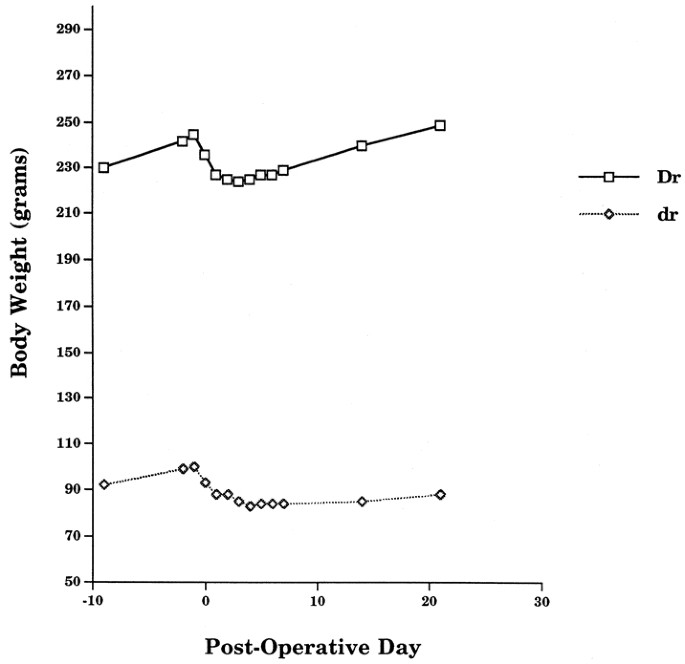
- Select a language for the TTS:
- UK English Female
- UK English Male
- US English Female
- US English Male
- Australian Female
- Australian Male
- Language selected: (auto detect) - EN
Play all audios:
ABSTRACT The residual small bowel undergoes profound adaptive alterations after surgical resection. GH is considered to have a role in regulation of these adaptive changes, but its precise
role is unknown. We investigated the role of GH by studying the response to intestinal resection in rats with isolated GH deficiency. Spontaneous dwarf rats, a strain of rats with congenital
isolated GH deficiency, underwent 60% resection of the small intestine and parameters of the response of the intestinal remnant were compared with age-matched GH-deficient rats undergoing
transection, GH-normal rats undergoing 60% resection, and nonmanipulated GH-normal rats. Deficiency of GH did not inhibit hyperplasia of the mucosal mass of the intestinal remnant,
indicating that GH is not required for regulation of this aspect of the adaptive response. However, GH deficiency resulted in lack of accumulation of mucosal protein, including lack of
accumulation of digestive hydrolases. In addition, GH deficiency resulted in alterations in processing of digestive hydrolases of the distal intestine, indicating that GH may have
region-specific effects on small intestinal function. We conclude that GH is required for the normal expression of specific components of the adaptive response to massive small intestinal
resection, but not for all aspects. The aspects that require GH appear to involve protein synthesis and processing. SIMILAR CONTENT BEING VIEWED BY OTHERS BARIATRIC SURGERY INDUCES A NEW
GASTRIC MUCOSA PHENOTYPE WITH INCREASED FUNCTIONAL GLUCAGON-LIKE PEPTIDE-1 EXPRESSING CELLS Article Open access 04 January 2021 INTESTINAL-DERIVED FGF15 PROTECTS AGAINST DELETERIOUS EFFECTS
OF VERTICAL SLEEVE GASTRECTOMY IN MICE Article Open access 06 August 2021 INTESTINAL RESECTION AFFECTS WHOLE-BODY ARGININE SYNTHESIS IN NEONATAL PIGLETS Article 13 September 2020 MAIN
Surgical resection of the small intestine provokes a profound adaptive response in the intestinal remnant characterized by dramatic hyperplasia of the mucosal epithelium and enhanced
segmental absorption of nutrients (1–4). These changes result in increased functional capacity of the residual intestine. Multiple lines of evidence suggest GH may be essential for the
marked increase in intestinal growth seen in adaptation to surgical resection. Our group has shown that GH administration is required for normalization of intestinal growth in rats
hypophysectomized in early infancy (5). Receptors for GH have been identified on crypt and villus epithelial cells of the small intestine (6). Small bowel growth has been shown to be
enhanced in transgenic mice overexpressing GH (7). Finally, GH is the major regulator of IGF-I, administration of which has been shown to result in enhanced intestinal adaptation after
resection (8, 9). Few studies have been performed to investigate the role of GH in adaptation of the residual small bowel after surgical resection in which exogenous human recombinant GH was
administered to GH-sufficient rats after small bowel resection. However, contradictory results were obtained, rendering the role of GH in this setting unclear (10–12). To clarify the
specific role of GH in regulation of adaptation of the small intestine to surgical resection, we studied a unique strain of Sprague Dawley rat, the SDR. The dwarfism results from an
autosomal recessive point mutation in the GH gene that causes abnormal splicing and a one base deletion in the GH mRNA resulting in markedly reduced levels of GH message. As the small
amounts of GH mRNA are nontranslatable, there is complete absence of GH protein (13). No GH is detectable in the pituitary of SDR rats when analyzed by PAGE, immunoblot analysis, RIA, or
immunocytochemistry. However, the deficiency of GH is isolated as no effect on prolactin, ACTH, TSH, or LH production has been detected (14). This model offers the opportunity for specific
investigation of GH and GH-dependent processes without methodological complications introduced by use of hypophysectomy or administration of exogenous GH. Using this model, we have examined
the role of GH or processes dependent upon or regulated by GH in intestinal adaptation to massive small bowel resection. We have previously used this model for investigation of the role of
GH in regulation of postnatal intestinal maturation (15). MATERIALS AND METHODS CHEMICALS AND REAGENTS. Sucrose, lactose, glucose oxidase (type X), horseradish peroxidase (type VI),
p-hydroxybenzoic acid, aminoantipyrine, leucyl-beta-napthylamide, phenylmethylsulfonylflouride, EDTA, and p-hydroxymercuribenzoate were purchased from Sigma Chemical Co. (St. Louis, MO,
