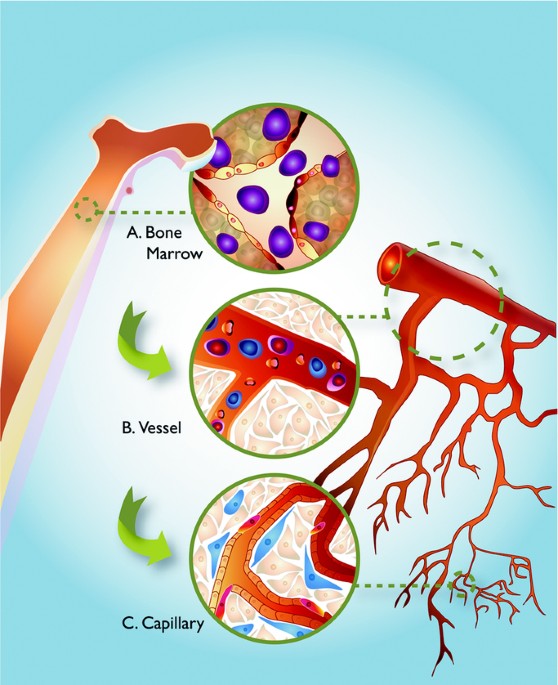
- Select a language for the TTS:
- UK English Female
- UK English Male
- US English Female
- US English Male
- Australian Female
- Australian Male
- Language selected: (auto detect) - EN
Play all audios:
ABSTRACT The concept of an Endothelial Progenitor Cell (EPC) that participates in adult angiogenesis is less than a decade old, yet it has received a great deal of attention due to its
potential for cell-based clinical therapies in many pathologies. However, controversy remains as to the identity of this bone marrow-derived cell type and its ability to give rise to new
endothelium in the adult. Reports on the contribution of EPCs to new vessels in ischemic tissue or tumors vary widely, ranging from 80–90% to negligible. As researchers hone their ability to
identify, isolate, and expand these cells by their markers and functionality, mounting evidence suggests that they might constitute multiple, but related cell types. At least two general
phenotypes have emerged from studies of bone marrow-derived cells contributing to angiogenesis: one that incorporates into the endothelial wall directly contributing to vascular expansion
and another that is able to home to neovessels, but it locates behind the endothelial wall. Nonetheless, experimental evidence indicates that this second cell type supports the viability of
newly formed vessels and thus it is equally relevant to neovascular growth. As our understanding of neovascularization in pathologic states expands, a more clear definition of the multiple
cellular components required for the process will shed light into new models of therapeutic intervention. The identification of a cell type that could be isolated, expanded and infused into
a patient would be very useful for promoting angiogenesis in ischemia, myocardial infarct and other pathologies. SIMILAR CONTENT BEING VIEWED BY OTHERS ANTI-INFLAMMATORY PROWESS OF
ENDOTHELIAL PROGENITOR CELLS IN THE REALM OF BIOLOGY AND MEDICINE Article Open access 30 September 2024 ENDOTHELIAL HETEROGENEITY IN BONE MARROW: INSIGHTS ACROSS DEVELOPMENT, ADULT LIFE AND
LEUKEMIA Article Open access 11 November 2024 DEVELOPMENT OF A POTENCY ASSAY FOR CD34+ CELL-BASED THERAPY Article Open access 11 November 2023 HISTORICAL PERSPECTIVE Vascular morphogenesis
in the embryo is initiated by a progenitor cell of mesodermal origin, the hemangioblast, capable of giving rise to endothelial and hematopoetic lineages. In the adult, however, the presence
of a common hematopoietic/endothelial progenitor has been long debated with relatively little experimental evidence. Mechanisms of postnatal angiogenesis were assumed to involve the local
outgrowth of pre-existing vessels via the expansion of mature endothelial cells in response to angiogenic growth factors. However, the search for an adult “hemangioblast” was given stronger
consideration when Asahara and colleagues described that a peripheral blood mononuclear population was able to differentiate into endothelial cells _in vitro_ and incorporate into ischemic
tissues at sites of angiogenesis (1). Shortly after Asahara first documented the presence of an endothelial progenitor in the circulating blood, Shi, and colleagues cultured a bone
marrow-derived CD34+ subset of cells and found that these cells were able to differentiate into endothelial cells and colonize aortic Dacron implants (2). These findings indicated the
existence of a bone marrow source of endothelial progenitors that could be recruited to a site of vascular repair and contribute to the expansion of the vasculature. Additional studies
reported similar phenomena demonstrating the incorporation of progenitor cells in vessels under repair in limb ischemia (3–10), sites of myocardial infarct (11–14), wound healing (1,15–17),
and atherosclerotic plaques (18,19). Models of tumor vascularization also showed bone marrow-derived cells incorporated into tumor neovessels (15,20–24). A pinnacle study, demonstrating the
dependence of bone marrow-derived endothelial progenitors for tumor growth, came in 2001, when Lyden and colleagues used an angiogenesis-impaired mouse model, a double knock-out for the
Id1/Id3 genes. This mouse failed to grow solid tumors due to poor vascular growth. Interestingly, tumor growth was restored after transplantation of wild type bone marrow, suggesting that
neovascularization of tumors required cells from the bone marrow (21). Studies before this landmark report had indicated the potential of a bone marrow-derived precursor to contribute to
endothelium, but failed to document a strict requirement of this endothelial progenitor to the progression of angiogenesis. Nonetheless the complete lack of tumor growth in the absence of
these progenitor cells and its restoration after transplantation provided a concrete demonstration that a bone marrow-derived cell was necessary and sufficient for adult neovascularization.
