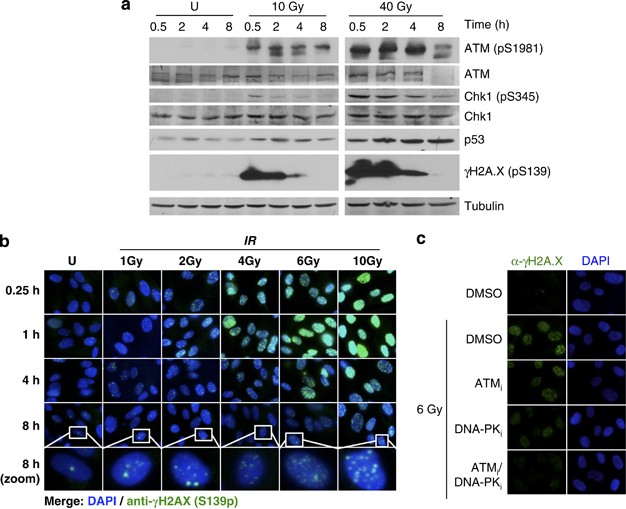
- Select a language for the TTS:
- UK English Female
- UK English Male
- US English Female
- US English Male
- Australian Female
- Australian Male
- Language selected: (auto detect) - EN
Play all audios:
ABSTRACT Primary cells respond to irradiation by activation of the DNA damage response and cell cycle arrest, which eventually leads to senescence or apoptosis. It is not clear in detail
which signaling pathways or networks regulate the induction of either apoptosis or senescence. Primary human fibroblasts are able to withstand high doses of irradiation and to prevent
irradiation-induced apoptosis. However, the underlying regulatory basis for this phenotype is not well understood. Here, a kinetic network analysis based on reverse phase protein arrays
(RPPAs) in combination with extensive western blot and cell culture analyses was employed to decipher the cytoplasmic and nuclear signaling networks and to identify possible antiapoptotic
pathways. This analysis identified activation of known DNA damage response pathways (e.g., phosphorylation of MKK3/6, p38, MK2, Hsp27, p53 and Chk1) as well as of prosurvival (e.g., MEK-ERK,
cAMP response element-binding protein (CREB), protein kinase C (PKC)) and antiapoptotic markers (e.g., Bad, Bcl-2). Interestingly, PKC family members were activated early upon irradiation,
suggesting a regulatory function in the ionizing radiation (IR) response of these cells. Inhibition or downregulation of PKC in primary human fibroblasts caused IR-dependent downregulation
of the identified prosurvival (CREB phosphorylation) and antiapoptotic (Bad phosphorylation, Bcl-2) markers and thus lead to a proliferation stop and to apoptosis. Taken together, our
analysis suggests that cytoplasmic PKC signaling conditions IR-stressed MRC-5 and IMR-90 cells to prevent irradiation-induced apoptosis. These findings contribute to the understanding of the
cellular and nuclear IR response and may thus eventually improve the efficacy of radiotherapy and help overcome tumor radioresistance. SIMILAR CONTENT BEING VIEWED BY OTHERS PPM1D ACTIVITY
PROMOTES CELLULAR TRANSFORMATION BY PREVENTING SENESCENCE AND CELL DEATH Article Open access 05 September 2024 A DNA-PK PHOSPHORYLATION SITE ON MET REGULATES ITS SIGNALING INTERFACE WITH THE
DNA DAMAGE RESPONSE Article Open access 15 May 2023 TARGETING TOPK SENSITISES TUMOUR CELLS TO RADIATION-INDUCED DAMAGE BY ENHANCING REPLICATION STRESS Article Open access 09 November 2020
MAIN Genotoxic stress such as ionizing radiation (IR) causes DNA damage and thereby induces diverse cellular responses such as cell cycle arrest and the activation of the DNA damage response
(DDR) pathways to deal with this threat.1 These complex DDRs are regulated by the PI3K-like kinases ATM, ATR and DNA-PK, which regulate downstream effectors that induce cell cycle
checkpoints via phosphorylation of checkpoint kinases (Chk1/2), phosphatases (Cdc25) as well as stabilization of p53 and activation of the p16INK4a-Rb pathway to delay progression into the S
phase or mitosis.2 Following the detection of DNA breaks, ATM initiates a nuclear signaling cascade, leading to the phosphorylation of protein substrates such as histone H2A.X, which is
thus modified into _γ_H2A.X and sensitively marks individual DNA breaks.3 Depending on the cell type, prolonged cell cycle arrest and extensive DNA damage leads to apoptosis or senescence,4,
5, 6 but the molecular mechanism leading to either of these outcomes is not understood in detail. Recent large-scale network analyses have extended our understanding not only of genotoxic
stress signaling and identified new phosphorylation but also of acetylation sites and target proteins mainly in the nucleus.7, 8, 9, 10, 11 Reverse phase protein arrays (RPPA) represent a
powerful technology for the sensitive detection and high-throughput quantification of protein changes at the single-cell level in a multiplex setting.12, 13 Protein arrays have already been
applied to study changed expression levels or post-translational modification status of proteins in cellular stress conditions or in normal and cancerous prostate and ovarian tissue.10, 13,
14, 15 Depending on the cell type, IR induces different responses and proteome changes. MRC-5 primary human lung fibroblasts were used in this study as a model cell strain, because these
cells tolerate IR doses up to 80 Gy and do not induce apoptosis.16, 17 Here, we applied the RPPA technology to study IR-induced stress pathways in primary cells on a ‘signalosome level’ by
analyzing cellular candidate markers. The RPPAs were probed with 165 different antibodies to quantify the major components and post-translational modifications of nuclear and cytoplasmic
stress signaling events. Besides classical components of the DDR, such as protein level and phosphorylation changes in Chk1, p53, p21 and cyclin D1, as well as activation of the MEK-ERK,
p38-Hsp27 and LKB-AMPK pathways, the RPPA analysis described here also identified specific components of the cellular IR response involved in antiapoptotic and prosurvival signaling. Among
these changes was also the activation of PKC family members. The PKC signaling pathway is known to integrate extracellular signals, calcium and secondary lipid messengers (diacylglycerol,
phosphatidylserine), to regulate diverse cellular responses, including apoptosis or survival during genotoxic stress.18, 19, 20 We found that experimental inhibition or downregulation of PKC
led to downregulation of prosurvival (cAMP response element-binding protein (CREB) phosphorylation) and antiapoptotic (Bad phosphorylation, Bcl-2) markers upon IR treatment and thus lead to
apoptosis in normal human MRC-5 and IMR-90 fibroblasts (normal human fibroblasts (NHFs)). These results thus implicated significantly changed kinetics of prosurvival (CREB phosphorylation)
and antiapoptotic (Bad phosphorylation, Bcl-2) key determinants in the ability to prevent apoptosis upon severe DNA damage. IR-induced PKC signaling is thus defined as the mechanism that
prevents IR-induced apoptosis. RESULTS IR INDUCES A DDR AND SENESCENCE IN MRC-5 FIBROBLASTS To induce DNA damage, primary human MRC-5 fibroblasts were irradiated with doses of 10 and 40 Gy.