U.S.A.). Hoechst H-33258 was obtained from Calbiochem (San Diego, CA, U.S.A.). BioRad reagent for protein assay was purchased from BioRad (Richmond, CA, U.S.A.). PEPTIDES. Recombinant human
IGF-1 was obtained from Bachen (Torrance, CA, USA). Recombinant, nonglycosylated IGFBP-3 from _Escherichia coli_ was a generous gift from Celtrix (Santa Clara, CA, U.S.A.). Peptides were
iodinated using a modification of the chloramine T method (16) to specific activities of 350–500 mCi/mg for IGF-I. Polyclonal antiserum for IGF-I was generously provided by the hormone
distribution program of the National Institute of Arthritis, Digestive Diseases and Kidney Diseases (NIADDK) and was originally developed by Drs. J.J. VanWyk and L.E. Underwood (17).
ANIMALS. Control animals were the offspring of pregnant dams of the Sprague Dawley strain purchased from Simonsen Laboratories (Gilroy, CA, U.S.A.). The strain of SDR was generously provided
by Dr. S. Okuma (Morishita Pharmaceutical Co., Ltd., Shiga, Japan). These rats carry an autosomal recessive mutation in the GH gene, represented by DR. Their expression of pituitary GH mRNA
is only 3–6% that of control animals, due to an aberrant splice site in the mRNA. Furthermore, this truncated mRNA is not translated into the mature protein due to premature translational
termination, such that no GH peptide can be measured in the anterior pituitary gland of SDR (13, 14). Unlike other GH-deficient dwarf rat strains available, these rats are unique because
they lack only GH and are otherwise pituitary-sufficient. All animals were housed in the facilities of the Department of Laboratory Animal Medicine of the Stanford University Medical Center.
The animals were maintained at 21 ± 1°C and had a 12-h light, 12-h dark cycle, with lights on at 0700 h. Standard food and water were available _ad libitum_. The protocols for animal use
were approved by the Department of Laboratory Animal Medicine, Stanford University. INTESTINAL RESECTION. Adult GH-deficient rats, designated DR, were taken at approximately 3 mo of age and
were given clear liquids only for 24 h before intestinal resection. At the time of operation they were anesthetized with an intramuscular injection of 40 mg/kg ketamine. Once sedated, the
animals received an i.p. injection of 3 mg/kg Rompun. The abdomen was shaved and then cleansed with povidone-iodine and isopropyl alcohol. Midline laparotomy was followed by evisceration and
measurement of the small intestine from the ligament of Treitz to the ileocecal valve by placing a measured suture along the antimesenteric border of the gently stretched bowel. The bowel
was divided 5 cm from the ligament of Treitz and 60% of the measured length of small bowel was then resected. Primary end-to-end anastomosis was accomplished with interrupted 9-0 nylon
suture. The abdominal wall was closed with 7-0 polypropylene suture in a running two-layer fashion. At completion of the operation, the animals received i.p. injections of 100 mg/kg
Ampicllin and 5 mg/kg Kanamycin, and a s.c. injection of 1.1 mg/kg Flunixin. The animals were returned to a warm cage until fully recovered. Once fully awake, they were allowed _ad libitum_
oral rehydration solution with glucose and electrolytes only, for the first 24 h after surgery. Beginning on the second postoperative day, the rats were placed in individual cages and given
rat chow and water _ad libitum_. Daily chow intake and body weight were measured. Two additional GH-deficient groups of rats were studied, one group that underwent intestinal transection
without resection (DS), and one group that was not surgically manipulated (DC). Control GH-sufficient animals of the same age, designated DR, similarly underwent intestinal resection by the
identical techniques described above. A control group consisting of nonsurgically manipulated GH-sufficient animals (DC) was also studied. ANALYSIS OF INTESTINAL TISSUES. Animals were killed
by cervical dislocation on postoperative d 21. The residual small intestine from the ligament of Treitz to the ileocecal valve was removed and flushed with cold isotonic saline. Pre- and
postanastomotic intestinal lengths were measured. The intestinal segments were then weighed and stored at −80°C for subsequent analysis. In nonresected animals, sections of small intestine
corresponding to those in resected animals were collected for analysis. Before freezing, small sections were placed in formalin for microscopic analysis. At the time of analysis, whole
intestinal samples were homogenized in 10 vol buffer (10 mM sodium phosphate and 5 mM Tris-HCl, pH 6.0) containing the protease inhibitors 1 mM phenylmethylsulfonylfluoride and 0.1 mM
p-hydroxymercuribenzoate. Activities of lactase-phlorizin hydrolase, and sucrase-isomaltase were determined from homogenates of whole intestine by the method of Dahlqvist (18), as modified
by Tsuboi (19). Lactose was used as substrate for brush border lactase. The activity of AOP, the major peptidase of the brush border membrane, was measured using 0.17 mM