These studies engendered tremendous enthusiasm in the field. For the first time, rapid and clinically meaningful therapies could be exploited with vast implications to a multiplicity of
vascular diseases. The immediate challenge faced by the next wave of studies was the identification of this progenitor and the functional demonstration that this cell had a high potential
for endothelial incorporation. These initial studies identified subsets of cells with similar potential for endothelial repair, but with widely variable levels of engraftment and, possibly,
different roles in the angiogenic process. In the literature, the reported engraftment has ranged from <1% to 95% (25,26). More recently, several studies have down-played the direct
involvement of bone marrow-derived endothelial progenitors as a mechanism for the physical expansion of the endothelial wall (27–30). Rather, these results would imply that endothelial
progenitor cells (EPCs) represent a monocytic-macrophage cell that aids in the repair and angiogenic potential of vessels undergoing growth via sprouting and that home to the perivascular
space. Together, the recent findings do not directly contest the conclusions obtained by Lyden, but question the mechanism by which bone marrow-derived cells are participating in vascular
expansion and to what degree. This point has not been resolved and is the subject of much discussion. The cell surface markers, experimental models, and engraftment readout in the
aforementioned studies have varied significantly, leading to controversy as to the identity of an EPC and its role in adult angiogenesis (Table 1). Regardless, cell based therapies are
currently being explored in pilot clinical studies. Thus, an overriding theme is the need to establish a working definition for the EPC. The current definition includes a cell that: 1)
resides in the bone marrow and can be recruited by mobilizing growth factors or other cues to sites of vascular injury or tumor growth; 2) expresses surface markers that include both
endothelial and hematopoetic cell types; 3) can be distinguished from mature, differentiated circulating endothelial cells (CECs); and 4) can integrate into the endothelium at sites of
vessel repair or angiogenesis (33,40) (Fig. 1). This definition, however, in light of the above conflicting reports, remains ever changing and incomplete. The identification of all bone
marrow-derived cells involved is paramount to define their specific contributions to growing vessels. In this review we provide a summary and discussion of the limitations encountered by
investigators in this area, the novel approaches being used to reconcile conflicting reports, and the current functional view of endothelial cell progenitors. THE DIFFICULTIES IN IDENTIFYING
EPCS: TOOLS, MARKERS AND MODELS INDISPENSABLE TOOL: FLOW CYTOMETRY. Flow cytometry has proven an indispensable tool in studying endothelial progenitors. Cell surface markers that represent
cell populations with both hematopoietic and endothelial lineages can be detected, quantified and isolated for further exploration in functional assays (31,32). The method is extremely
sensitive and it provides the easiest alternative for screening large numbers of cells, quickly and accurately, using multiple parameters (markers) needed to identify rare progenitor
populations. EPCs have been shown to represent between 0.01% and 0.0001% of the total mononuclear cell fraction from a normal peripheral blood sample, making collection efforts dependent on
a very large amount of blood or pooling of multiple samples (32). A widely used method of enriching the EPC fraction in blood is to use known mobilization factors as a treatment to
artificially recruit cells into circulation. Vascular Endothelial Growth Factor (VEGF), Stromal Derived Factor-1 (SDF-1), Granulocyte-Colony Stimulating Factor (G-CSF),
Granulocyte/Macrophage- Colony Stimulating Factor (GM-CSF), and Angiopoietin 1 (Ang-1) are a few of the most used factors that can mobilize EPCs, that is, these factors have been shown to
recruit EPCs from the bone marrow into the circulation (5,6,21,33,34). The biology behind how these factors mobilize EPCs from the marrow is not entirely understood. However, it appears that
matrix metalloproteases are involved in at least one step. Matrix Metalloprotease 9 (MMP-9), an extracellular matrix metalloproteinase expressed by bone marrow stromal cells, is required
for shedding c-kit (a cell surface molecule expressed by stem/progenitor cells) from the cell surface; this event appears to be an essential signal for EPC release from the marrow stroma.
Likely, multiple mechanisms participate in the exit of EPCs from the marrow; some of these are likely to be dependent on the stimulus (VEGF _versus_ SDF-1, for example). Regardless of the
specific mechanism, the release of EPCs from the bone marrow upon exposure to these factors is well documented and is an established technique for increasing the yield of these cells
experimentally. With recent advances in cytometric technology, the explosion of available MAb, and increased knowledge in stem and progenitor cell biology, flow cytometry has become the gold
standard for characterization and isolation. However, it has limitations in the context of studying differentiating cells, as the cell surface molecules used as markers represent only a
snapshot of a highly plastic and immature cell type. Presumably, the EPC exists in multiple states of differentiation in any peripheral blood sample. A putative EPC is likely to display a
complement of surface markers when in the bone marrow that changes once released into blood stream and subsequently within the growing vessel at its final destination. Unfortunately, flow
cytometry also fails to reveal the source of the cell if not used in combination with a technique to indicate bone marrow origin. For example, mature CECs have been shown to slough off from
the endothelial wall, circulate and can be mistaken for circulating EPCs originating from the bone marrow (25,26,32,35,36). To overcome these concerns many studies currently use flow
cytometry in combination with functional assays and these have supported a significant, yet incompletely understood, role for a bone marrow-derived cell in vascular repair and
neoangiogenesis. CELL SURFACE MARKERS. Flow cytometry relies on the specificity of cell surface proteins expressed by distinct cell populations and the corresponding antibodies that are used
to identify them. The most widely used combination of surface markers for identifying peripheral blood EPCs have been CD34, Prominin-1 (CD133) and VEGFR2/flk-1 (4,26,37). Certain
considerations exist in using these markers mostly because alone, none are specific for EPCs. CD34, for example, is a hematopoietic marker and can be found on other bone marrow-derived stem
cells. In fact, EPCs represent a very small fraction of the total CD34+ population in the bone marrow or peripheral blood (32). CD34 can also be found on mature CECs (38). Therefore, CD34
must be used in conjunction with other markers to identify a more specific population of cells. A list of common markers used for EPC identification, alternate names and expression on cell
types other than EPCs can be found in Table 2. CD133 was first isolated and characterized by Weigmann, as a marker of neuroepithelial stem cells (39). It was subsequently noted by a second
group to be expressed on CD34+ hematopoetic stem cells (40,41). Since, CD133 has held great promise for being a definitive EPC marker because of its seemingly specific expression pattern in
endothelial progenitors in the adult. This was in spite of some embryonic, nonendothelial tissue expression that was detected when first cloned and characterized (38,41–43). As the
endothelial progenitor differentiates into a mature endothelial cell, CD133 is down-regulated, presumably while cells are in transit and in the process of differentiating into mature
endothelial cells. At this point, the expression profile of EPCs has been shown to change and acquire markers such as von Willebrand Factor (vWF), Platelet Endothelial Cell Adhesion
Molecule-1 (PECAM-1 (CD31)) and Vascular Endothelial-Cadherin (Ve-Cad) (44). Consistent with this notion, cultured CD133+ cells differentiate into mature endothelial cells as demonstrated by
expression of specific markers (23). Reports of CD133 expression in a bone marrow-endothelial progenitor gave weight to the notion that CD133 was a definitive marker for the EPC.
