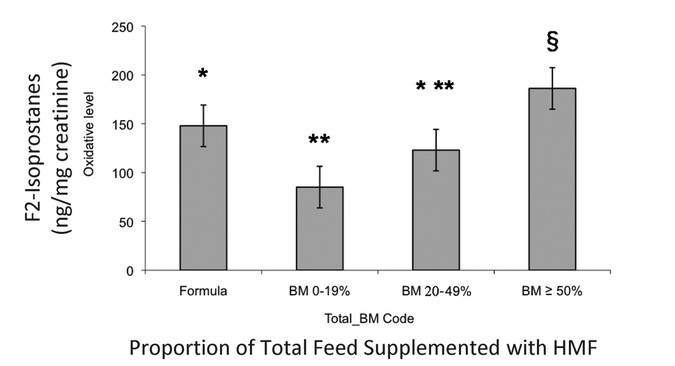
- Select a language for the TTS:
- UK English Female
- UK English Male
- US English Female
- US English Male
- Australian Female
- Australian Male
- Language selected: (auto detect) - EN
Play all audios:
ABSTRACT Morbidity in the premature (PT) infant may reflect difficult adaptation to oxygen. We hypothesized that feeding including formula feeding (F) and feeding mother's milk (HM)
with added fortifier would affect redox status. Therefore, 65 PT infants (birth weight: 1146 ± 261 g; GA: 29 ± 2.5 wk; mean ± SD) were followed biweekly, once oral feeds were introduced.
Feeding groups: F (>75% total feeds) and HM (>75% total feeds) were further subdivided according to human milk fortifier (HMF) content of 0–19, 20–49, and ≥50%. Oxidative stress was
quantified by F2-isoprostanes (F2-IsoPs) in urine, protein carbonyls, and oxygen radical absorbance capacity (ORAC) in plasma. F2-IsoPs (ng/mg creatinine): 0–2 wk, 125 ± 63; 3–4 wk, 191 ±
171; 5–6 wk, 172 ± 83; 7–8 wk, 211 ± 149; 9–10 wk, 222 ± 121; and >10 wk, 183 ± 67. Protein carbonyls from highest [2.41 ± 0.75 (_n_ = 9)] and lowest [2.25 ± 0.89 (_n_ = 12) pmol/μg
protein] isoprostane groups did not differ. ORAC: baseline, 6778 ± 1093; discharge, 6639 ± 735 [full term 4 and 12 M, 9010 ± 600 mg (_n_ = 12) TE]. Highest isoprostane values occurred in
infants with >50% of their mother's milk fortified. Further research on HMF is warranted. SIMILAR CONTENT BEING VIEWED BY OTHERS PRENATAL OXIDATIVE STRESS AND RAPID INFANT WEIGHT
GAIN Article 03 April 2023 BILE ACIDS PROFILE AND REDOX STATUS IN HEALTHY INFANTS Article 22 October 2022 NEONATAL INTERMITTENT HYPOXIA, FISH OIL, AND/OR ANTIOXIDANT SUPPLEMENTATION ON GUT
MICROBIOTA IN NEONATAL RATS Article 28 August 2021 MAIN Elevated concentrations of reactive oxygen species (ROS) have long been implicated as causal factors in human illness (1,2). Although
a number of enzymatic and nonenzymatic antioxidant defense mechanisms normally maintain an “uneasy truce” between oxidants and reductants, an imbalance can perturb homeostasis and result in
disease. Nowhere is this perhaps more an issue than for the newborn infant. Birth itself may be viewed as a hyperoxic challenge, wherein the fetus transitions from a relatively hypoxic
environment with a arterial oxygen tension of 25–35 mm Hg (3) to the extrauterine environment where arterial oxygen tension reaches 100 mm Hg. Coupled with the oxidative stress induced
through labor and higher-than-adult levels of mitochondrial respiration and peroxide production (4), the neonate may rely on compensatory mechanisms to transition successfully through the
postpartum period such as ROS scavenging ability provided by human milk (5). Previously, we showed that healthy breast-fed infants are under acute oxidative stress in early infancy
reflecting the difficulty in adapting to increased oxygen exposure after birth (5). The challenge seems to be even more acute for the premature (PT) infant, for whom the normal developmental
expression of antioxidant systems is either incomplete or compromised (6,7) and who is often subjected to oxygen therapy and/or feeding strategies that contribute to redox imbalance (8–10).