Both doses induced key components of the classical DDR, including phosphorylation of ATM at serine 1981 (pS1981), of Chk1 at serine 345 (pS345), of H2A.X at serine 139 (pS139) and
stabilization of p53 protein levels (Figure 1a). The IR doses were chosen based on preliminary experiments and reflect the high capacity to repair IR-induced DNA damage of MRC-5 cells, which
have been shown to efficiently remove DNA strand breaks (DSBs) up to IR doses of 80 Gy16 and to enter the senescent state upon treatment with various stress stimuli.21 In agreement with
these findings, irradiation of MRC-5 cells with IR doses between 4 and 10 Gy induced a dose-dependent increase and time-dependent decrease of H2A.X pS139 that was mediated by ATM and DNA-PK
(Figures 1b and c). The majority of DSBs was repaired within 8 h post-irradiation as indicated by the reduction of _γ_H2AX foci and the remaining _γ_H2AX foci continued to be repaired 24–48
h following IR treatment (Supplementary Figures S1a and b). The strong reduction in colony formation of single cells after 10 days and the appearance of _β_-galactosidase-positive cells
(MRC-5 and IMR-90) by 72 h after irradiation indicated growth arrest and induction of cell senescence (Supplementary Figures S1c and d), which was further supported by elevated p21 gene
expression as well as p21 and p16 protein levels in MRC-5 and IMR-90 primary fibroblasts (Supplementary Figures S1e–g). Irradiation caused only a slight increase in the G2/M cell cycle
population, but this effect was intensified upon ATM inhibition, suggesting that the efficient DDR prevented a more pronounced effect on cell cycle progression of irradiated MRC-5 cells
(Supplementary Figures S2a–d). Cells did not exhibit elevated apoptosis even at the 8 h time point after 40 Gy irradiation, as indicated by the stable fraction of Annexin V-positive cells
and undetectable expression of NOXA, PUMA and Bax (relative to a significant increase after staurosporine or cycloheximide treatment; Supplementary Figures S3a–d). These results documented
the suitability of 10 and 40 Gy IR to induce non-lethal, but senescence responses in primary fibroblasts. IR INDUCES SIGNIFICANT PROTEOME CHANGES IN MRC-5 FIBROBLASTS To quantify the kinetic
proteome changes upon IR, MRC-5 fibroblasts were harvested at the 0.5, 2, 4 and 8 h time points after IR exposure (10 and 40 Gy) and RPPA analysis was performed (Figure 2a and Supplementary
Figure S4). The time points were chosen based on the observed kinetics of _γ_H2AX activation (Figure 1b) and were similar to those used in earlier studies.7 The protein arrays were probed
with 165 validated antibodies that specifically recognize the activation state of crucial proteins of all major signaling pathways. Only antibodies previously assessed and certified for
commercial RPPA analysis services (identifying only one single band in a conventional western blot) were used and the spotted protein samples were diluted to increase the dynamic range for
protein detection.13 All quantitative RPPA data of three independent biological experiments (eight technical replicates) were subjected to stringent statistical analysis (ANOVA: _P_<0.05
and fold change cutoff: fold change ≥1.5 × S.D.) (Supplementary Figures S5–9). Altogether, 90 unique proteome changes were either significant based on ANOVA and/or passed the 1.5 × S.D.