Unfortunately, subsequent studies demonstrated promiscuous expression in adult epithelial cell types, as well as other nonendothelial immature cell types in the adult (45). More recent
clinical studies on patients with nonsmall cell lung carcinoma (NSCLC) have argued against the notion that CD133 is an endothelial progenitor marker (46). Florek and colleagues used a CD133
specific antiserum as opposed to a MAb to study the adult expression pattern and found expression in the adult kidney and mammary gland (45). They also found expression at the periphery of
kidney tumors. This observation along with several others noting expression in solid tumors and in several leukemias, gave rise to the additional classification of CD133 as a cancer stem
cell marker. It should be stated that the function of CD133 is yet to be determined (42). This engenders some uncertainty about using it as a definitive endothelial progenitor marker, at
least until its role in endothelial progenitor function can be established. Lastly, the marker VEGFR2 is expressed by mature endothelial cells and is often used to delineate EPC populations
in bone marrow or peripheral blood cells. CECs, being endothelial cells sloughed from a vessel wall, also express VEGFR2, possibly mistaking differentiated cells of non-bone marrow origin
for EPCs. Thus, stem cell markers are used in combination with VEGFR2 to ensure the progenitor nature of the cell (38). Additional cell surface markers that have been used to identify cells
with progenitor characteristics are c-kit and Sca-1. The role of c-kit in EPC biology was discussed above and it relates to their mobilization from the bone marrow. Sca-1 appears to be
associated with cell fate decisions of pluripotent progenitors and regulation of c-kit expression. In fact, Sca-1(−/−) bone marrow cells had fewer myeloid progeny and decreased c-kit on
their cell surface (47). Again, when used in combination, these markers may detect more defined populations of a possible EPC, but may still exclude important intermediaries or include side
populations that do not directly contribute to angiogenesis. With the limitations of cell surface marker characterization, genetic techniques have been used to more accurately trace cell
lineages of interest. GENETIC MODELS. With no clear understanding of the contextual clues for differentiation during the transit of EPCs and the incomplete characterization of their cell
surface markers, it is difficult to pin-down the exact phenotype of the EPC population in human samples or in experimental models. Therefore, genetic markers have been used in combination
with bone marrow transplantation models to trace cells of bone marrow origin to their end point in sites of angiogenesis. Whereas cell surface markers may be transiently expressed, a genetic
marker is a permanent event regardless of the differentiation status of that cell. In some instances, Cre-recombinase in combination with a reporter gene preceded by a loxP-stop codon-loxP
cassette has been used to trace that cell and mark its entire progeny. Current genetic models that have allowed significant insight into EPC biology include Tie2/LacZ and Tie2/GFP,
SCL-1/LacZ, and Ve-Cadherin/Cre/Rosa26R. Tie-2 is a receptor tyrosine kinase expressed by endothelial and some hematopoietic cells. The promoter has been effective for tracking endothelial
progenitors in neovessels in tandem with bone marrow transplantation studies (15,48–50). The ligand for Tie2 receptor is Angiopoietin-1, and as aforementioned, Ang-1 can be used to
artificially mobilize EPCs and enrich the peripheral blood with Tie2 expressing cells. The Tie2 model has been extremely useful in tracking cells thought to be involved in postnatal
angiogenesis, however, the normal expression pattern of Tie2 in the adult vasculature remains largely uncharacterized, an unfortunate caveat for some studies. Evaluation of EPCs in tumor
angiogenesis (15) and ischemia, (15,50,51), using Tie2 expressing bone marrow cells, have shown endothelial incorporation and differentiation. However, there have been other reports of a
Tie2 expressing monocytic cell type homing to the perivascular space that does not contribute directly to the endothelial wall (27,30,52,53). Interestingly, elimination of this monocytic
cell type resulted in a significant inhibition of tumor growth in mouse xenograft assays. This finding, in combination with similar studies, plainly demonstrates the contribution of multiple
cell types in tumor angiogenesis. Recently, De Palma and colleagues characterized three distinct cell types that were targeted by a Tie2 lentiviral vector (30). Therefore, evaluation of
models using Tie2 as a genetic marker may be difficult to compare with other EPC studies, as the subset of cells being evaluated might not be entirely specific to the endothelial lineage. A
transgenic mouse using an enhancer element from the stem cell leukemia-1 (SCL-1) gene has also been used in EPC lineage studies (54). This transcription factor was identified in
hematopoietic disorders, as mutations were noted in several types of leukemia. Nonetheless, SCL was also found to be expressed by endothelial cells during development (54,55). Further
dissection of the SCL promoter using transgenic mice identified a 5′ element shown to drive expression specifically in the endothelial compartment. By using a tamoxifen-inducible reporter
system driven by the SCL-1 endothelial enhancer, Gothert and colleagues found that newly generated vessels did not contain any bone marrow-derived cells, but rather were derived from the
hosts' mature endothelial cells (28). This is in contrast to other genetic models, including those using Ve-Cadherin. Ve-Cadherin (or CD144) is an endothelial specific adhesion molecule
that binds homotypically to molecules on adjacent endothelial cells (56). The promoter of the Ve-Cadherin gene has been well characterized and its activity appears to be constitutive in all
endothelial cells during development, as well as in the adult quiescent endothelium (57–59). Because of its broad and constitutive endothelial expression, a transgenic model was developed
in which the Ve-Cadherin promoter drives expression of Cre-Recombinase (60). Once mated with the ROSA26R mouse, the resulting combination provides a functional read-out of the Cre activity
via the expression of the lacZ reporter gene (61). Being an irreversible excision, LacZ serves as permanent marker for all Ve-Cadherin expressing cells and their progeny, irrespective of the
Ve-Cadherin status of its descendents. In this way, the model offers a lineage map of cells that at one time had expressed Ve-Cadherin. This mouse has been used in bone marrow
transplantation studies and revealed low, albeit clear contribution of bone marrow cells to the endothelial wall of tumor neovessels. It has also shown monocytic cells within the
perivascular space of the tumor, a result of VE-cadherin expression in hematopoietic progenitors. The finding of monocytic cells is also consistent with reports by several other groups using
different models (27,30,52). RETROSPECTIVE HUMAN STUDIES. Perhaps one of the greatest untapped resources for evaluation of EPC incorporation is preserved human tissue obtained from biopsies
post-transplantation. One of the most convincing studies used patients that underwent sex-mismatched bone marrow transplants. Searching for the Y chromosome in tissue from female
recipients, it was possible to demonstrate bone marrow-derived cell incorporation in the endothelium. The study reports that on average 2% of the recipients' endothelium was
donor-derived (62). Human atherosclerotic plaques from sex-mismatched recipients have also been evaluated and shown to contribute to smooth muscle cells associated with the vessel along
sites of plaque formation (63). In cases of heart transplantation and coronary artery grafts, host cells were found contributing to the grafted tissues' endothelium (64). Transplanted
liver patients also displayed significant contribution to the new organs' endothelium (65). IN VITRO EXPANSION OF PROGENITOR POPULATIONS. EPCs are a rare population in the peripheral
blood and bone marrow. As aforementioned, they represent between 0.01% and 0.0001% of the total peripheral blood mononuclear cell (PB-MNC) fraction from a normal blood sample. For this
reason, researchers have cultured subsets of cells taken from total PBMNCs _in vitro_ in an effort to obtain a sufficient number of cells to test incorporation or vascular repair _in vivo_.