Accordingly, oxidative stress is proposed to be either causal or at least strongly linked to a number of neonatal disorders, including bronchopulmonary dysplasia (BPD), periventricular
leukomalacia, retinopathy of prematurity, intraventricular hemorrhage (IVH), and necrotizing eneterocolitis (NEC) (11–14). What is clear is that a certain amount of oxidative stress is
necessary to activate specific enzymatic pathways and that ROS act as signaling molecules. The PT infant would be expected to have a more difficult time at birth in the transition from fetal
to neonatal life (2). The extent of the challenge posed by free radicals and ROS for the preterm infant is becoming increasingly evident. Two plasma markers of lipid peroxidation,
F2-isoprostanes (F2-IsoPs) and malonaldehyde, are elevated in very LBW infants with cerebral white matter injury, the latter >10-fold compared with adult controls (15). The significance
of such findings is highlighted by the fact that preterm white matter is selectively vulnerable to oxidative damage (12). More recently, plasma F2-IsoPs have been shown to be elevated in
children who were born preterm (16). The perinatal period may be a time of both increased exposure to and risk from oxidative stress. We have found that human milk _in vitro_ acts as a
better free radical scavenger than does infant formula (5). We hypothesized that PT infants who were breast-fed would show less evidence of oxidative stress after birth than those who were
formula fed. In this report, we present preliminary evidence that dietary factors may contribute to the overall level of oxidative stress in preterm infants. MATERIALS AND METHODS SUBJECTS.
Sixty-five infants with birth weights <1500 g were recruited from the Health Sciences Center and St. Boniface Research Center, Winnipeg, Manitoba, Canada, after birth but before oral
feeds. Eligibility criteria included the following: prematurity (<37 wk GA by date of last menstrual period or early ultrasound) and birth weight <1500 g. Exclusion criteria included
the following: presence of any major congenital anomaly, underlying genetic syndrome, presence of clinical or confirmed diagnosis of congenital infections, or unlikely to comply with
necessary follow-up (_i.e._ out-of-province patients). Ethical approval was obtained from the University of Manitoba Research Ethics Board, and informed consent was obtained from all
participants. For feeding purposes, the breast milk group was defined _a priori_ as having received no <75% of all oral feeds during their entire hospital stay as mother's own milk.
The formula-feeding group was defined as having received no <75% of all feedings in hospital from humanized infant formulas. The mixed-feeding group was defined as anything in between
these two criteria. Given the variable intake of human milk fortifier (HMF) received during hospitalization, the breast milk group was further subdivided according to the total amount of
breast milk fortified with HMF: ≥50, 20–49, and 0–19%. SAMPLE COLLECTION. At study entry and biweekly thereafter, a blood sample was collected by venipuncture or from a catheter inserted
centrally. Whole blood (0.5–0.7 mL) was collected in heparinized microtainers. Plasma was separated, and red blood cells were washed three times in saline before freezing at −80°C until
analysis. Urine samples were collected with a Hollister U-Bag (17), and after aliquoting to 1.5 mL, clear plastic tubes were frozen at −80°C. Data recorded from hospital records included the
following: Apgar scores, SNAP scores (both severity of illness indices), duration and volume of parenteral nutrition, fortification with HMF (Mead Johnson Nutritionals, Evansville, IN);
weight gain; incidence of oxygen exposure at both 28 d and 36 wk postconceptional age was recorded for defining BPD; days of ventilatory support; the worst grade of IVH including
periventricular leukomalacia; incidence with none or either suspected or confirmed NEC (perforation and not perforated), using radiography; retinopathy of prematurity not present or
identified as stage 1 or greater; cranial ultrasonography for IVH-PVH (d 7 and ∼36 wk); infections; not present or present with blood culture-proven sepsis (bacterial or fungal);