cutoff (Supplementary Figures S6 and 7 and Supplementary Table). CLUSTERING ANALYSIS IDENTIFIES EARLY CYTOPLASMIC SIGNALS AND SUBSEQUENT ACTIVATION OF CLASSICAL DDR PROTEINS The analysis
described here identified distinct clusters based on the temporal up- and downregulation of specific proteins or protein modifications. In general, treatment of MRC-5 fibroblasts with 40 Gy
induced more distinct clusters as compared with 10 Gy irradiation and three broad groups were apparent (Figures 2b and c and Supplementary Figures S10a and -b). The earliest changes affected
many cytoplasmic proteins such as MEK1/2 (pS217/222, pS221/226), ERK1/2 (pT202/185, pY204/187), LKB (pS428) and cyclin D1 (pT286), which were sharply induced at 0.5 h after irradiation and
relaxed to much lower and constant levels thereafter (cluster 1; Figure 2c and Supplementary Figure S10b). Following this first wave of protein changes, a cluster with a distinct maximum at
2 h after 40 Gy IR appeared (clusters 2 and 5). The protein changes that appeared at later time points after 40 Gy IR comprised changes that were upregulated throughout the entire IR
response or specifically increased by the higher IR dose (clusters 3 and 4). The consecutive activation and inactivation of different groups of proteins indicated a signaling cascade that
progresses from the cytoplasm (early activation of MEK1/2, ERK1/2, LKB) to the nucleus (delayed activation of classical nuclear DDR proteins such as Chk1, p53, p21, MAPKAP2, Hsp27).
VALIDATION OF RPPA ANALYSIS CONFIRMS UPREGULATION OF KNOWN DDR PATHWAYS AND IDENTIFIES PRB DOWNREGULATION The detection of many classical DDR proteome changes upon both 10 and 40 Gy IR
validated the RPPA analysis of IR-treated MRC-5 fibroblasts. Known proteome changes of the DDR pathway included higher total amounts of p53 and p21 as well as increased levels of
phosphorylated p38 MAPK (pT180, pY182), Hsp27 (pS78), p53 (pS15) and Chk1 (pS345) (clusters 1–3 for 10 Gy and clusters 2–5 for 40 Gy; Figures 2b and c and Supplementary Figures S10a and -b).
In addition, Chk1 phosphorylation upon 10 and 40 Gy IR was confirmed by western blot analysis (Figure 1a and Supplementary Figure S10c). Levels of Chk1 phosphorylation determined by RPPA
and western blot analysis showed similar kinetics (early increase after 30 min recovery from IR followed by a decline over the 8 h time course) and thus confirmed the validity of the RPPA
approach. IR-induced Chk2 phosphorylation could only be shown by western blotting because of its poor antibody performance on the arrays (Supplementary Figure S10f). Even though ANOVA
(_P_<0.05) and fold cutoff (S.D.=1.5) analysis did not reveal significantly changed phosphorylation of the classical DDR marker H2A.X (pS139) in response to 10 Gy, at the 2 h time point,
the Student’s _t_-test showed a significantly increased H2A.X phosphorylation (_P_<0.05) (Supplementary Figures S7 and 8). The kinetics of H2A.X phosphorylation upon 10 and 40 Gy
correlated well, but the higher dose resulted in a stronger fold induction (Supplementary Figures S8 and 9). In agreement with previously published studies,22 activation of the cytoplasmic
stress kinase p38 MAPK and its downstream effector, the small heat-shock protein Hsp27, were both ATM-dependent as confirmed by western blotting and ATM inhibitor studies (Supplementary
Figure S10d). Other proteome changes that were also analyzed by western blotting similarly confirmed the RPPA results, albeit western blotting revealed higher fold changes. Temporarily
increased levels of p53, which is the main target for ATM in response to IR,2 correlated with delayed upregulation of p21 (clusters 1 and 2 for 10 Gy; Figure 2b). Surprisingly, the important
cell cycle regulator retinoblastoma protein (pRb) was significantly downregulated upon 10 Gy irradiation (cluster 5; Figure 2b), representing a proteome change that has not been implicated
in the IR response previously. The RPPA-based pRb quantification correlated strongly with results obtained by western blotting (Supplementary Figures S10e and f), supporting _in vivo_ pRb
downregulation upon IR in MRC-5 fibroblasts. In contrast to total pRb levels, the phosphorylation of pRb at S780 (relative to total pRb) increased upon 10 Gy after 8 h and already after 2 h
following irradiation with 40 Gy (Supplementary Figure S10f). In line with this finding, a strong cell-cycle effect was not observed 24–48 h after irradiation compared with a clear
accumulation of cells at the G1/S boundary upon hydroxyurea treatment (Supplementary Figure S2), suggesting that the pRb changes may be important for the maintenance of cell cycle
progression during the immediate IR response. The role of the p53 pathway in DDR and its function as a ‘guardian of the genome’23, 24 is emphasized by the fact that it was significantly
over-represented among the proteins responding to both 10 and 40 Gy IR (Figure 3a), and by its many interactions (more than 14 interactions) in the predicted protein network (Figure 3b and
Supplementary Figure S10g). The agreement of the RPPA analysis with western blot quantifications, as well as the identification of the known key players in the DDR, confirmed the validity of
the RPPA approach described here and proved that the IR-treated cells used in these experiments launched a classical DDR. IR UPREGULATES ANTIAPOPTOTIC AND PROSURVIVAL MARKERS IN MRC-5 CELLS