Many studies are also employing _in vitro_ culture to produce a large number of relatively clonogenic endothelial precursors (66). The success of _ex vivo_ amplification and/or genetic
manipulation in many reports has already yielded promise for clinical use (24,27,30,67–70). Many studies currently rely on _in vitro_ growth behavior as a selection criteria for EPCs (71).
However, there is an inherent risk in driving the cells' differentiation program by changing its microenvironment. Removing EPCs from the contextual clues present in the bone marrow
stroma or peripheral blood, or by addition of artificial signals, is likely to induce differentiation toward a particular phenotype and force highly plastic cell types to assume a precursor
identity that might not normally occur _in vivo_. In considering data from transplantation or infusion studies using ex vivo expanded cells there is, on average, a higher reported
incorporation rate than in experiments using noncultured cells, (about 7%, as opposed to 2%) (25). This increased incorporation may be due to a re-programming event, whereby culture
conditions elicit a differentiation response from multiple progenitor cell lineages toward the endothelial fate that might not occur in all cells _in vivo_. Koizumi and colleagues have
assessed the incorporation rate of organ derived endothelial cells, such as human aortic endothelial cells (HAEC) and human umbilical vein endothelial cells (HUVEC) and found both, seemingly
mature, endothelial cell types to possess the potential to survive, proliferate and incorporate, when transplanted, into mouse endothelium (72). Recently, long-term culture experiments were
performed with HAEC and HUVEC cells (73). These cells were previously thought to be short-lived in culture because of their differentiated state. However, these cells proliferated _in
vitro_ just as long as EPC cultures from other experiments. Also, a small percentage of cells in HUVEC and HAEC cultures had progenitor characteristics, similar to those of EPCs (73). This
paradox led to the conclusion that there might be tissue-resident EPCs (71). With respect to clinical applications for cell-based therapy in revascularization, the real or induced potential
of a progenitor bears little importance as long as there is measurable improvement in the patients' condition. In fact, driving differentiating cells down an endothelial path may be
useful in the repair of injured endothelium. However, for the future of EPC therapy, clarifying the contribution and identity of all involved cell types will aid in reducing side effects and
enhancing desired outcomes. THE PRO-ANGIOGENIC MONOCYTE: AN INSTRUCTIVE EPC? As mentioned above, several cell types that differ slightly in molecular cell surface repertoire of proteins
have been identified. More importantly, these cell types have displayed functional differences with regard to their effects _in vivo_. It was assumed that the most obvious role for an
endothelial progenitor would be its direct contribution to the endothelial wall. However, two phenotypes have emerged from the data regarding the differentiation fate of the EPC. One, as
mentioned above, integrating into endothelium, and another that takes residence immediately behind the vessel wall (52,74–76). The role of this second cell type is presumably to provide
paracrine signals to nearby endothelial cells (Fig. 1). In fact, this “pro-angiogenic monocyte” has been found to secrete angiogenic growth factors to the adjacent endothelial cells. Rehman
and colleagues cultured peripheral blood mononuclear cells and found that they secreted high levels of VEGF, HGF, G-CSF and GM-CSF (52). It has become apparent that mural cells, which
consist of pericytes and vascular smooth muscle cells, are critical mediators in the angiogenic response and directly contribute to vascular stability and remodeling. In a recent study, Tie2
expressing monocytic cells were co-injected with tumor cells. In that study, it was found that there was a significant increase in the level and quality of angiogenesis compared with
injecting tumor cells alone (30). Numerous studies have reinforced the importance of interaction between the vascular endothelium and mural cells (reviewed by Armulik, 2005) (77). Therefore,
it is conceivable that a marrow-derived cell could aid in the establishment of new capillary beds by providing important paracrine signals and not by contributing directly to the
endothelial wall. The pro-angiogenic monocyte would then, by the current definition, not be an EPC. These observations present a paradigm shift from the simplistic view that a single cell
type is responsible for angiogenic growth in adult tissues and steers toward a process where multiple bone marrow-derived cells are involved. FUTURE DIRECTIONS The potential therapeutic
value of EPCs is undisputed. What remains in dispute, however, is the definition of the EPC. The specific questions that will help to define the EPC are: Which are the pro-angiogenic
progenitors in the bone marrow? What is the identity (cell surface repertoire) of the EPC while in circulation? What are its roles in angiogenesis? By striving to more accurately
characterize, the cells involved in the process of postnatal angiogenesis, by both phenotype and function, researchers will be able to better compare their results and select subsets of
cells with advantageous characteristics for use in future clinical trials. At least two independent bone marrow-derived cell types have now been described and implicated in vascular
expansion/viability using various techniques. One has been shown to differentiate into a mature endothelial cell and directly incorporates into the endothelial wall. The other appears to
have monocytic features, incorporates behind the endothelial wall, and secretes pro-angiogenic growth factors, presumably to enhance the survival and growth of the nearby resident