cerebrospinal fluid culture proven meningitis; and urine positive result: presumed but not culture-proven sepsis. F2-IsoPs a marker of lipid peroxidation in urine are prostaglandin-like
compounds formed during peroxidation of arachidonic acid (18). The precision of the mass spectrometry assay is ± 5% with 80% accuracy. Normal adult levels in urine are 38.1 ± 19.1 ng/mg
creatinine (18). Creatinine was measured by standard techniques (18). Measurement of this compound in urine has the advantage that there is less likely to be autoxidation of samples when
compared with blood analysis (18). Antioxidant activity of plasma samples at the beginning and end of the study and mother's milk collected every 2 wk was measured using the oxygen
radical absorbance capacity (ORAC) according to procedures described by Prior _et al._ (19). An FLx800 micro plate fluorescence reader (Bio-Tek Instruments, Inc., Winooski, VT) was used with
fluorescence filters for an excitation wavelength of 485/20 nm and an emission wavelength of 528/20 nm. The plate reader was controlled by KC4 3.0 software (version 29). Dilution of sample,
control, and Trolox standard was done manually. The quantity of 300 μL each of buffer solution (blank) and diluted sample solution, control, and Trolox standard was transferred to a 96-well
flat-bottom polystyrene micro plate (Corning Incorporated, Corning, NY) by hand according to their designated positions. A full automation of plate-to-plate liquid transfer was programmed
by using a Precision 2000 micro plate pipetting system (Bio-Tek Instruments, Inc.). Peroxyl radical was generated by AAPH (2,2′-azobis-2-methyl-propanimidamide, dihydrochloride) during
measurement, and fluorescein was used as the substrate. All the reaction mixtures were prepared in the measured plate in duplicate, and at least three independent assays were performed for
each sample. Final ORAC values were calculated by using a regression equation between the Trolox concentration and the net area under the fluorescence decay curve. Area under the curve (AUC)
was calculated as follows: where _f_0 is the initial fluorescence reading at 0 min and _f_i is the fluorescence reading at time in min. The net AUC was obtained by subtracting the AUC of
the blank from that of the sample. ORAC values were expressed as Trolox equivalents by using the standard curve. Final results were calculated and expressed as TE (μmol Trolox equivalents)
of plasma samples. Antioxidant enzymes in red blood cells including superoxide dismutase, catalase, and glutathione peroxidase were analyzed as reported previously (5). Protein carbonyls
were measured in plasma by a dot-blot immunoassay, following dinitrophenyl hydrazine reaction of protein carbonyl groups, using commercially available primary antibody to the resulting
dinitrophenyl (DNP) derivatives [primary rabbit anti-DNP and horseradish peroxidase (HRP)-conjugated secondary goat anti-rabbit], followed by ECL+ and chemiluminescence detection (20).
Plasma samples used for analysis of protein carbonyls were selected from those infants whose urine had the highest and lowest urinary isoprostane values to determine whether carbonyl content
reflected isoprostane values and if further analysis should be done on the entire urine sample collection. Normal plasma protein carbonyl levels in appropriate-for-GA newborns have been
reported as 1.31 ± 0.24 pmol/μg protein (21). DATA ANALYSIS. Data for continuous variables were analyzed using univariate and multivariate repeated measures ANOVA procedures [SAS 9.1 (SAS
Institute, Inc., Cary, NC) and SPSS 16.0 (SPSS, Inc, Chicago, IL)]. Log transformations were done for variables, which were not Gaussian. Significance was assigned to _p_ ≤ 0.05. RESULTS
Subject characteristics are presented in Table 1. Plasma ORAC and red blood cell enzyme values are presented in Table 2; no significant differences were found over time. Subject clinical
characteristics are presented in Table 3. F2-IsoPs values were as follows: 0–2 wk, 125 ± 63; 3–4 wk, 191 ± 171; 5–6 wk, 172 ± 83; 7–8 wk, 211 ± 149; 9–10 wk, 222 ± 121; and >10 wk, 183 ±