leucyl-β-naphthylamide as substrate, as previously described (20). Protein was determined by the BioRad assay, using BSA as the standard (21). DNA content was determined by the method of
Labarca and Paigen (22). Enzyme activity units (U) were defined as micromoles of substrate hydrolyzed per minute at 37°C, and specific activities were expressed as milliunits of enzyme
activity per milligram protein. Tissues for histology were embedded in paraffin, sectioned along the longitudinal axes, and stained with hematoxylin and eosin. Villus height was determined
under blinded conditions. IMMUNOPRECIPITATION AND GEL ELECTROPHORESIS. The particulate and brush border membrane fractions were solubilized by sonication in PBS containing 1% Triton X-100
and the protease inhibitor mixture and were centrifuged at 100,000 ×_g_ for 30 min. Lactase, sucrase, and AOP were immunoprecipitated from the supernatant using monospecific, polyclonal
antibodies as previously described (19, 23–25). The immune pellets were washed three times in 62.5 mM Tris, pH 6.8, resuspended in 62.5 mM Tris, pH 6.8, 2% SDS, 10% glycerol, 5%
2-mercaptoethanol, and heated at 95°C for 3 min. SDS-PAGE was performed in 7.0% separating gels according to the method of Laemmli (26). The gels were stained with Coomassie blue. IGF
MEASUREMENT. Sera from each animal (25–50 μL) were diluted to 0.5 mL in 1.0% (wt/vol) formic acid and subjected to size-exclusion chromatography through a G50 Sephadex column (Pharmacia,
Piscataway, NJ, U.S.A.) in 1.0% (wt/vol) formic acid. The IGF were in the protein fraction eluted between 50 and 85 mL and were collected in siliconized glass tubes containing 1.0 mL of 1.0%
(wt/vol) BSA. Following lyophilization, samples were reconstituted to 1.0 mL in 10 mmol HCl/L, before storage at −20°C. IGF-I was measured by RIA, as previously described (16). The minimal
detectable concentrations were 0.1 mg/L for IGF-I. The interassay coefficients of variation were 11% and 12% for the IGF-I assays. STATISTICS. All data were analyzed using InStat, a
statistical software package provided by GraphPad Software (San Diego, CA, U.S.A.). All results are presented as the mean ± SE. Groups were compared using ANOVA. RESULTS The mortality rate
for the intestinal resection in the GH-sufficient animal was 25% (eight animals underwent resection and six survived). The mortality rate for the GH-deficient animals undergoing resection or
transection was 36% in that 14 animals underwent surgery and 9 survived. Dwarf rats required only 50% of the anesthetic dose required by GH-sufficient animals per body weight, most likely
reflecting their altered physiologic state. SOMATIC GROWTH AND DIETARY INTAKE. As expected, the growth of the GH-sufficient (DR) animals exceeds that of their GH-deficient (DR) counterparts
before surgery. As shown in Figure 1, DR animals are gaining approximately 1.65 g/d compared with the DR animals' growth of 1.0 g/d. This is consistent with previously published data
(27). Following resection, there is a period of approximately 5–7 d during which time the body weight of DR animals decreases before returning to preoperative levels. At this point, DR
animals begin to gain weight rapidly. In contrast, DR animals do not regain their preoperative weight even by 21 d after surgery (Fig. 1). The postoperative growth curve for this group of
animals is essentially flat. Despite this, DR animals remained active and well groomed. The dietary intake data are given in Table 1. No significant differences were noted in dietary intake
among the four groups of animals. No differences in intake were noted between GH-deficient animals that underwent intestinal transection (DS) and those that were not surgically manipulated
(DC). These data were therefore pooled and given under DC. PARAMETERS OF INTESTINAL GROWTH. On the 21st postoperative day, resected animals were killed and the pre- and postanastomotic
intestinal remnants were weighed, measured, and stored frozen for subsequent analysis. At analysis, no differences in results were found between the DC and DS groups, hence the data from
these two animal groups were pooled and given as DC. The parameters of the preanastomotic intestinal segments are given in Table 2. In GH-sufficient animals, the preanastomotic segments of
animals that underwent intestinal resection showed a two- to threefold increase in weight/length, DNA/centimeter, and protein content/centimeter, thus exhibiting the adaptive growth expected
after resection (DC _versus_ DR). In DR, significant increases in intestinal wt/length and DNA/cm were also observed. Increases in protein content/centimeter were also noted but these did
not achieve statistical significance (DR _versus_ DC). The results of the postanastomotic segment are given in Table 3. In the postanastomotic remnant of GH-sufficient animals, significant
increases were observed in weight for length, DNA/centimeter, and protein/centimeter. In contrast, the postanastomotic segments of GH-deficient animals showed no increases in weight/length