endothelial cells. Rather than juxtaposing these data, some investigators viewed this as quite fortuitous, as multiple subsets of cells could modulate angiogenesis by independent mechanisms
that could be exploited in the development of strategies for therapy. Clinical trials with subsets of bone marrow cells are progressing with extreme caution, with the understanding that
inclusion of side population cells that might not contribute to angiogenesis could be harmful. The most appealing explanation for the diversity of observations of EPC function is a highly
plastic cell type with the ability to respond to the vascular needs of the immediate surroundings once it has been recruited to the site of angiogenesis. Due to their accessibility and rapid
response, circulating EPCs have been used as surrogate markers for a wide variety of pathologies. Several experimental studies have shown a direct correlation between EPCs and pathologic
status. In fact, these cells have been shown to correlate with ischemia extent in a stroke or myocardial infarction; level of angiogenesis in tumors; and risk factor status in interventions
associated with atherosclerosis. While the use of EPCs as surrogate markers in the clinic might occur soon; a more direct exploration of these cells as therapeutic tools in patients is
long-reached. Additional information from basic science is needed to aid in the development of safe and predictable therapies with measurable outcomes. There is little doubt that adult
angiogenesis is a more complex process than previously anticipated. Nonetheless, persistent exploration of the biology of EPCs in preclinical settings will most likely allow a quick
transition of this technology into clinically relevant therapies. ABBREVIATIONS * CEC: circulating endothelial cell * EPC: endothelial progenitor cell * HAEC: human aortic endothelial cell *
HUVEC: human umbilical vein endothelial cell * VEGF: vascular endothelial growth factor * VEGFR2: vascular endothelial growth factor receptor 2 REFERENCES * Asahara T, Murohara T, Sullivan
A, Silver M, van der Zee R, Li T, Witzenbichler B, Schatteman G, Isner JM 1997 Isolation of putative progenitor endothelial cells for angiogenesis. _Science_ 275: 964–967 Article CAS
PubMed Google Scholar * Shi Q, Rafii S, Wu MH, Wijelath ES, Yu C, Ishida A, Fujita Y, Kothari S, Mohle R, Sauvage LR, Moore MA, Storb RF, Hammond WP 1998 Evidence for circulating bone
marrow-derived endothelial cells. _Blood_ 92: 362–367 CAS PubMed Google Scholar * Asahara T, Kalka C, Isner JM 2000 Stem cell therapy and gene transfer for regeneration. _Gene Ther_ 7:
451–457 Article CAS PubMed Google Scholar * Asahara T, Isner JM 2002 Endothelial progenitor cells for vascular regeneration. _J Hematother Stem Cell Res_ 11: 171–178 Article PubMed
Google Scholar * Takahashi T, Kalka C, Masuda H, Chen D, Silver M, Kearney M, Magner M, Isner JM, Asahara T 1999 Ischemia- and cytokine-induced mobilization of bone marrow-derived
endothelial progenitor cells for neovascularization. _Nat Med_ 5: 434–438 Article CAS PubMed Google Scholar * Kalka C, Masuda H, Takahashi T, Gordon R, Tepper O, Gravereaux E, Pieczek A,
Iwaguro H, Hayashi SI, Isner JM, Asahara T 2000 Vascular endothelial growth factor(165) gene transfer augments circulating endothelial progenitor cells in human subjects. _Circ Res_ 86:
1198–1202 Article CAS PubMed Google Scholar * Schatteman GC, Hanlon HD, Jiao C, Dodds SG, Christy BA 2000 Blood-derived angioblasts accelerate blood-flow restoration in diabetic mice. _J
Clin Invest_ 106: 571–578 Article CAS PubMed PubMed Central Google Scholar * Iwaguro H, Yamaguchi J, Kalka C, Murasawa S, Masuda H, Hayashi S, Silver M, Li T, Isner JM, Asahara T 2002
Endothelial progenitor cell vascular endothelial growth factor gene transfer for vascular regeneration. _Circulation_ 105: 732–738 Article CAS PubMed Google Scholar * Yamaguchi J, Kusano
KF, Masuo O, Kawamoto A, Silver M, Murasawa S, Bosch-Marce M, Masuda H, Losordo DW, Isner JM, Asahara T 2003 Stromal cell-derived factor-1 effects on ex vivo expanded endothelial progenitor
cell recruitment for ischemic neovascularization. _Circulation_ 107: 1322–1328 Article CAS PubMed Google Scholar * Urbich C, Heeschen C, Aicher A, Dernbach E, Zeiher AM, Dimmeler S 2003
Relevance of monocytic features for neovascularization capacity of circulating endothelial progenitor cells. _Circulation_ 108: 2511–2516 Article PubMed Google Scholar * Orlic D,
Kajstura J, Chimenti S, Limana F, Jakoniuk I, Quaini F, Nadal-Ginard B, Bodine DM, Leri A, Anversa P 2001 Mobilized bone marrow cells repair the infarcted heart, improving function and
survival. _Proc Natl Acad Sci U S A_ 98: 10344–10349 Article CAS PubMed PubMed Central Google Scholar * Orlic D, Kajstura J, Chimenti S, Bodine DM, Leri A, Anversa P 2001 Transplanted
adult bone marrow cells repair myocardial infarcts in mice. _Ann N Y Acad Sci_ 938: 221–229; discussion 229–230. Article CAS PubMed Google Scholar * Jackson KA, Majka SM, Wang H, Pocius
J, Hartley CJ, Majesky MW, Entman ML, Michael LH, Hirschi KK, Goodell MA 2001 Regeneration of ischemic cardiac muscle and vascular endothelium by adult stem cells. _J Clin Invest_ 107:
1395–1402 Article CAS PubMed PubMed Central Google Scholar * Kocher AA, Schuster MD, Szabolcs MJ, Takuma S, Burkhoff D, Wang J, Homma S, Edwards NM, Itescu S 2001 Neovascularization of
ischemic myocardium by human bone-marrow-derived angioblasts prevents cardiomyocyte apoptosis, reduces remodeling and improves cardiac function. _Nat Med_ 7: 430–436 Article CAS PubMed
Google Scholar * Asahara T, Masuda H, Takahashi T, Kalka C, Pastore C, Silver M, Kearne M, Magner M, Isner JM 1999 Bone marrow origin of endothelial progenitor cells responsible for
postnatal vasculogenesis in physiological and pathological neovascularization. _Circ Res_ 85: 221–228 Article CAS PubMed Google Scholar * Asahara T, Takahashi T, Masuda H, Kalka C, Chen
D, Iwaguro H, Inai Y, Silver M, Isner JM 1999 VEGF contributes to postnatal neovascularization by mobilizing bone marrow-derived endothelial progenitor cells. _EMBO J_ 18: 3964–3972 Article