67 (ng/mg creatinine). Normal levels from 40 adults: 38.1 ± 19.1 ng/mg creatinine (18). Protein carbonyls from those subjects whose urine had the highest and lowest isoprostane values were
as follows: high group: 2.41 ± 0.75 (_n_ = 9) pmol/μg protein; low group: 2.25 ± 0.89 (_n_ = 12) pmol/μg protein (mean ± SD; _p_ = 0.33, not significant). Plasma ORAC: baseline, 6778 ± 1093;
discharge, 6639 ± 735; [healthy PT 6 mo, 8134 ± 706 (_n_ = 7); full term 4 and 12 mo, 9010 ± 600 mg (_n_ = 12); and adult 9295 ± 887 (_n_ = 6) TE]. Isoprostane values according to
percentage of HMF in total feedings are presented in Fig. 1. F2-IsoPs in the >50% group were significantly greater than the formula-fed group (_p_ = 0.002). F2-IsoPs did not correlate
either in a univariate or multivariate model with either of enzyme assays, anthropometric measurements, total days of O2 intake, GA, Apgar scores, type of feed in previous pregnancies,
morbidity, or plasma or feed ORAC values. F2-IsoPs were related to gender (females > males) and whether or not the infant had surgery for a patent ductus arteriosus (higher for surgery
than no surgery). DISCUSSION We found high levels of F2-IsoPs in urine during early infancy in the PT infant (Fig. 1) compared with normal adult levels of ∼38 ± 19 (_n_ = 40) ng/mg
creatinine (18). These levels persisted to >9 wk after birth, reflecting the stress of prematurity. This was supported by our findings of nearly 2-fold elevated protein carbonyl in the
plasma of these infants compared with appropriate-weight-for-GA full-term newborns [1.31 ± 0.24 pmol/μg protein (21)]. Our results support those of other authors who assessed oxidative
status in the PT infants (22–26). Farkouh _et al._ (22) reported elevated urinary peroxides in PT infants (10 μmol/mmol creatinine) compared with those of full-term infants (5 μmol/mmol
creatinine) within the first 72 h of life. Cervantes-Munguia _et al._ (23) measured serum lipoperoxide levels in PT infants <33 wk gestation every week for the first month of life. Those
infants who eventually developed retinopathy of prematurity (11/50) were more likely to have elevated lipoperoxides than those who did not develop the disease (5.4 ± 1.3 mmol/mL _versus_ 2.9
± 0.9 mmol/mL _p_ = 0.0001). Similar results were found by Buonocore _et al._ (24) in both hypoxic and nonhypoxic PT infants with a normal clinical course at birth and 7 d later. Weinberger
_et al._ (25) reported increasing levels of MDA, a lipid peroxidation product, in urine up to 10 d after birth in PT infants born <30 wk gestation. A recent report of F2-IsoPs in urine
found no correlation with later outcome of BPD (26), whereas Ahola _et al._ (11) did find that plasma F2-IsoPs increased in PT infants who developed BPD. Metabolism of F2-IsoPs is likely
different in urine and plasma and may explain the lack of consistency between studies. Data from this study suggest that the PT infant is under considerable oxidative stress in early life.
Elevated F2-IsoPs were also reported in children aged 8–13 y who had been born small-for-GA (16). In that study, compared with children born appropriate-for-GA, urinary isoprostanes were
significantly elevated (46 ± 4.6 _versus_ 29 ± 3.1 ng/mg creatinine). These findings (16) were supported by increased erythrocyte oxidative products in small-for-GA (287 ± 17) _versus_
appropriate-for-GA infants (216 ± 11 mmol/g Hb). It seems that early oxidative stress in PT infants may persist throughout childhood although other intervening variables may play a role. It
is noteworthy that F2-IsoPs did not decline over the first 3 mo in these PT infants, whereas F2-IsoPs did decline over that time period in the full-term infant (5). The measurement of
F2-IsoPs has emerged as possibly the most reliable approach to assessing oxidative stress _in vivo_ (18,27). PT infants are known to have elevated metabolic rates compared with full-term
infants and adults (4). Oxygen consumption is related to metabolic rate, which is dependent primarily on growth rate. As PT infants grow faster than term infants their metabolic rate will be
higher (28). It is quite likely that the high levels of isoprostanes reflects this elevated metabolism and the possible weakened antioxidant defense mechanisms reported by others (6).