The kinetic RPPA analysis described here also revealed many new cytoplasmic factors, which were further analyzed in more detail. The here identified changes included prosurvival signals
CREB (pS133) as well as MEK1/2 (pS217/222, pS221/226), ERK1/2 (pT202/185, pY204/187) and AMPK (pT172) for 40 Gy only, antiapoptotic markers (Bad pS112 and pS136, Bcl-2 pS70 for 10 Gy only)
as well as a cluster comprising protein kinase C (PKC) family members. The changes in PKC family members included the phosphorylation of the novel PKC-_δ_ (pT505) and of the atypical
PKC_ζ/λ_ (pT410/403) in the activation loop (Figures 2b and c), which marks the activated state of these two PKC isoforms.25 These analyses suggested that IR treatment of primary fibroblasts
activates not only the classical DDR pathways MEK-ERK and p38-Hsp27 but that also the cytoplasmic PKC signaling and specific antiapoptotic and prosurvival factors were regulated. It is thus
possible that PKC signaling is involved in mediating the high IR resistance of MRC-5 and IMR-90 cells by preventing apoptosis and stimulating prosurvival factors. INHIBITOR SCREEN
IDENTIFIES PKC SIGNALING AS IMPORTANT FOR IR RESISTANCE To elucidate whether PKC or other main signaling cascades regulate cell viability in NHFs upon IR treatment, a radiosensitivity screen
with inhibitors specific for key components of the MEK-ERK, p38, JNK or PKC pathways was performed (Figure 4 and Supplementary Figures S11a–e). While JNK inhibitors had only a weak effect
on cell viability, inhibition of MEK and p38 affected cell proliferation, but independent of IR (i.e., similar effect in treated and untreated cells). Interestingly, already 48 h after the
cotreatment with either one of two PKC inhibitors (GF109203X or Ro-318220) and with IR, MRC-5 cells showed a significant reduction in cell viability compared with cells treated with DMSO
(the solvent) or with IR alone (Figure 4 and Supplementary Figure S11). The NHF strain IMR-90 behaved similarly and showed a significant reduction in growth when treated with the PKC
inhibitor GF109203X (2.5 _μ_M) and either 10 or 40 Gy irradiation (Supplementary Figures S12a–c). These results implicated PKC activity and signaling in the radiosensitivity of MRC-5 and
IMR-90 cells. INHIBITION OR DOWNREGULATION OF PKC DIRECTS CELLS TOWARDS APOPTOSIS To confirm and validate PKC activation upon IR, western blot analyses were performed. In agreement with the
RPPA results and the inhibitor studies, phosphorylation of PKC_ζ/λ_ (pT410/pT403) as well as of PKC_β_ (pT641) were confirmed to be increased upon IR (Figures 5a and b). To further
characterize the mechanism of the PKC-dependent reduction in cell viability, one prosurvival (CREB phosphorylation)26 and one antiapoptotic marker (phosphorylation of Bad)27 was analyzed in
more detail by western blotting (Figures 5c–e). In combination with IR treatment, PKC inhibition or downregulation caused strongly reduced phosphorylation of CREB (pS133) and Bad (pS136),
while the total protein levels were only marginally affected (Figures 5c and d for MRC-5; Figure 5e for IMR-90). Interestingly, ATM (pS1981) was not affected by PKC inhibition (Figures 5c
and e) and did not affect CREB phosphorylation or protein levels (Figure 5c), suggesting that the PKC pathway is activated independent of the classical DDR. Although primary human
fibroblasts are known to undergo senescence as a response to irradiation, the reduced phosphorylation of CREB and Bad, as well as the reduced cell viability, upon IR treatment and PKC
inhibition or downregulation indicated the induction of apoptosis.28, 29 It was therefore assessed whether irradiation and PKC inhibition affects senescence and possibly induces apoptosis.
Upon PKC inhibition and irradiation with 10 or 40 Gy, MRC-5 and IMR-90 cells both showed reduced _β_-galactosidase staining as compared with the DMSO-treated control, which is indicative of
reduced senescence (Figure 5f and Supplementary Figure S13a). Using an alternative senescence marker, reduced p16 protein levels upon irradiation, in combination with PKC inhibition or
downregulation, could be confirmed in MRC-5 cells (Figure 5h). The p16 levels were not significantly reduced in IMR-90 cells in response to IR and PKC inhibition; however; the significant
reduction in p21 protein levels (Supplementary Figures S13b and c) was another alternative indicator for cellular senescence.30 In contrast, in the presence of PKC inhibitors, IR treatment
led to induced levels of the proapoptotic marker Bad and reduced amounts of the antiapoptotic marker Bcl-2, persistently increased p53 protein levels (in addition to the temporary p53
stabilization observed as a response to IR alone) and ARTD1 (PARP-1) cleavage (reduction in ARTD1 full-length levels and accumulation of the 89 kDa cleavage fragment), all suggestive of a
switch from senescence to apoptosis, specifically upon IR in combination with PKC inhibitor treatment or PKC knockdown after 48 h of IR recovery (Figures 5g and h). Reduced ARTD1 levels and
an increased sub-G1 cell population in the NHF strain IMR-90 also confirmed the findings with the MRC-5 strain (Supplementary Figures S13b–f), in addition to the reduced senescence and cell