or protein/centimeter, whereas DNA/centimeter was significantly elevated. The results of the histologic measurements performed in this study are given in Figure 2. In GH-sufficient animals
(DR), there were significant increases in the villus height in both the pre- and postanatomotic segment. In contrast, in GH deficiency, the villus height of the preanastomotic segment also
increased in response to intestinal resection, whereas the villi of the postanastomotic segment of GH-deficient animals did not and were significantly shorter. DIGESTIVE HYDROLASE
PARAMETERS. The catalytic activities of the key digestive hydrolases, lactase, sucrase, and aminooligopeptidase were measured and the results are given in Figures 3 and 4. In the
preanastomotic segment (Fig. 3), no differences in enzyme specific activities are noted in GH-sufficient animals, but lactase and sucrase were significantly higher than control in
GH-deficiency. No differences were noted in AOP activity levels. Total enzyme activities (expressed as mU/cm intestine) were significantly higher in intestines of GH-sufficient animals,
whereas no differences were noted in GH-deficient animals (Table 2). Similar findings were noted in the postanastomotic segment. As seen in Figure 4, lactase- and sucrase-specific activities
were no different in GH-sufficient animals whereas AOP levels were significantly higher in control animals. Lactase and sucrase were higher in GH-deficiency whereas aminooligopeptidase
activities were unchanged. As shown in Table 3, total activities of the hydrolases were all markedly elevated in GH-sufficiency, whereas in GH-deficiency, total activities were either
unchanged (AOP) or lower than control (lactase, sucrase). Analysis of the subunit structure of the hydrolases was done by SDS-PAGE. As seen in Figure 5, the subunit structure of lactase is
identical in GH-sufficiency and GH-deficiency in the preanastomotic segment. However, in the postanastomotic segment of GH-deficient animals, the major 130 kDa lactase band is missing and
two lower molecular weight lactase subunits appear, suggesting altered processing of lactase. Similar findings were noted on analysis of AOP by SDS-PAGE. The subunit structure is identical
in the preanastomotic segments of both GH-sufficient and GH-deficient animals, whereas the major 140 kDa band migrates more slowly in the postanastomotic segment of GH-deficient animals
(Fig. 7). This suggests that the processing of the AOP protein is altered in GH-deficiency. No differences in sucrase subunits were noted (Fig. 6). SERUM LEVELS OF IGF-I. As a further
confirmation of GH status, serum IGF-I levels, a peptide known to be GH dependent, were determined on a subset of three animals from each experimental group. The results were DR = 1186 ± 262
ng IGF-I/mL serum, DC = 1277 ± 227 ng IGF-I/mL serum, DR = 18 ± 4 ng IGF-I/mL serum, DC = 29.5 ± 6 ng IGF-I/mL serum. As is apparent, GH-deficient animals had exceedingly low levels of
IGF-I, as expected. DISCUSSION Although previous studies have suggested a role for GH in regulation of intestinal adaptation to resection (12, 28), the specific role of GH has not yet been
elucidated. To study the role of GH, a resection of 60% of the mid-small bowel was performed on rats congenitally deficient in GH. The mechanism of GH deficiency in this rat model has been
extensively investigated and has been established as an isolated deficiency of GH (27, 29). This model therefore allows investigation of the specific role of GH in regulation of the
augmentation of growth of the intestinal remnant that characterizes adaptation to surgical resection. Massive resection of the small intestine results in profound hyperplasia of the mucosal
epithelium, producing markedly increased digestive and absorptive function of the remnant intestine (2–4, 30–33). Features of this classic response are exhibited by our group of
GH-sufficient animals undergoing intestinal resection (DR). As seen in Table 2 and Table 3, both the pre- and postanastomotic segments of DR intestine showed increased segmental weight, DNA
content, protein content, and villus height compared with analogous intestinal segments in control animals not undergoing intestinal resection (DC). In addition, segmental enzyme activities
are also significantly higher in DR as opposed to DC. Because enzyme specific activities were generally no different in DR and DC (Figs. 3 and 4), the increases in enzyme content are most
likely due to increased numbers of epithelial cells containing a normal concentration of enzyme, as has been proposed previously (1, 34). A different pattern of response is seen in the
intestines of GH-deficient animals. Although increases in segmental weight and DNA content are noted generally, no significant increases in segmental protein content occurred, including lack