Plasma ORAC results (Table 2) suggest that the PT infant is somewhat compromised compared with full-term infants (9010 ± 600 TE) in early life. ORAC seems to rise over time as Franco _et
al._ (16) found no difference in total antioxidant status between children who had been born PT or full term although there was greater evidence of oxidative stress in the children who had
been born PT. These data are difficult to interpret and present a dilemma because a high value may mean increased ability to resist oxidative stress, whereas a low value may also mean that
the infant is being protected. Prior _et al._ (19) have shown that ORAC is sensitive to dietary intake of antioxidants. However, a low ORAC value has not been linked to increased risk of
disease. Furthermore, in this study, we did not find any relationship between ORAC and lipid peroxidation or any difference in peroxidation between infants who were breast-fed or formula
fed. Subtle differences according to feeding may take longer to manifest even though breast milk and breast feeding have been shown to be protective to the PT infant (29–32). It should be
mentioned that not all subjects were able to provide enough sample for all analyses, which may have affected our final results. Previously, we reported that human milk was a better scavenger
of free radicals than was infant formula (5). We tested a variety of PT formulas and mother's milk from PT infants and their relative ability to scavenge free radicals and inhibit
lipid peroxidation. We found that mother's milk had a clear advantage even though known antioxidant molecules such as vitamins C and E are higher in PT formulas. Therefore, we expected
to find lower levels of F2-IsoPs in the urine of breast-fed infants compared with that of formula fed-infants. Although this occurred for those infants who were fed primarily human milk
without any supplements, an unexpected finding was the elevated urinary F2-IsoPs level in those infants who had their mother's milk fortified with HMF at the highest concentration (Fig.
1). HMF is widely used in neonatal units and was originally formulated to improve bone mineralization in PT infants (33). Why the group receiving the highest amounts of fortifier had the
highest F2-IsoPs levels and higher than those fed only formula is unclear. The manufacturer's website (enfamil.ca) is clear that feeding should be prepared in a precise manner or there
is risk of harm to the infant. In our experience, supplemental use in the neonatal unit shows wide variation with no consistent protocol (10). The known risk is that fortified human milk can
spoil quickly and the consumption of oxidized lipids will increase the total body pool of oxidized lipids (34). Antibacterial activity of human milk was almost totally inhibited by the
addition of bovine protein-based HMF (35). Other studies have shown that HMF sequesters endogenous milk anti-inflammatory cytokines (36). We have found that human milk with HMF allows
bacterial growth within 1 h (unpublished). There is a paucity of information on the proper reconstitution and use of human breast milk substitutes (37). Typical errors include over- and
under concentration, but there are persistent clinical questions as to the need for sterility, the safety and means of storage, and liquid _versus_ powder formulations. A recent Cochrane
review (38) presents disturbing data but concludes that the “Use of a multicomponent fortifiers does not appear to be associated with adverse effects, although the total number of infants
studied and the large amount of missing data reduces confidence in this conclusion.” Currently, we are engaged in research using a cell culture model in an attempt to elucidate the possible