viability upon IR treatment in combination with PKC inhibition. In summary, these results demonstrated the IR-dependent activation of cytoplasmic PKC signaling and discovered the consecutive
PKC-dependent activation of prosurvival signaling mediated via CREB and Bcl-2 as well as the inactivation of proapoptotic pathways (Bad), which direct normal human MRC-5 and IMR-90
fibroblasts towards senescence and prevent IR-induced apoptosis (Figure 6). Activation of cytoplasmic PKC signaling upon IR is thus a novel mechanism that orchestrates the different
IR-induced responses to ensure cell survival of primary human fibroblasts. DISCUSSION Human primary fibroblasts such as MRC-5 cells are able to repair DNA damage induced by IR doses up to 80
Gy.16, 17 However, it was so far not clear how MRC-5 fibroblasts prevent apoptosis, which is induced at much lower IR doses in other cell types.31 Understanding the dynamics of _in vivo_
signaling events in response to IR in human primary fibroblast may thus reveal medically relevant IR resistance mechanisms. The network analysis of IR-induced proteome changes in MRC-5
fibroblasts presented here identified upregulation of known DDR factors (H2AX, p53, p21, MKK3/6, p38, MK2, Hsp27, Chk2) only 2–8 h after irradiation (Supplementary Table). In contrast,
growth factor- and cytokine-dependent signaling pathways including members of the MAPK family (MEK/ERK), PKC family (PKC_δ_, PKC_ζ/λ_, PKC_β_II) as well as anti- and proapoptotic Bcl-2
family members (Bcl-2, Bad) tended to respond with faster kinetics and thus indicated that cytoplasmic signaling events are upstream of the canonical DDR. Indeed, an increasing body of
evidence implicates an IR-dependent cytoplasmic signaling network in regulating DNA break repair.32 Although RPPA analysis is restricted by antibody performance and reduced dynamic range,
western blotting confirmed the tested proteome changes discovered by RPPA analysis, supporting the stringent statistical analysis. However, western blotting indicated that RPPA analysis
usually underestimated the IR-induced proteome changes. Most proteome changes, in particular upon 40 Gy IR, affected mitogenic signaling events controlling cell survival (MKK3/6-p38-MK2
pathway, MEK-ERK pathway, LKB-AMPK pathway, PKC-Bad pathway). Previously published results have demonstrated p38-MK2 pathway activation and subsequent cell cycle arrest in G2/M in response
to UV irradiation, thus protecting DNA damaged cell from entering mitosis, which would lead to a mitotic catastrophe and eventually to apoptosis.33, 34 In agreement with these findings, in
response to 10 and even more pronounced upon 40 Gy, we could show activation of the major p38-MK2 pathway components, with phosphorylation of MKK3/6, p38, MAPKAP-2 (MK2) and Hsp27, besides
the slight increase in the percentage of the G2/M cell cycle population 24–48 h after IR recovery (Supplementary Figures S2 and S7). The most important finding of this systematic RPPA
analysis is the identification of PKC as a key player that orchestrates the downstream signaling pathways regulating apoptosis and cell survival (Figure 6). IR-dependent PKC activation
regulated Bad, Bcl-2 and CREB to prevent apoptosis and to induce prosurvival signaling. Whether PKC family members directly phosphorylate the identified downstream proteins or rather
indirectly affect their activation is currently not known. Interestingly, PKCs are known to translocate to the nucleus35 and all identified regulatory components (Bad, Bcl-2, CREB) contain
putative target sites for phosphorylation by PKC.36 PKC inhibitors, or PKC downregulation before IR treatment, downregulated antiapoptotic factors (Bad phosphorylation, Bcl-2) and induced
apoptosis (ARTD1 cleavage, increased p53 stabilization, Bad upregulation, increased sub-G1 population and decline in cell number). Sensitization of primary fibroblasts by PKC inhibitors or
downregulation thus induced an apoptotic program that is mediated by effects on CREB, Bad and proapoptotic genes. PKC-dependent regulation of cell survival, apoptosis as well as cell cycle
progression is thus proposed as a part of the mechanism by which primary fibroblasts resist high IR doses and circumvent apoptosis, which is in agreement with the high DNA repair efficiency
and the PKC-dependent regulation of p53 function.16, 37 The inhibitor and siRNA experiments described here imply PKC signaling in the prevention of apoptosis in human primary MRC-5 and
IMR-90 fibroblasts. However, these analyses do not identify which PKC family member mediates the observed phenotypes. The RPPA analysis identified PKC_δ_ activation, which was previously
shown to be induced upon DNA damage38 and linked to pro- and antiapoptotic functions (Basu and Pal39 and many reference therein). Future studies on the upstream regulators relevant for PKC
activation upon IR in MRC-5 and IMR-90 cells will identify the required cofactors and signaling components and thereby indicate which PKC subgroup mediates the antiapoptotic function. Taken
together, our findings provide the cellular mechanism, which is responsible for this phenotype and may thus open up possiblities for new treatment schemes of highly IR-resistant tumors. This
result has not only important implications for our understanding of the IR response but also for the comprehension of nuclear regulatory events and supports the concept of the cellular, in
