinoma. Cell Death Differ. 2023;30:137–51. Article CAS PubMed Google Scholar * Jie XH, et al. USP9X-mediated KDM4C deubiquitination promotes lung cancer radioresistance by
epigenetically inducing TGF-beta 2 transcription. Cell Death Differ. 2021;28:2095–111. Article CAS PubMed PubMed Central Google Scholar * Zetrini AE, et al. Remodeling tumor immune
microenvironment by using polymer-lipid-manganese dioxide nanoparticles with radiation therapy to boost immune response of castration-resistant prostate cancer. Research. 2023;6:0247.
Article CAS PubMed PubMed Central Google Scholar * Nambiar DK, Mishra D, Singh RP. Targeting DNA repair for cancer treatment: lessons from PARP inhibitor trials. Oncol Res.
2023;31:405–21. Article PubMed PubMed Central Google Scholar * Wan CH, Wu MM, Zhang SS, Chen YY, Lu CH. alpha 7nAChR-mediated recruitment of PP1 gamma promotes TRAF6/NF-kappa B cascade
to facilitate the progression of hepatocellular carcinoma. Mol Carcinogen. 2018;57:1626–39. Article CAS Google Scholar * Li CS, et al. Overexpression of protein phosphatase 1 gamma (PP1
gamma) is associated with enhanced cell proliferation and poor prognosis in hepatocellular carcinoma. Digest Dis Sci. 2017;62:133–42. Article CAS PubMed Google Scholar * Sogawa K, et al.
Enhanced expression of PP1 gamma 1, a catalytic subunit isoform of protein phosphatase type 1, in invasive ductal carcinoma of the breast. Cancer Lett. 1997;112:263–8. Article CAS PubMed
Google Scholar * Sogawa K, et al. Enhanced expression of catalytic subunit isoform PP1 gamma 1 of protein phosphatase type 1 associated with malignancy of osteogenic tumor. Cancer Lett.
1995;89:1–6. CAS PubMed Google Scholar * Kou Y, Zhang S, Chen X, Hu S. Gene expression profile analysis of colorectal cancer to investigate potential mechanisms using bioinformatics. Onco
Targets Ther. 2015;8:745–52. PubMed PubMed Central Google Scholar * Malone D, Lardelli RM, Li MQ, David M. Dephosphorylation activates the interferon-stimulated Schlafen family member 11
in the DNA damage response. J Biol Chem. 2019;294:14674–85. Article PubMed PubMed Central Google Scholar * Dobbs TA, Tainer JA, Lees-Miller SP. A structural model for regulation of NHEJ
by DNA-PKcs autophosphorylation. DNA Repair. 2010;9:1307–14. Article CAS PubMed PubMed Central Google Scholar * Hammel M, et al. Ku and DNA-dependent protein kinase dynamic
conformations and assembly regulate DNA binding and the initial non-homologous end joining complex. J Biol Chem. 2010;285:1414–23. Article PubMed Google Scholar * Dong YS, et al.
Regulation of BRCC, a holoenzyme complex containing BRCA1 and BRCA2, by a signalosome-like subunit and its role in DNA repair. Mol Cell. 2003;12:1087–1099. Article CAS PubMed Google
Scholar * Chen XW, Arciero CA, Wang CR, Broccoli D, Godwin AK. BRCC36 is essential for ionizing radiation-induced BRCA1 phosphorylation and nuclear foci formation. Cancer Res.
2006;66:5039–46. Article CAS PubMed Google Scholar * Tao H, et al. BRCC3 promotes tumorigenesis of bladder cancer by activating the NF-kappaB signaling pathway through targeting TRAF2.
Front Cell Dev Biol. 2021;9:720349. Article PubMed PubMed Central Google Scholar * Hu Y, Zhang Y, Ding M, Xu R. Long noncoding RNA TMPO-AS1/miR-126-5p/BRCC3 axis accelerates gastric
cancer progression and angiogenesis via activating PI3K/Akt/mTOR pathway. J Gastroenterol Hepatol. 2021;36:1877–88. Article CAS PubMed Google Scholar * Zhao Y, et al. Hypermethylation of
UCHL1 promotes metastasis of nasopharyngeal carcinoma by suppressing degradation of cortactin (CTTN). Cells. 2020;9:559. Article CAS PubMed PubMed Central Google Scholar * Zhao Y, et
al. USP2a supports metastasis by tuning TGF-beta signaling. Cell Rep. 2018;22:2442–54. Article CAS PubMed Google Scholar * Liu N, et al. Reduced expression of Dicer11 is associated with
poor prognosis in patients with nasopharyngeal carcinoma. Med Oncol. 2013;30:360. Article CAS PubMed Google Scholar Download references ACKNOWLEDGEMENTS The authors thank Prof. M. Zeng,
Prof. and Prof. H. Mai (Sun Yat-sen University Cancer Center, China) for human NPC cells, plasmids and technical advice. Professor Liu Ranyi (Sun Yat-sen University Cancer Center, China)
kindly contributed reporter constructs for HR, NHEJ, and the I-SceI expression plasmid pCMV-NLS-_I-SceI_. This work was sponsored by the National Natural Science Foundation of China
(81872464), Science and Technology Program of Guangdong Province, China (2021A0505110010), and National High-tech R&D Program of China (863 Program) (2006AA02Z4B4). FUNDING This work was
sponsored by the National Natural Science Foundation of China (81872464), Science and Technology Program of Guangdong Province, China (2021A0505110010 and 010192200059), and National
High-tech R&D Program of China (863 Program) (2006AA02Z4B4). AUTHOR INFORMATION Author notes * These authors contributed equally: Ping Feng, Ying Wang. AUTHORS AND AFFILIATIONS * State