mechanisms for generation of lipid peroxidation. Clearly, more work needs to be done. ABBREVIATIONS * AUC: area under the curve * BPD: bronchopulmonary dysplasia * F2-IsoPs: F2-isoprostanes
* HMF: human milk fortifier * IVH: intraventricular hemorrhage * ORAC: oxygen radical absorbance capacity * PT: premature * PVH: periventricular hemorrhage * ROS: reactive oxygen species *
TE: μmol Trolox equivalents REFERENCES * Boenig HV 1966 Free radicals and health: indicators for a unifying concept. _J Am Geriatr Soc_ 14: 1211–1220 Article CAS PubMed Google Scholar *
Flora SJ 2007 Role of free radicals and antioxidants in health and disease. _Cell Mol Biol (Noisy-le-grand)_ 53: 1–2 CAS Google Scholar * Blackburn S 1992 Alterations of the respiratory
system in the neonate: implications for clinical practice. _J Perinat Neonatal Nurs_ 6: 46–58 Article CAS PubMed Google Scholar * Singer D, Muhlfeld C 2007 Perinatal adaptation in
mammals: The impact of metabolic rate. _Comp Biochem Physiol A Mol Integr Physiol_ 148: 780–784 Article PubMed Google Scholar * Friel JK, Martin SM, Langdon M, Herzberg GR, Buettner GR
2002 Milk from mothers of both premature and full-term infants provides better antioxidant protection than does infant formula. _Pediatr Res_ 51: 612–618 Article PubMed Google Scholar *
Frank L, Bosanko IR 1987 Prenatal development of lung antioxidant enzymes in four species. _J Pediatr_ 110: 106–110 Article CAS PubMed Google Scholar * Asikainen TM, Raivio KO, Saksela
M, Kinnula VL 1998 Expression and developmental profile of antioxidant enzymes in human lung and liver. _Am J Respir Cell Mol Biol_ 19: 942–949 Article CAS PubMed Google Scholar * Vento
G, Mele MC, Mordente A, Romagnoli C, Matassa PG, Zecca E, Zappacosta B, Persichilli S 2000 High total antioxidant activity and uric acid in tracheobronchial aspirate fluid of preterm infants
during oxidative stress: an adaptive response to hyperoxia?. _Acta Paediatr_ 89: 336–342 Article CAS PubMed Google Scholar * Chess PR, D'Angio CT, Pryhuber GS, Maniscalco WM 2006
Pathogenesis of bronchopulmonary dysplasia. _Semin Perinatol_ 30: 171–178 Article PubMed Google Scholar * Friel JK, Diehl-Jones WL, Suh M, Tsopmo A, Shirwadkar VP 2007 Impact of iron and
vitamin C-containing supplements on preterm human milk: in vitro. _Free Radic Biol Med_ 42: 1591–1598 Article CAS PubMed Google Scholar * Ahola T, Fellman V, Kjellmer I, Raivio KO,
Lapatto R 2004 Plasma 8-isoprostane is increased in preterm infants who develop bronchopulmonary dysplasia or periventricular leukomalacia. _Pediatr Res_ 56: 88–93 Article CAS PubMed
Google Scholar * Back SA, Luo NL, Mallinson RA, O'Malley JP, Wallen LD, Frei B, Morrow JD, Petito CK, Roberts CT Jr Murdoch GH, Montine TJ 2005 Selective vulnerability of preterm white
matter to oxidative damage defined by F2-isoprostanes. _Ann Neurol_ 58: 108–120 Article CAS PubMed Google Scholar * Kermorvant-Duchemin E, Sennlaub F, Chemtob S, Behar-Cohen F 2006
[Pathophysiology of retinopathy of prematurity]. _Arch Pediatr_ 13: 566–568 Article CAS PubMed Google Scholar * Zhou Y, Wang Q, Mark Evers B, Chung DH 2006 Oxidative stress-induced
intestinal epithelial cell apoptosis is mediated by p38 MAPK. _Biochem Biophys Res Commun_ 350: 860–865 Article CAS PubMed PubMed Central Google Scholar * Inder T, Mocatta T, Darlow B,
Spencer C, Volpe JJ, Winterbourn C 2002 Elevated free radical products in the cerebrospinal fluid of VLBW infants with cerebral white matter injury. _Pediatr Res_ 52: 213–218 Article CAS
PubMed Google Scholar * Franco MC, Kawamoto EM, Gorjao R, Rastelli VM, Curi R, Scavone C, Sawaya AL, Fortes ZB, Sesso R 2007 Biomarkers of oxidative stress and antioxidant status in
children born small for gestational age: evidence of lipid peroxidation. _Pediatr Res_ 62: 204–208 Article CAS PubMed Google Scholar * Friel JK, Bessie JC, Belkhode SL, Edgecombe C,