contrast to the nuclear, radiation response.40 Cellular stress such as IR is perceived at the plasma membrane and in the cytoplasm and initiates cytoplasmic signaling pathways that
consecutively control nuclear events such as DNA repair. This is also suggested by the induction of IR-induced signaling in cells exposed to IR conditioned medium, which is termed the
‘bystander effect’.41 The classical DDR, comprised of nuclear events regulating cell cycle progression and DNA repair, is thus preceded and controlled by the cytoplasmic radiation response,
which is mediated by the PKC signaling pathway as well as other signaling pathways such as the MEK-ERK pathway. This RPPA network analysis of IR-induced signaling identified a new,
cytoplasmic, PKC-dependent regulatory mechanism that conditions stressed cells by preventing apoptosis. The PKC-dependent induction of prosurvival signaling and evasion of apoptosis is thus
a mechanism, which renders primary human fibroblasts resistant to high doses of IR, and may thereby protect the microenvironment and permit survival of tumors. Our results thus provide an
explanation for the cellular response to severe irradiation stress and will therefore help optimize and improve radiotherapy. MATERIALS AND METHODS CELL CULTURE, IR TREATMENT, SIRNA
TRANSFECTION, LYSIS, VIABILITY AND SENESCENCE ASSAYS The MRC-5 and IMR-90 human lung fibroblast cell strains42, 43 were obtained from the American Type Culture Collection and cultured in
supplemented MEM (Invitrogen; Life Technologies, Carlsbad, CA, USA). Cells were exposed to IR using an X-ray generator (Pantak Seifert X-ray System, Ahrensburg, Germany; 120 kV; 19 mA;
aluminum filter, 3.11 Gy/min) and recovered for different time periods. Inhibitor treatment was performed as indicated in the figure legends before the exposure to IR. To reduce PKC
expression, 1 × 105 MRC-5 cells were transfected using human siPKC pan (Santa Cruz Biotechnology, Dallas, TX, USA; sc-29449) over 3 and 4 days, before irradiation. Whole cell extracts were
prepared with Zeptosens Cell Lysis Buffer CLB1 (Bayer Technology Services GmbH, Leverkusen, Germany) or RIPA lysis buffer and total protein concentration was determined using the standard
Lowry method. Cell viability was determined by seeding 2 × 103 cells in 96-well plates overnight before irradiation or drug treatment. Cell viability was quantified using the WST-1
proliferation reagent (Roche, Basel, Switzerland) or alamarBlue cell viability assay (Invitrogen) and a plate reader (Tecan, Männedorf, Switzerland). The clonogenic and senescence assays
were performed as described elsewhere.44, 45 REVERSE PHASE PROTEIN ARRAYS RPPA were prepared as described.46 In brief, whole cell extracts were spotted onto hydrophobically coated Zeptosens
Chips (Bayer Technology Services GmbH). Serially diluted lysates (100, 75, 50 and 25%) were arrayed in duplicates onto hydrophobic Zeptosens Chips using the Nanoplotter NP2.0 (GeSiM;
Gesellschaft für Silizium-Mikrosysteme mbH, Grosserkmannsdorf, Germany), followed by blocking in an ultrasonic nebulizer (ZeptoFOG; Bayer Technology Services GmbH). Antibody incubation,
microarray data acquisition (ZeptoREADER; Bayer Technology Services GmbH) and data analysis (ZeptoVIEW version 3.1.0.2; Bayer Technology Services GmbH) was performed exactly as described.47
The eight data points (100, 75, 50 and 25% lysate amount in duplicates) were fitted using a weighted linear least-squares fit48 and the relative fluorescence intensity determined by
interpolating at the median protein concentration or modification. To correct for small variations in protein content, relative intensities were normalized to the signals of _β_-catenin,
which did not show any significant variation (ANOVA, _P_<0.05; 1.5 × S.D.) in response to IR over indicated time points. SIGNIFICANCE AND CLUSTERING ANALYSIS To identify significant
proteome changes in response to IR, relative fluorescence intensities were imported to MeV version 4.6.49 Relative fluorescence intensities were log 2 transformed and normalized, before
performing statistical analysis using one-way ANOVA as described in Zar.50 The mean transformed fluorescence intensities for group 1 (untreated: 0.5, 2, 4 and 8 h), group 2 (biological
triplicates of 10 or 40 Gy at 0.5 h), group 3 (biological triplicates of 10 or 40 Gy at 2 h), group 4 (biological triplicates of 10 or 40 Gy at 4 h) and group 5 (biological triplicates of 10
or 40 Gy at 8 h) were compared using F-statistics with _P_<0.05. Owing to technical problems during preparation of the serial dilution of the whole cell extracts, two biological
replicates for the 8 h time point of 10 Gy irradiated samples (10 Gy at 8 h) and one biological replicate for the 2 and 8 h time point of 40 Gy irradiated samples (40 Gy at 2 h and 40 Gy at
8 h) were excluded from the statistical analysis. For fold-change analysis, transformed means of the biological replicates were normalized to the untreated sample (set as 1) and proteome
changes were filtered (cutoff set at 1.5 × S.D. equal to log 2>0.27/<−0.27 for 10 Gy or log 2>0.29/<−0.29 for 40 Gy). Significant proteome changes in response to IR were
selected, if significant by one-way ANOVA (_P_<0.05) and fold cutoff (1.5 × S.D.). Alternatively, significance analysis was performed using two-tailed Student’s _t_-test (_P_<0.05).