CAS PubMed PubMed Central Google Scholar * Crosby JR, Kaminski WE, Schatteman G, Martin PJ, Raines EW, Seifert RA, Bowen-Pope DF 2000 Endothelial cells of hematopoietic origin make a
significant contribution to adult blood vessel formation. _Circ Res_ 87: 728–730 Article CAS PubMed Google Scholar * Sata M, Saiura A, Kunisato A, Tojo A, Okada S, Tokuhisa T, Hirai H,
Makuuchi M, Hirata Y, Nagai R 2002 Hematopoietic stem cells differentiate into vascular cells that participate in the pathogenesis of atherosclerosis. _Nat Med_ 8: 403–409 Article CAS
PubMed Google Scholar * Luttun A, Tjwa M, Moons L, Wu Y, Angelillo-Scherrer A, Liao F, Nagy JA, Hooper A, Priller J, De Klerck B, Compernolle V, Daci E, Bohlen P, Dewerchin M, Herbert JM,
Fava R, Matthys P, Carmeliet G, Collen D, Dvorak HF, Hicklin DJ, Carmeliet P 2002 Revascularization of ischemic tissues by PlGF treatment, and inhibition of tumor angiogenesis, arthritis and
atherosclerosis by anti-Flt1. _Nat Med_ 8: 831–840 Article CAS PubMed Google Scholar * Garcia-Barros M, Paris F, Cordon-Cardo C, Lyden D, Rafii S, Haimovitz-Friedman A, Fuks Z,
Kolesnick R 2003 Tumor response to radiotherapy regulated by endothelial cell apoptosis. _Science_ 300: 1155–1159 Article CAS PubMed Google Scholar * Lyden D, Hattori K, Dias S, Costa C,
Blaikie P, Butros L, Chadburn A, Heissig B, Marks W, Witte L, Wu Y, Hicklin D, Zhu Z, Hackett NR, Crystal RG, Moore MA, Hajjar KA, Manova K, Benezra R, Rafii S 2001 Impaired recruitment of
bone-marrow-derived endothelial and hematopoietic precursor cells blocks tumor angiogenesis and growth. _Nat Med_ 7: 1194–1201 Article CAS PubMed Google Scholar * Reyes M, Dudek A,
Jahagirdar B, Koodie L, Marker PH, Verfaillie CM 2002 Origin of endothelial progenitors in human postnatal bone marrow. _J Clin Invest_ 109: 337–346 Article CAS PubMed PubMed Central
Google Scholar * Gehling UM, Ergun S, Schumacher U, Wagener C, Pantel K, Otte M, Schuch G, Schafhausen P, Mende T, Kilic N, Kluge K, Schafer B, Hossfeld DK, Fiedler W 2000 In vitro
differentiation of endothelial cells from AC133-positive progenitor cells. _Blood_ 95: 3106–3112 CAS PubMed Google Scholar * Davidoff AM, Ng CY, Brown P, Leary MA, Spurbeck WW, Zhou J,
Horwitz E, Vanin EF, Nienhuis AW 2001 Bone marrow-derived cells contribute to tumor neovasculature and, when modified to express an angiogenesis inhibitor, can restrict tumor growth in mice.
_Clin Cancer Res_ 7: 2870–2879 CAS PubMed Google Scholar * Urbich C, Dimmeler S 2004 Endothelial progenitor cells: characterization and role in vascular biology. _Circ Res_ 95: 343–353
Article CAS PubMed Google Scholar * Rafii S, Lyden D, Benezra R, Hattori K, Heissig B 2002 Vascular and haematopoietic stem cells: novel targets for anti-angiogenesis therapy?. _Nat Rev
Cancer_ 2: 826–835 Article CAS PubMed Google Scholar * De Palma M, Venneri MA, Roca C, Naldini L 2003 Targeting exogenous genes to tumor angiogenesis by transplantation of genetically
modified hematopoietic stem cells. _Nat Med_ 9: 789–795 Article CAS PubMed Google Scholar * Gothert JR, Gustin SE, van Eekelen JA, Schmidt U, Hall MA, Jane SM, Green AR, Gottgens B, Izon
DJ, Begley CG 2004 Genetically tagging endothelial cells in vivo: bone marrow-derived cells do not contribute to tumor endothelium. _Blood_ 104: 1769–1777 Article PubMed Google Scholar *
Jain RK, Duda DG 2003 Role of bone marrow-derived cells in tumor angiogenesis and treatment. _Cancer Cell_ 3: 515–516 Article CAS PubMed Google Scholar * De Palma M, Venneri MA, Galli
R, Sergi LS, Politi LS, Sampaolesi M, Naldini L 2005 Tie2 identifies a hematopoietic lineage of proangiogenic monocytes required for tumor vessel formation and a mesenchymal population of
pericyte progenitors. _Cancer Cell_ 8: 211–226 Article CAS PubMed Google Scholar * Masuda H, Kalka C, Asahara T 2000 Endothelial progenitor cells for regeneration. _Hum Cell_ 13: 153–160
CAS PubMed Google Scholar * Khan SS, Solomon MA, McCoy JP 2005 Detection of circulating endothelial cells and endothelial progenitor cells by flow cytometry. _Cytometry B Clin Cytom_ 64:
1–8 Article PubMed Google Scholar * Aicher A, Zeiher AM, Dimmeler S 2005 Mobilizing endothelial progenitor cells. _Hypertension_ 45: 321–325 Article CAS PubMed Google Scholar *
Hattori K, Dias S, Heissig B, Hackett NR, Lyden D, Tateno M, Hicklin DJ, Zhu Z, Witte L, Crystal RG, Moore MA, Rafii S 2001 Vascular endothelial growth factor and angiopoietin-1 stimulate
postnatal hematopoiesis by recruitment of vasculogenic and hematopoietic stem cells. _J Exp Med_ 193: 1005–1014 Article CAS PubMed PubMed Central Google Scholar * Khakoo AY, Finkel T
2005 Endothelial progenitor cells. _Annu Rev Med_ 56: 79–101 Article CAS PubMed Google Scholar * Lin Y, Weisdorf DJ, Solovey A, Hebbel RP 2000 Origins of circulating endothelial cells
and endothelial outgrowth from blood. _J Clin Invest_ 105: 71–77 Article CAS PubMed PubMed Central Google Scholar * Hristov M, Erl W, Weber PC 2003 Endothelial progenitor cells:
isolation and characterization. _Trends Cardiovasc Med_ 13: 201–206 Article CAS PubMed Google Scholar * Peichev M, Naiyer AJ, Pereira D, Zhu Z, Lane WJ, Williams M, Oz MC, Hicklin DJ,
Witte L, Moore MA, Rafii S 2000 Expression of VEGFR-2 and AC133 by circulating human CD34(+) cells identifies a population of functional endothelial precursors. _Blood_ 95: 952–958 CAS
PubMed Google Scholar * Weigmann A, Corbeil D, Hellwig A, Huttner WB 1997 Prominin, a novel microvilli-specific polytopic membrane protein of the apical surface of epithelial cells, is
targeted to plasmalemmal protrusions of non-epithelial cells. _Proc Natl Acad Sci U S A_ 94: 12425–12430 Article CAS PubMed PubMed Central Google Scholar * Yin AH, Miraglia S, Zanjani
ED, Almeida-Porada G, Ogawa M, Leary AG, Olweus J, Kearney J, Buck DW 1997 AC133, a novel marker for human hematopoietic stem and progenitor cells. _Blood_ 90: 5002–5012 CAS PubMed Google