Key Laboratory of Oncology in South China; Guangdong Key Laboratory of Nasopharyngeal Carcinoma Diagnosis and Therapy; Guangdong Provincial Clinical Research Center for Cancer, Sun Yat-sen
University Cancer Center, Guangzhou, 510060, China Ping Feng, Ying Wang, Na Liu, Yujun Hu, Zilu Huang, Ya Liu, Shuohan Zheng, Tongchao Jiang, Xiang Xiao, Peng Huang & Yunfei Xia *
Department of Radiation Oncology, Sun Yat-sen University Cancer Center, Guangzhou, 510060, China Ping Feng, Ying Wang, Na Liu, Yujun Hu, Zilu Huang, Ya Liu, Shuohan Zheng, Tongchao Jiang,
Xiang Xiao & Yunfei Xia * The Second Affiliated Hospital, Hengyang Medical School, University of South China, Hengyang, 421001, Hunan, China Yanming Chen * Department of Clinical
Oncology, University of Hong Kong, Hong Kong (SAR), China Wei Dai * University of Hong Kong-Shenzhen Hospital, Shenzhen, China Wei Dai * Metabolic Innovation Center, Sun Yat-sen University,
Guangzhou, 510060, China Peng Huang Authors * Ping Feng View author publications You can also search for this author inPubMed Google Scholar * Ying Wang View author publications You can also
search for this author inPubMed Google Scholar * Na Liu View author publications You can also search for this author inPubMed Google Scholar * Yanming Chen View author publications You can
also search for this author inPubMed Google Scholar * Yujun Hu View author publications You can also search for this author inPubMed Google Scholar * Zilu Huang View author publications You
can also search for this author inPubMed Google Scholar * Ya Liu View author publications You can also search for this author inPubMed Google Scholar * Shuohan Zheng View author publications
You can also search for this author inPubMed Google Scholar * Tongchao Jiang View author publications You can also search for this author inPubMed Google Scholar * Xiang Xiao View author
publications You can also search for this author inPubMed Google Scholar * Wei Dai View author publications You can also search for this author inPubMed Google Scholar * Peng Huang View
author publications You can also search for this author inPubMed Google Scholar * Yunfei Xia View author publications You can also search for this author inPubMed Google Scholar
CONTRIBUTIONS PF, YFX, and YW designed, carried out and analyzed most of the experiments. NL checked the Figures, controlled the experiment steps, and was involved in discussions of the
data. YMC provided helped with pathological analysis. YJH, SHZ, and YL helped organize and analyze clinical information and follow-up data. XX, ZLH, and TCJ contributed to several
experiments. WD touched up the manuscript. PF wrote the manuscript with input from all other authors. PH and YFX conceived the study, provided scientific directions, and established
collaborations. All authors read and approved the final paper. CORRESPONDING AUTHORS Correspondence to Peng Huang or Yunfei Xia. ETHICS DECLARATIONS COMPETING INTERESTS The authors declare
no competing interests. ETHICS APPROVAL The study was approved by the Sun Yat-sen University Cancer Center’s Institutional Ethical Review Board (GZR2018-100), and the informed consent was
obtained from all patients. Animal experiments were approved by the Animal Research Ethics Committee of Sun Yat-sen University Cancer Center and complied with the relevant ethical guidelines
(L102012020120M). All research protocols adhered to the Declaration of Helsinki. ADDITIONAL INFORMATION PUBLISHER’S NOTE Springer Nature remains neutral with regard to jurisdictional claims
in published maps and institutional affiliations. SUPPLEMENTARY INFORMATION SUPPLEMENTAL INFORMATION ORIGINAL WESTERN BLOTS RIGHTS AND PERMISSIONS Springer Nature or its licensor (e.g. a
society or other partner) holds exclusive rights to this article under a publishing agreement with the author(s) or other rightsholder(s); author self-archiving of the accepted manuscript
version of this article is solely governed by the terms of such publishing agreement and applicable law. Reprints and permissions ABOUT THIS ARTICLE CITE THIS ARTICLE Feng, P., Wang, Y.,
Liu, N. _et al._ High expression of PPP1CC promotes NHEJ-mediated DNA repair leading to radioresistance and poor prognosis in nasopharyngeal carcinoma. _Cell Death Differ_ 31, 683–696
(2024). https://doi.org/10.1038/s41418-024-01287-5 Download citation * Received: 08 August 2023 * Revised: 19 March 2024 * Accepted: 20 March 2024 * Published: 08 April 2024 * Issue Date:
May 2024 * DOI: https://doi.org/10.1038/s41418-024-01287-5 SHARE THIS ARTICLE Anyone you share the following link with will be able to read this content: Get shareable link Sorry, a
shareable link is not currently available for this article. Copy to clipboard Provided by the Springer Nature SharedIt content-sharing initiative