of increase or actual decrease in segmental enzyme-protein content. These data suggest that GH is not required for mucosal cell proliferation and hyperplasia after intestinal resection but
is required for the normal expression of the profound accumulation of mucosal protein and digestive hydrolases that normally occurs in response to intestinal resection. The metabolism of the
digestive hydrolases investigated in this study was clearly different in GH-deficient animals after intestinal resection. Instead of the well-described increases in total intestinal
digestive enzyme content that is an essential feature of adaptation to surgical resection, total intestinal digestive enzyme levels were either no different from control (preanastomotic
segment) or actually lower than control (postanastomotic segment) in GH deficiency after resection (Tables 2 and 3). In addition, the specific activities for lactase and sucrase were reduced
in both segments in GH-deficient animals after resection to levels similar to those found in GH normal animals. (Figs. 3 and 4). The mechanism for the reduction in lactase- and
sucrase-specific activities after intestinal resection in GH deficiency is unclear. Because no differences in dietary intake were found (Table 1), these lower enzyme activities cannot be
ascribed to lack of luminal nutrients. Baseline specific activities of lactase and sucrase in transected (control) GH deficient small bowel are elevated relative to levels found in resected
GH-deficient intestine as well as those levels found in GH normal intestine (Figs. 3 and 4). The etiology of this finding itself is not clear, but may reflect the relatively lower intestinal
protein content of the GH deficient bowel (Tables 2 and 3). We have previously suggested that decreased intestinal protein may be a distinguishing feature in GH deficiency (15). Indeed,
increased levels of intestinal protein are found in mice overexpressing GH (7). As enzyme-specific activities are calculated by dividing enzyme catalytic activities by intestinal protein
levels, lower levels of intestinal protein will result in elevated specific activity levels. Other possibilities for this finding include alterations in cell lifespan, increased rates of
enzyme synthesis, or decreased rates of enzyme degradation, but these were not directly examined in this study. To investigate possible mechanisms for the reductions in enzyme-specific
activities in GH-deficient animals after intestinal resection, analysis of subunit structure of the enzyme-protein was done by SDS-PAGE. No differences in migration of enzyme subunits were
noted in the preanastomotic segments of GH-deficient animals, but obvious differences were noted in the distal segments for AOP and lactase. In GH-deficiency, the AOP band clearly migrates
more slowly than in GH-sufficient animals. Although the mechanism of this difference is unclear, it is possible that this difference reflects alterations in glycosylation. For AOP, we have
shown that the proximal and distal differences in migration of AOP subunits were due to regional differences in glycosylation, as removal of the carbohydrate sidechains by endoglycosidase
treatment resulted in AOP enzyme-protein bands that no longer differed in migration (24). Striking differences in lactase subunit structure were noted in the distal intestinal segment in GH
deficiency. The absence of the normally present 130 kDa form and the appearance of a lower molecular weight doublet indicates that the processing of the lactase enzyme-protein was
dramatically altered in absence of GH. These data indicate that GH is required for the normal adaptive increases in digestive hydrolases after intestinal resection. In absence of GH, not
only is there a lack of increase in digestive enzyme content, but alterations in lactase and AOP enzyme structure are produced. Whether these changes in enzyme structure result in
alterations in enzyme metabolism, such as increased rates of degradation, is unclear. Other potential mechanisms for alterations in enzyme-specific activities to be considered include
alterations in epithelial cell lifespan. Epithelial cell lifespan has been shown to be decreased due presumably to more rapid cellular turnover after intestinal resection (1). This may
result in decreased enzyme activities because of accelerated enzyme loss (23). The mechanisms by which GH acts on intestinal tissues to regulate protein synthesis in general and digestive
hydrolase metabolism in particular are not known. GH is a potent anabolic hormone that stimulates nitrogen retention and accumulation of other nutrients essential for growth (16). As GH may
stimulate an increase in dietary intake (12), it is possible that GH deficiency might result in diminished nutrient intake and hence adversely affect intestinal adaptation, which is known to
require enteral nutrients (1). As shown in Table 1, however, all groups of GH-deficient animals had equivalent dietary intakes to GH-normal animals, in spite of slow rates of growth (Fig.