Scholar * Miraglia S, Godfrey W, Yin AH, Atkins K, Warnke R, Holden JT, Bray RA, Waller EK, Buck DW 1997 A novel five-transmembrane hematopoietic stem cell antigen: isolation,
characterization, and molecular cloning. _Blood_ 90: 5013–5021 CAS PubMed Google Scholar * Shmelkov SV, St Clair R, Lyden D, Rafii S 2005 AC133/CD133/Prominin-1. _Int J Biochem Cell Biol_
37: 715–719 Article CAS PubMed Google Scholar * Handgretinger R, Gordon PR, Leimig T, Chen X, Buhring HJ, Niethammer D, Kuci S 2003 Biology and plasticity of CD133+ hematopoietic stem
cells. _Ann N Y Acad Sci_ 996: 141–151 Article CAS PubMed Google Scholar * Rafii S, Lyden D 2003 Therapeutic stem and progenitor cell transplantation for organ vascularization and
regeneration. _Nat Med_ 9: 702–712 Article CAS PubMed Google Scholar * Florek M, Haase M, Marzesco AM, Freund D, Ehninger G, Huttner WB, Corbeil D 2005 Prominin-1/CD133, a neural and
hematopoietic stem cell marker, is expressed in adult human differentiated cells and certain types of kidney cancer. _Cell Tissue Res_ 319: 15–26 Article CAS PubMed Google Scholar *
Hilbe W, Dirnhofer S, Oberwasserlechner F, Schmid T, Gunsilius E, Hilbe G, Woll E, Kahler CM 2004 CD133 positive endothelial progenitor cells contribute to the tumour vasculature in
non-small cell lung cancer. _J Clin Pathol_ 57: 965–969 Article CAS PubMed PubMed Central Google Scholar * Bradfute SB, Graubert TA, Goodell MA 2005 Roles of Sca-1 in hematopoietic
stem/progenitor cell function. _Exp Hematol_ 33: 836–843 Article CAS PubMed Google Scholar * De Palma M, Naldini L 2002 Transduction of a gene expression cassette using advanced
generation lentiviral vectors. _Methods Enzymol_ 346: 514–529 Article CAS PubMed Google Scholar * Asahara T, Chen D, Takahashi T, Fujikawa K, Kearney M, Magner M, Yancopoulos GD, Isner
JM 1998 Tie2 receptor ligands, angiopoietin-1 and angiopoietin-2, modulate VEGF-induced postnatal neovascularization. _Circ Res_ 83: 233–240 Article CAS PubMed Google Scholar * Zhang ZG,
Zhang L, Jiang Q, Chopp M 2002 Bone marrow-derived endothelial progenitor cells participate in cerebral neovascularization after focal cerebral ischemia in the adult mouse. _Circ Res_ 90:
284–288 Article CAS PubMed Google Scholar * Tepper OM, Capla JM, Galiano RD, Ceradini DJ, Callaghan MJ, Kleinman ME, Gurtner GC 2005 Adult vasculogenesis occurs through in situ
recruitment, proliferation, and tubulization of circulating bone marrow-derived cells. _Blood_ 105: 1068–1077 Article CAS PubMed Google Scholar * Rehman J, Li J, Orschell CM, March KL
2003 Peripheral blood “endothelial progenitor cells” are derived from monocyte/macrophages and secrete angiogenic growth factors. _Circulation_ 107: 1164–1169 Article PubMed Google Scholar
* Gulati R, Jevremovic D, Peterson TE, Chatterjee S, Shah V, Vile RG, Simari RD 2003 Diverse origin and function of cells with endothelial phenotype obtained from adult human blood. _Circ
Res_ 93: 1023–1025 Article CAS PubMed Google Scholar * Begley CG, Green AR 1999 The SCL gene: from case report to critical hematopoietic regulator. _Blood_ 93: 2760–2770 CAS PubMed
Google Scholar * Drake CJ, Brandt SJ, Trusk TC, Little CD 1997 TAL1/SCL is expressed in endothelial progenitor cells/angioblasts and defines a dorsal-to-ventral gradient of vasculogenesis.
_Dev Biol_ 192: 17–30 Article CAS PubMed Google Scholar * Lampugnani MG, Corada M, Caveda L, Breviario F, Ayalon O, Geiger B, Dejana E 1995 The molecular organization of endothelial cell
to cell junctions: differential association of plakoglobin, beta-catenin, and alpha-catenin with vascular endothelial cadherin (VE-cadherin). _J Cell Biol_ 129: 203–217 Article CAS PubMed
Google Scholar * Gory S, Vernet M, Laurent M, Dejana E, Dalmon J, Huber P 1999 The vascular endothelial-cadherin promoter directs endothelial-specific expression in transgenic mice.
_Blood_ 93: 184–192 CAS PubMed Google Scholar * Hisatsune H, Matsumura K, Ogawa M, Uemura A, Kondo N, Yamashita JK, Katsuta H, Nishikawa S, Chiba T, Nishikawa S 2005 High level of
endothelial cell-specific gene expression by a combination of the 5′ flanking region and the 5′ half of the first intron of the VE-cadherin gene. _Blood_ 105: 4657–4663 Article CAS PubMed
Google Scholar * Prandini MH, Dreher I, Bouillot S, Benkerri S, Moll T, Huber P 2005 The human VE-cadherin promoter is subjected to organ-specific regulation and is activated in tumour
angiogenesis. _Oncogene_ 24: 2992–3001 Article CAS PubMed PubMed Central Google Scholar * Alva JA, Zovein AC, Monvoisin A, Murphy T, Salazaar A, Harvey N, Carmeliet P, Iruela-Arispe ML
2006 VE-Cadherin Cre recombinase transgenic mouse: a tool for lineage analysis and gene deletion in endothelial cells. _Dev Dyn_, in press. * Soriano P 1999 Generalized lacZ expression with
the ROSA26 Cre reporter strain. _Nat Genet_ 21: 70–71 Article CAS PubMed Google Scholar * Jiang S, Walker L, Afentoulis M, Anderson DA, Jauron-Mills L, Corless CL, Fleming WH 2004
Transplanted human bone marrow contributes to vascular endothelium. _Proc Natl Acad Sci U S A_ 101: 16891–16896 Article CAS PubMed PubMed Central Google Scholar * Caplice NM, Bunch TJ,
Stalboerger PG, Wang S, Simper D, Miller DV, Russell SJ, Litzow MR, Edwards WD 2003 Smooth muscle cells in human coronary atherosclerosis can originate from cells administered at marrow
transplantation. _Proc Natl Acad Sci U S A_ 100: 4754–4759 Article CAS PubMed PubMed Central Google Scholar * Quaini F, Urbanek K, Beltrami AP, Finato N, Beltrami CA, Nadal-Ginard B,
Kajstura J, Leri A, Anversa P 2002 Chimerism of the transplanted heart. _N Engl J Med_ 346: 5–15 Article PubMed Google Scholar * Hove WR, van Hoek B, Bajema IM, Ringers J, van Krieken JH,
Lagaaij EL 2003 Extensive chimerism in liver transplants: vascular endothelium, bile duct epithelium, and hepatocytes. _Liver Transpl_ 9: 552–556 Article PubMed Google Scholar * Hur J,
Yoon CH, Kim HS, Choi JH, Kang HJ, Hwang KK, Oh BH, Lee MM, Park YB 2004 Characterization of two types of endothelial progenitor cells and their different contributions to neovasculogenesis.