Similar profiles of the fold changes over time were identified by clustering analysis using the Self Organizing Tree Algorithm51 and default parameters of MeV version 4.6.49 PATHWAY AND
NETWORK ANALYSIS Gene pathway membership data were obtained from protein interaction database, PID52 and KEGG.53 A total of 200 and 211 pathways were obtained from PID and KEGG,
respectively. For statistical analysis, all analyzed proteins in this study (unique IDs) were set as the background list and all pathways consisting of more than five proteins from the
background list were considered for statistical analysis by Fisher’s exact test, resulting in a total of 93 pathways from PID and 63 from KEGG. Fisher’s exact test was performed to identify
pathways significantly affected by proteins (modifications) altered in response to irradiation (significant by ANOVA and fold change) using the R statistical framework.54 To account for
multiple testing, _P_-values were corrected for false discovery rates (FDRs) using Benjamini–Hochberg correction. We have used an FDR-corrected _P-_value cutoff of 0.1 to identify pathways
significantly affected by proteome changes. Significant proteome changes, identified by statistical (ANOVA) and fold-change analysis (log 2 cutoff) were subjected to protein–protein
interaction analysis using STRING (v. 9.0, http://www.string-db.org/).55 Only interactions with a STRING score of 0.7 and above were further analyzed using Cytoscape
(http://www.cytoscape.org/).56 IMMUNOBLOTTING For western blot analysis, proteins were separated by SDS-PAGE gel electrophoresis and bands were visualized by using either horseradish
peroxidase-conjugated antibodies (1 : 5000; GE Healthcare, Life Sciences, Uppsala, Sweden) and ECL detection (GE Healthcare) or IR-dye-conjugated antibodies (1 : 15 000; LI-COR Biosciences)
and detection by the Odyssey infrared imaging system (LI-COR Biosciences, Lincoln, NE, USA). For quantification, bands were analyzed by ImageJ 1.46 (ref. 57) and the Odyssey imaging software
(LI-COR Biosciences). Antibodies used for western blotting were anti-ATM (GeneTex, Irvine, CA, USA), anti-ATM Phospho (pS1981) (Epitomics-an Abcam Company, Burlingame, CA, USA), anti-Bad
(CST), anti-Bad Phospho (pS136) (Cell Signaling Technology (CST), Danvers, MA, USA), anti-Chk1 (CST), anti-Chk1 Phospho (pS345) (1 : 500; CST), anti-Chk2 Phospho (pT68) (CST), anti-CREB
(CST), anti-CREB Phospho (pS133)/anti-ATF-1 (phospho) (CST), anti-histone H2A.X Phospho (pS139) (Millipore, Billerica, MA, USA), anti-Hsp27 (CST), anti-Hsp27 Phospho (pS78) (1 : 500; CST),
anti-PARP1 (Santa Cruz Biotechnology), anti-PARP1 cleaved (1 : 500; CST), anti-p16 (1 : 500; Santa Cruz Biotechnology), anti-p21 (Santa Cruz Biotechnology), anti-p38 (CST), anti-p38 Phospho
(pThr180/Tyr182), anti-p44/42 Erk1/2 (CST), anti-p44/42 Erk1/2 Phospho (pThr202/Tyr204) (CST), anti-p53 (Santa Cruz Biotechnology), anti-Rb (1 : 500; Epitomics), anti-Rb (pS780) (CST),
anti-tubulin (1 : 10 000; Sigma-Aldrich, St Louis, MO, USA), anti-PKC_β_II Phospho (pT641) (CST), anti-PKC_δ_ (CST), anti-PKC_ζ/λ_ (CST) and anti-PKC_ζ/λ_ Phospho (pT410/pT403) (CST). Unless
otherwise stated, antibody dilution was 1 : 1000. IMMUNOFLUORESCENCE MICROSCOPY MRC-5 cells grown on cover slips over night (≈1 × 104 cells) were irradiated and immunohistochemically
stained with primary (1 : 500 mouse anti-histone H2A.X Phospho (S139) immunoglobulin G1 (IgG1) (Millipore) or 1 : 500 rabbit anti-53BP1 IgG1 (Santa Cruz Biotechnology)) and secondary
antibodies (1 : 250 FITC- or cyanine 3-conjugated IgG anti-mouse IgG (Jackson ImmunoResearch Laboratories, West Grove, PA, USA) or 1 : 250 Alexa Fluor 488-conjugated anti-rabbit IgG
(Invitrogen)). FLOW CYTOMETRY Cell cycle analysis of IR-treated or -untreated MRC-5 cells was performed using standard ethanol fixation/PI-staining protocol and flow cytometry analysis
(FACS) with a Dako CyAn ADP flow cytometer (Dako, North America, Carpinteria, CA, USA). For sub-G1 peak analysis of IMR-90, cell debris and necrotic cells were gated out according to the
protocol of Riccardi and Nicoletti.58 Annexin V staining was performed using the FITC Annexin V apoptosis detection kit (BD Biosciences, San Jose, CA, USA) and a Dako CyAn ADP flow cytometer
(Dako). RNA EXTRACTION AND REAL-TIME PCR ANALYSIS Total RNA was reverse transcribed using High-Capacity cDNA Reverse Transcription kit (Applied Biosystems; Life Technologies). Real-time PCR
was performed using SYBR green premixed buffer and analyzed by the Rotor-Gene Q cycler (Qiagen, Hilden, Germany). ABBREVIATIONS * CREB: cAMP response element-binding protein * DDR: DNA
damage response * DNA: deoxyribonucleic acid * IgG: immunoglobulin G * IR: ionizing radiation * NHF: normal human fibroblast * PKC: protein kinase C * RPPA: reverse phase protein array
REFERENCES * Harper JW, Elledge SJ . The DNA damage response: ten years after. _Mol Cell_ 2007; 28: 739–745. Article CAS PubMed Central Google Scholar * Shiloh Y . ATM and related
protein kinases: safeguarding genome integrity. _Nate Rev Cancer_ 2003; 3: 155–168. Article CAS Google Scholar * Bonner WM, Redon CE, Dickey JS, Nakamura AJ, Sedelnikova OA, Solier S _et
al_. GammaH2AX and cancer. _Nat Rev Cancer_ 2008; 8: 957–967. Article CAS PubMed Central Google Scholar * Surova O, Zhivotovsky B . Various modes of cell death induced by DNA damage.