1). Hence the alterations in the adaptive response to intestinal resection observed in this study are not due to diminished dietary intake in this animal model. Alternatively, the lack of GH
could have resulted in lack of substrate for protein synthesis in GH-dependent tissues. It has been shown that a short course of supplemental GH therapy enhances amino acid uptake in the
human small bowel (35). In absence of GH, it is therefore possible that amino acid transport in the small intestine is diminished, resulting in diminished levels of protein synthesis in
intestinal tissues. Finally, it is also possible that GH deficiency results in alterations in protein synthesis or rates of protein turnover such that abnormal and possibly inactive proteins
are synthesized or rates of protein turnover are increased to make amino acid substrates more available for local or systemic sites. In summary, we have investigated the role of GH in the
adaptive response to massive small intestinal resection in rats with isolated GH deficiency. In GH deficiency, the marked hyperplasia of the mucosal epithelium, characteristically seen in
the adaptive response, was preserved in both the proximal (jejunal) and distal (ileal) intestinal remnant, indicating that this feature of intestinal adaptation to resection is not GH
dependent. However, GH deficiency resulted in lack of accumulation of intestinal protein in both segments, including lack of accumulation of digestive hydrolases. In addition, GH deficiency
resulted in alterations in processing of digestive hydrolases of the distal intestine, indicating that GH has region-specific effects on the small intestine. In the aggregate, these data
indicate that specific components of the adaptive response to massive small intestinal resection require GH for their normal expression, but other components do not. Because of these
findings, studies investigating the effect of exogenous GH in intestinal resection would very likely produce no effect on intestinal hyperplasia, as this is not a GH-dependent process.
However, there may be an effect seen on the digestive hydrolases of the distal intestinal remnant, as we have demonstrated alterations in digestive enzyme activity and structure in this
region of the intestine in GH-deficient animals undergoing surgical resection. Indeed, the results of a recent report appear to support these predictions in that the authors report the
infusion of GH in rats undergoing massive small bowel resection did not stimulate hyperplasia but did stimulate sucrase and maltase levels in the ileal remnant (10). ABBREVIATIONS * SDR:
spontaneous dwarf rats * dr: adult GH deficient rats undergoing intestinal resection * ds: adult GH deficient rats undergoing intestinal transection * dc: adult GH deficient rats not
surgically manipulated * Dr: adult GH normal rats undergoing intestinal resection * Dc: adult GH normal rats not surgically manipulated * AOP: aminooligopeptidase REFERENCES * Williamson R,
Chir M 1978 Intestinal adaptation. _N Engl J Med_ 298: 1393–1450 Article CAS Google Scholar * Dowling RE, Booth CC 1967 Structural and functional changes following small intestinal
resection in the rat. _Clin Sci_ 32: 139–149 CAS PubMed Google Scholar * Dowling RH, Gleeson MH 1973 Cell turnover following small bowel resection and by-pass. _Digestion_ 8: 176–190
Article CAS Google Scholar * Dowling RH 1982 Small bowel adaptation and its regulation. _Scand J Gastroenterol_ 17( suppl 74) : 53–74 Google Scholar * Castillo RO, Glasscock GF, Noren
KM, Reisenauer AM 1991 Pituitary regulation of postnatal small intestinal ontogeny in the rat: differential regulation of digestive hydrolase maturation by thyroxine and growth hormone.
_Comp Biochem Physiol C Pharmacol Toxicol_ 129: 1417–1423 CAS Google Scholar * Lobie PE, Breipohl S, Water MJ 1990 Growth hormone receptor expression in the rat gastrointestinal tract.