Steele-Rodway M, Downton G, Kwa PG, Aziz K 2001 Thiamine, riboflavin, pyridoxine, and vitamin C status in premature infants receiving parenteral and enteral nutrition. _J Pediatr
Gastroenterol Nutr_ 33: 64–69 Article CAS PubMed Google Scholar * Davies SS, Zackert W, Luo Y, Cunningham CC, Frisard M, Roberts LJ II 2006 Quantification of dinor, dihydro metabolites
of F2-isoprostanes in urine by liquid chromatography/tandem mass spectrometry. _Anal Biochem_ 348: 185–191 Article CAS PubMed Google Scholar * Prior RL, Gu L, Wu X, Jacob RA, Sotoudeh G,
Kader AA, Cook RA 2007 Plasma antioxidant capacity changes following a meal as a measure of the ability of a food to alter in vivo antioxidant status. _J Am Coll Nutr_ 26: 170–181 Article
CAS PubMed Google Scholar * Cockell KA, Wotherspoon AT, Belonje B, Fritz ME, Madere R, Hidiroglou N, Plouffe LJ, Ratnayake WM, Kubow S 2005 Limited effects of combined copper deficiency:
iron overload on oxidative stress parameters in rat liver and plasma. _J Nutr Biochem_ 16: 750–756 Article CAS PubMed Google Scholar * Saker M, Mokhtari NS, Merzouk SA, Merzouk H,
Belabor B, Nacre M 2008 Oxidant and antioxidant status in mothers and their newborns according to birthweight. _Eur J Obstet Gynecol Reprod Biol_ 141: 95–99 Article CAS PubMed Google
Scholar * Farkouh CR, Merrill JD, Ballard PL, Ballard RA, Ischiropoulos H, Lorch SA 2006 Urinary metabolites of oxidative stress and nitric oxide in preterm and term infants. _Biol Neonate_
90: 233–242 Article CAS PubMed Google Scholar * Cervantes-Munguía R, Espinosa-López L, Gómez-Contreras P, Hernández-Flores G, Domínguez-Rodríguez J, Bravo-Cuéllar A 2006 Retinopathy of
prematurity and oxidative stress. _An Pediatr (Barc)_ 64: 126–131 Article Google Scholar * Buonocore G, Perrone S, Longini M, Vezzosi P, Marzocchi B, Paffetti P, Bracci R 2002 Oxidative
stress in preterm neonates at birth and on the seventh day of life. _Pediatr Res_ 52: 46–49 Article CAS PubMed Google Scholar * Weinberger B, Anwar M, Henien S, Sosnovsky A, Hiatt M,
Jochnowitz N, Witz G, Hegyi T 2004 Association of lipid peroxidation with antenatal betamethasone and oxygen radial disorders in preterm infants. _Biol Neonate_ 85: 121–127 Article CAS
PubMed Google Scholar * Reuter SD, O'Donovan DJ, Hegemier SE, Smith EO, Heird WC, Fernandes CJ 2007 Urinary F2-isoprostanes are poor prognostic indicators for the development of
bronchopulmonary dysplasia. _J Perinatol_ 27: 303–306 Article CAS PubMed Google Scholar * Fessel JP, Jackson Roberts L 2005 Isofurans: novel products of lipid peroxidation that define
the occurrence of oxidant injury in settings of elevated oxygen tension. _Antioxid Redox Signal_ 7: 202–209 Article CAS PubMed Google Scholar * Chessex P, Reichman BL, Verellen GJ, Putet
G, Smith JM, Heim T, Swyer PR 1981 Relation between heart rate and energy expenditure in the newborn. _Pediatr Res_ 15: 1077–1082 Article CAS PubMed Google Scholar * Friel JK, Friesen
RW, Harding SV, Roberts LJ 2004 Evidence of oxidative stress in full-term healthy infants. _Pediatr Res_ 56: 878–882 Article CAS PubMed Google Scholar * Aycicek A, Erel O, Kocyigit A,
Selek S, Demirkol MR 2006 Breast milk provides better antioxidant power than does formula. _Nutrition_ 22: 616–619 Article CAS PubMed Google Scholar * Hylander MA, Strobino DM, Pezzullo
JC, Dhanireddy R 2001 Association of human milk feedings with a reduction in retinopathy of prematurity among very low birthweight infants. _J Perinatol_ 21: 356–362 Article CAS PubMed
Google Scholar * van Zoeren-Grobben D, Lindeman JH, Houdkamp E, Brand R, Schrijver J, Berger HM 1994 Postnatal changes in plasma chain-breaking antioxidants in healthy preterm infants fed