_Arterioscler Thromb Vasc Biol_ 24: 288–293 Article CAS PubMed Google Scholar * Ferrari N, Glod J, Lee J, Kobiler D, Fine HA 2003 Bone marrow-derived, endothelial progenitor-like cells
as angiogenesis-selective gene-targeting vectors. _Gene Ther_ 10: 647–656 Article CAS PubMed Google Scholar * Jevremovic D, Gulati R, Hennig I, Diaz RM, Cole C, Kleppe L, Cosset FL,
Simari RD, Vile RG 2004 Use of blood outgrowth endothelial cells as virus-producing vectors for gene delivery to tumors. _Am J Physiol Heart Circ Physiol_ H494–500 Article CAS PubMed
Google Scholar * Wei J, Blum S, Unger M, Jarmy G, Lamparter M, Geishauser A, Vlastos GA, Chan G, Fischer KD, Rattat D, Debatin KM, Hatzopoulos AK, Beltinger C 2004 Embryonic endothelial
progenitor cells armed with a suicide gene target hypoxic lung metastases after intravenous delivery. _Cancer Cell_ 5: 477–488 Article CAS PubMed Google Scholar * Kaushal S, Amiel GE,
Guleserian KJ, Shapira OM, Perry T, Sutherland FW, Rabkin E, Moran AM, Schoen FJ, Atala A, Soker S, Bischoff J, Mayer JE 2001 Functional small-diameter neovessels created using endothelial
progenitor cells expanded ex vivo. _Nat Med_ 7: 1035–1040 Article CAS PubMed PubMed Central Google Scholar * Ingram DA, Caplice NM, Yoder MC 2005 Unresolved questions, changing
definitions, and novel paradigms for defining endothelial progenitor cells. _Blood_ 106: 1525–1531 Article CAS PubMed Google Scholar * Koizumi K, Tsutsumi Y, Kamada H, Yoshioka Y,
Watanabe M, Yamamoto Y, Okamoto T, Mukai Y, Nakagawa S, Tani Y, Mayumi T 2003 Incorporation of adult organ-derived endothelial cells into tumor blood vessel. _Biochem Biophys Res Commun_
306: 219–224 Article CAS PubMed Google Scholar * Ingram DA, Mead LE, Moore DB, Woodard W, Fenoglio A, Yoder MC 2005 Vessel wall-derived endothelial cells rapidly proliferate because they
contain a complete hierarchy of endothelial progenitor cells. _Blood_ 105: 2783–2786 Article CAS PubMed Google Scholar * Salven P, Mustjoki S, Alitalo R, Alitalo K, Rafii S 2003 VEGFR-3
and CD133 identify a population of CD34+ lymphatic/vascular endothelial precursor cells. _Blood_ 101: 168–172 Article CAS PubMed Google Scholar * Conejo-Garcia JR, Benencia F, Courreges
MC, Kang E, Mohamed-Hadley A, Buckanovich RJ, Holtz DO, Jenkins A, Na H, Zhang L, Wagner DS, Katsaros D, Caroll R, Coukos G 2004 Tumor-infiltrating dendritic cell precursors recruited by a
beta-defensin contribute to vasculogenesis under the influence of Vegf-A. _Nat Med_ 10: 950–958 Article CAS PubMed Google Scholar * Rajantie I, Ilmonen M, Alminaite A, Ozerdem U, Alitalo
K, Salven P 2004 Adult bone marrow-derived cells recruited during angiogenesis comprise precursors for periendothelial vascular mural cells. _Blood_ 104: 2084–2086 Article CAS PubMed
Google Scholar * Armulik A, Abramsson A, Betsholtz C 2005 Endothelial/pericyte interactions. _Circ Res_ 97: 512–523 Article CAS PubMed Google Scholar Download references
ACKNOWLEDGEMENTS We would like to thank Amanda Hammond for her artistic rendering in Fig. 1 and Dr. Sunyoung Lee and Dr. Minako Partyka for critical evaluation of the review. AUTHOR
INFORMATION AUTHORS AND AFFILIATIONS * Molecular Biology Institute, University of California, Los Angeles, Los Angeles, 90095, CA Chad L Barber * Department of Molecular, Cellular and
Developmental Biology, University of California, Los Angeles, Los Angeles, 90095, CA M Luisa Iruela-Arispe * Jonsson Comprehensive Cancer Center, University of California, Los Angeles, Los
Angeles, 90095, CA M Luisa Iruela-Arispe Authors * Chad L Barber View author publications You can also search for this author inPubMed Google Scholar * M Luisa Iruela-Arispe View author
publications You can also search for this author inPubMed Google Scholar CORRESPONDING AUTHOR Correspondence to M Luisa Iruela-Arispe. ADDITIONAL INFORMATION This work was supported by
grants from the National Institutes of Health to M. Luisa Iruela-Arispe (1RO1CA77420, 2RO1CA65624, 1RO1HL074455). Chad L. Barber holds a Pre-Doctoral Fellowship from the American Heart
Association (0515003Y). RIGHTS AND PERMISSIONS Reprints and permissions ABOUT THIS ARTICLE CITE THIS ARTICLE Barber, C., Iruela-Arispe, M. The Ever-Elusive Endothelial Progenitor Cell:
Identities, Functions and Clinical Implications. _Pediatr Res_ 59 (Suppl 4), 26–32 (2006). https://doi.org/10.1203/01.pdr.0000203553.46471.18 Download citation * Received: 16 November 2005 *
Accepted: 21 December 2005 * Issue Date: 01 April 2006 * DOI: https://doi.org/10.1203/01.pdr.0000203553.46471.18 SHARE THIS ARTICLE Anyone you share the following link with will be able to
read this content: Get shareable link Sorry, a shareable link is not currently available for this article. Copy to clipboard Provided by the Springer Nature SharedIt content-sharing
initiative