_Comp Biochem Physiol C Pharmacol Toxicol_ 126: 299–306 CAS Google Scholar * Ulshen MH, Dowling RH, Fuller CR, Zimmerman EM, Lund PK 1993 Enhanced growth of small bowel in transgenic mice
overexpressing bovine growth hormone. _Gastroenterol_ 104: 973–980 Article CAS Google Scholar * Lemmey AB, Martin AA, Read LC, Thomas FM, Owens PC, Ballard FJ 1991 IGF-I and the truncated
analogue des-(1–3)-IGF-I enhance growth in rats after gut resection. _Am J Physiol_ 260: E213–E219 Article CAS Google Scholar * Vanderhoof JA, McCusker RH, Clark R, Mohammadpour H,
Blackwood DJ, Harty RF, Park JHY 1992 Truncated and native insulin-like growth factor I enhance mucosal adaptation after jejunoileal resection. _Gastroenterology_ 102: 1949–1956 Article CAS
Google Scholar * Park J, Vanderhoof J 1996 Growth hormone did not enhance mucosal hyperplasia after small bowel resection. _Scand J Gastroenterol_ 31: 349–354 Article CAS Google Scholar
* Benhamou PH, Canarelli JP, Leroy C, DeBoissieu D, Dupont C 1994 Stimulation by recombinant human growth hormone of growth and development of remaining bowel after subtotal
ileojejunectomy in rats. _J Pediatr Gastroenterol Nutr_ 18: 446–452 Article CAS Google Scholar * Schulman DI, Hu CS, Duckett G, Lavelle-Grey M 1992 Effects of short-term growth hormone
therapy in rats undergoing 75% small intestinal resection. _J Pediatr Gastroenterol Nutr_ 14: 3–11 Article Google Scholar * Takeuchi T, Suzuki H, Sakurai S, Nogami H, Okuma S, Ishikawa H
1990 Molecular mechanism of growth hormone (GH) deficiency in the spontaneous dwarf rat: detection of abnormal splicing of GH messenger ribonucleic acid by polymerase chain reaction. _Comp
Biochem Physiol C Pharmacol Toxicol_ 126: 31–38 CAS Google Scholar * Nogami H, Takeuchi T, Suzuki K, Okuma S, Ishikawa H 1989 Studies on prolactin and growth hormone gene expression in the
pituitary gland of spontaneous dwarf rats. _Comp Biochem Physiol C Pharmacol Toxicol_ 125: 964–970 CAS Google Scholar * Durant M, Gargosky S, Dahlstrom K, Hellman B, Castillo R 1996
Regulation of postnatal intestinal maturation by growth hormone: studies in rats with isolated growth hormone deficiency. _Pediatr Res_ 40: 88–93 Article CAS Google Scholar * Gargosky SE,
Tapanainen P, Rosenfeld RG 1994 Administration of growth hormone (GH) but not insulin-like growth factor-I (IGF-I), by continuous infusion can induce the formation of the 150-kilodalton
IGF-binding protein-3 complex in GH-deficient rats. _Comp Biochem Physiol C Pharmacol Toxicol_ 134: 2267–2276 CAS Google Scholar * Underwood LE, Wyk JJV 1992 _Normal and Aberrant Growth_.
WB Saunders, Philadelphia, 1075–1138. Google Scholar * Dahlqvist A 1964 Method for assay of intestinal disaccharidases. _Ann Biochem_ 7: 18–25 Article CAS Google Scholar * Tsuboi KK,
Kwong LK, Burrill PH, Sunshine P 1979 Sugar hydrolases and their arrangement on the rat intestinal microvillus membrane. _J Membr Biol_ 50: 101–122 Article CAS Google Scholar *
Wojnarowska F, Gray GM 1975 Intestinal surface peptide hydrolases: identification and characterization of three enzymes from rat brush border. _Biochimica Biophysica Acta_ 403: 147–160
Article CAS Google Scholar * Bradford MM 1972 A rapid and sensitive method for the quantitation of microgram quantities of protein utilizing the principle of protein-dye binding. _Ann
Biochem_ 72: 248–254 Article Google Scholar * Labarca C, Paigen K 1980 A simple, rapid and sensitive DNA assay procedure. _Ann Biochem_ 102: 344–352 Article CAS Google Scholar *
Castillo RO, Reisenauer AM, Kwong LK, Tsuboi KK, Quan R, Gray GM 1990 Intestinal lactase in the neonatal rat: maturational changes in intracellular processing and brush border degradation.