formula and/or human milk. _Am J Clin Nutr_ 60: 900–906 Article CAS PubMed Google Scholar * Schanler RJ, Abrams SA 1995 Postnatal attainment of intrauterine macromineral accretion rates
in low birth weight infants fed fortified human milk. _J Pediatr_ 126: 441–447 Article CAS PubMed Google Scholar * Staprãns I, Rapp JH, Pan XM, Kim KY, Feingold KR 1994 Oxidized lipids
in the diet are a source of oxidized lipid in chylomicrons of human serum. _Arterioscler Thromb_ 14: 1900–1905 Article PubMed Google Scholar * Chan GM, Lee ML, Rechtman DJ 2007 Effects of
a human milk-derived human milk fortifier on the antibacterial actions of human milk. _Breastfeed Med_ 2: 205–208 Article PubMed Google Scholar * Jocson MA, Mason EO, Schanler RJ 1997
The effects of nutrient fortification and varying storage conditions on host defense properties of human milk. _Pediatrics_ 100: 240–243 Article CAS PubMed Google Scholar * Renfrew MJ,
Ansell P, Macleod KL 2003 Formula feed preparation: helping reduce the risks; a systematic review. _Arch Dis Child_ 88: 855–858 Article CAS PubMed PubMed Central Google Scholar *
Kuschel CA, Harding JE 2004 Multicomponent fortified human milk for promoting growth in preterm infants. _Cochrane Database Syst Rev_ CD000343 Download references ACKNOWLEDGEMENTS We thank
all parents who took part in this study. Sample collection was carried out by Cheryl Farrell, Maureen Pilz, Diana Schultz, and Lisa Merrill. Haifeng Yang, Gavin Broughton, Philip Griffin,
and Apollo Tsopmo provided technical assistance. AUTHOR INFORMATION AUTHORS AND AFFILIATIONS * Department of Human Nutritional Sciences, University of Manitoba, Winnipeg, R3T 2N2, Manitoba,
Canada James K Friel * Faculty of Nursing, University of Manitoba, Winnipeg, R3T 2N2, Manitoba, Canada Bill Diehl-Jones * Department of Pediatrics, University of Manitoba, Winnipeg, R3E 0W3,
Manitoba, Canada James K Friel & Aaron Chiu * Nutrition Research Division, Health Canada, Ottawa, K1A 0K9, Ontario, Canada Kevin A Cockell * Manitoba Institute of Child Health,
Winnipeg, R3E 3P4, Manitoba, Canada Rasheda Rabanni * Department of Pharmacology, Vanderbilt University, Nashville, 37232, TN Sean S Davies & L Jackson Roberts II Authors * James K Friel
View author publications You can also search for this author inPubMed Google Scholar * Bill Diehl-Jones View author publications You can also search for this author inPubMed Google Scholar
* Kevin A Cockell View author publications You can also search for this author inPubMed Google Scholar * Aaron Chiu View author publications You can also search for this author inPubMed
Google Scholar * Rasheda Rabanni View author publications You can also search for this author inPubMed Google Scholar * Sean S Davies View author publications You can also search for this
author inPubMed Google Scholar * L Jackson Roberts II View author publications You can also search for this author inPubMed Google Scholar CORRESPONDING AUTHOR Correspondence to James K
Friel. ADDITIONAL INFORMATION Supported from Canadian Institutes of Health Research operating Grant MOP 74463 and the Manitoba Institute of Child Health. RIGHTS AND PERMISSIONS Reprints and
permissions ABOUT THIS ARTICLE CITE THIS ARTICLE Friel, J., Diehl-Jones, B., Cockell, K. _et al._ Evidence of Oxidative Stress in Relation to Feeding Type During Early Life in Premature
Infants. _Pediatr Res_ 69, 160–164 (2011). https://doi.org/10.1203/PDR.0b013e3182042a07 Download citation * Received: 08 April 2010 * Accepted: 23 September 2010 * Issue Date: February 2011
* DOI: https://doi.org/10.1203/PDR.0b013e3182042a07 SHARE THIS ARTICLE Anyone you share the following link with will be able to read this content: Get shareable link Sorry, a shareable link
is not currently available for this article. Copy to clipboard Provided by the Springer Nature SharedIt content-sharing initiative