_J Biol Chem_ 265: 15889–15893 CAS PubMed Google Scholar * Reisenauer AM, Lee EA, Castillo RO 1992 Ontogeny of membrane and soluble aminooligopeptidases in rat intestine. _Am J Physiol_
262: G178–G184 CAS PubMed Google Scholar * Tsuboi KK, Schwartz SM, Burrill PH, Kwong LK, Sunshine P 1979 Sugar hydrolases of the infant rat intestine and their arrangement on the brush
border membrane. _Biochimica Biophysica Acta_ 554: 234–248 Article CAS Google Scholar * Laemmli UK 1970 Cleavage of structural proteins during the assembly of the head of bacteriophage
T4. _Nature_ 227: 680–685 Article CAS Google Scholar * Okuma S, Kawashima S 1980 Spontaneous dwarf rat. _Exp Animal Sci_ 29: 301 Google Scholar * Hart MH, Phares CK, Erdnman SH,
Grandjean CJ, Park JHY, Vanderhoof JA 1987 Augmentation of postresection mucosal hyperplasia by Plerocercoid growth factor (PCF) analog of human growth hormone. _Dig Dis Sci_ 32: 1275–1280
Article CAS Google Scholar * Park JHY, Vanderhoof JA, Blackwood D, MacDonald RG 1990 Characterization of type I and type II insulin-like growth factor receptors in an intestinal
epithelial cell line. _Comp Biochem Physiol C Pharmacol Toxicol_ 126: 2998–3005 CAS Google Scholar * Weser E, Hernandez MH 1971 Studies of small bowel adaptation after intestinal resection
in the rat. _Gastroenterology_ 60: 69–75 CAS PubMed Google Scholar * Vanderhoof JA, Burkley KT, Antonson DL 1983 Potential for mucosal adaptation following massive small bowel resection
in 3-week versus 8-week old rats. _J Pediatr Gastroenterol Nutr_ 2: 672–676 Article CAS Google Scholar * Johnson LR 1988 Regulation of gastrointestinal mucosal growth. _Physiol Rev_ 68:
456–502 Article CAS Google Scholar * Booth CC, Evans KT, Menzies T, Street DF 1959 Intestinal hypertrophy following partial resection of the small bowel in the rat. _Br J Surg_ 46:
403–410 Article CAS Google Scholar * Hartman GE, Castillo RO, Kwong LK, Sunshine P, Tsuboi KK 1988 Maturational patterns of carbohydrases in the ileal remnant of rats after jejunectomy at
infancy. _Am J Clin Nutr_ 47: 868–874 Article CAS Google Scholar * Inoue Y, Copeland E, Souba W 1994 Growth hormone enhances amino acid uptake by the human small intestine. _Ann Surg_
219: 715–724 Article CAS Google Scholar Download references AUTHOR INFORMATION AUTHORS AND AFFILIATIONS * Department of Pediatrics Stanford University School of Medicine, Stanford, CA,
U.S.A. Michael Durant, Sharron E Gargosky, Rixun Fang & Ricardo O Castillo * Department of Pathology Stanford University School of Medicine, Stanford, CA, U.S.A. Barry H Hellman *
Digestive Disease Center Stanford University School of Medicine, Stanford, CA, U.S.A. Ricardo O Castillo * Department of Pediatrics, Huddinge Hospital, Karolinska Institute, Stockholm,
Sweden K Anders Dahlstrom Authors * Michael Durant View author publications You can also search for this author inPubMed Google Scholar * Sharron E Gargosky View author publications You can
also search for this author inPubMed Google Scholar * K Anders Dahlstrom View author publications You can also search for this author inPubMed Google Scholar * Rixun Fang View author
publications You can also search for this author inPubMed Google Scholar * Barry H Hellman View author publications You can also search for this author inPubMed Google Scholar * Ricardo O
Castillo View author publications You can also search for this author inPubMed Google Scholar ADDITIONAL INFORMATION Supported in part by National Institutes of Health grant HD 27366 and
grants from the Throne-Holst Foundation, the Swedish Medical Research Council, the Swedish Medical Association, Crown Princess Lovisas Fund, the Karolinska Institute, and Sandoz
Nutritionals. RIGHTS AND PERMISSIONS Reprints and permissions ABOUT THIS ARTICLE CITE THIS ARTICLE Durant, M., Gargosky, S., Dahlstrom, K. _et al._ The Role of Growth Hormone in Adaptation
to Massive Small Intestinal Resection in Rats. _Pediatr Res_ 49, 189–196 (2001). https://doi.org/10.1203/00006450-200102000-00010 Download citation * Received: 02 February 2000 * Accepted:
27 June 2000 * Issue Date: 01 February 2001 * DOI: https://doi.org/10.1203/00006450-200102000-00010 SHARE THIS ARTICLE Anyone you share the following link with will be able to read this
content: Get shareable link Sorry, a shareable link is not currently available for this article. Copy to clipboard Provided by the Springer Nature SharedIt content-sharing initiative