_Oncogene_ 2012 E-pub ahead of print; doi: 10.1038/onc.2012.556. Article PubMed Central Google Scholar * Roos WP, Kaina B . DNA damage-induced cell death by apoptosis. _Trends Mol Med_
2006; 12: 440–450. Article CAS PubMed Central Google Scholar * Sabin RJ, Anderson RM . Cellular senescence – its role in cancer and the response to ionizing radiation. _Genome Integr_
2011; 2: 7. Article CAS PubMed Central Google Scholar * Bennetzen M, Larsen D, Bunkenborg J, Bartek J, Lukas J, Andersen J . Site-specific phosphorylation dynamics of the nuclear
proteome during the DNA damage response. _Mol Cell Proteomics_ 2010; 9: 1314–1323. Article CAS PubMed Central Google Scholar * Bensimon A, Schmidt A, Ziv Y, Elkon R, Wang SY, Chen DJ _et
al_. ATM-dependent and -independent dynamics of the nuclear phosphoproteome after DNA damage. _Sci Signal_ 2010; 3: rs3. Article CAS PubMed Central Google Scholar * Beli P, Lukashchuk
N, Wagner SA, Weinert BT, Olsen JV, Baskcomb L _et al_. Proteomic investigations reveal a role for RNA processing factor THRAP3 in the dna damage response. _Mol Cell_ 2012; 46: 212–225.
Article CAS PubMed Central Google Scholar * Lee MJ, Ye AS, Gardino AK, Heijink AM, Sorger PK, MacBeath G _et al_. Sequential application of anticancer drugs enhances cell death by
rewiring apoptotic signaling networks. _Cell_ 2012; 149: 780–794. Article CAS PubMed Central Google Scholar * Tentner AR, Lee MJ, Ostheimer GJ, Samson LD, Lauffenburger DA, Yaffe MB .
Combined experimental and computational analysis of DNA damage signaling reveals context-dependent roles for Erk in apoptosis and G1/S arrest after genotoxic stress. _Mol Syst Biol_ 2012; 8:
568. Article PubMed Central Google Scholar * van Oostrum J, Voshol H . Antibody-based proteomics to study cellular signalling networks. _Eur Pharmaceut Rev_ 2008; 2: 31–35. Google
Scholar * Paweletz CP, Charboneau L, Bichsel VE, Simone NL, Chen T, Gillespie JW _et al_. Reverse phase protein microarrays which capture disease progression show activation of pro-survival
pathways at the cancer invasion front. _Oncogene_ 2001; 20: 1981–1989. Article CAS PubMed Central Google Scholar * Nishizuka S, Ramalingam S, Spurrier B, Washburn FL, Krishna R,
Honkanen P _et al_. Quantitative protein network monitoring in response to DNA damage. _J Proteome Res_ 2008; 7: 803–808. Article CAS PubMed Central Google Scholar * Hudson ME,
Pozdnyakova I, Haines K, Mor G, Snyder M . Identification of differentially expressed proteins in ovarian cancer using high-density protein microarrays. _Proc Natl Acad Sci USA_ 2007; 104:
17494–17499. Article CAS PubMed Central Google Scholar * Kuhne M, Riballo E, Rief N, Rothkamm K, Jeggo PA, Lobrich M . A double-strand break repair defect in ATM-deficient cells
contributes to radiosensitivity. _Cancer Res_ 2004; 64: 500–508. Article PubMed Central Google Scholar * Lobrich M, Shibata A, Beucher A, Fisher A, Ensminger M, Goodarzi AA _et al_.
gammaH2AX foci analysis for monitoring DNA double-strand break repair: strengths, limitations and optimization. _Cell Cycle_ 2010; 9: 662–669. Article PubMed Central Google Scholar *
Yoshida K . Role for PKCδ on apoptosis in the DNA damage response In: Chen CC (eds). _Selected Tpics in DNA Repair_. InTech: San Diego, USA, 2011 pp 293–304. Google Scholar * Newton AC .
Protein kinase C: poised to signal. _Am J Physiol Endocrinol Metab_ 2010; 298: E395–E402. Article CAS PubMed Central Google Scholar * Jackson DN, Foster DA . The enigmatic protein kinase
Cdelta: complex roles in cell proliferation and survival. _FASEB J_ 2004; 18: 627–636. Article CAS PubMed Central Google Scholar * Kosar M, Bartkova J, Hubackova S, Hodny Z, Lukas J,
Bartek J . Senescence-associated heterochromatin foci are dispensable for cellular senescence, occur in a cell type- and insult-dependent manner and follow expression of p16(ink4a). _Cell
Cycle_ 2011; 10: 457–468. Article CAS PubMed Central Google Scholar * Cosentino C, Grieco D, Costanzo V . ATM activates the pentose phosphate pathway promoting anti-oxidant defence and
DNA repair. _EMBO J_ 2011; 30: 546–555. Article CAS PubMed Central Google Scholar * Meek DW . Tumour suppression by p53: a role for the DNA damage response? _Nat Rev Cancer_ 2009; 9:
714–723. Article CAS PubMed Central Google Scholar * Lavin MF, Gueven N . The complexity of p53 stabilization and activation. _Cell Death Differ_ 2006; 13: 941–950. Article CAS PubMed
Central Google Scholar * Parekh DB, Ziegler W, Parker PJ . Multiple pathways control protein kinase C phosphorylation. _EMBO J_ 2000; 19: 496–503. Article CAS PubMed Central Google
Scholar * Lonze BE, Riccio A, Cohen S, Ginty DD . Apoptosis, axonal growth defects, and degeneration of peripheral neurons in mice lacking CREB. _Neuron_ 2002; 34: 371–385. Article CAS
PubMed Central Google Scholar * Datta SR, Dudek H, Tao X, Masters S, Fu H, Gotoh Y _et al_. Akt phosphorylation of BAD couples survival signals to the cell-intrinsic death machinery.
_Cell_ 1997; 91: 231–241. Article CAS Google Scholar * Adams JM, Cory S . The Bcl-2 apoptotic switch in cancer development and therapy. _Oncogene_ 2007; 26: 1324–1337. Article CAS
PubMed Central Google Scholar * Vogler M, Dinsdale D, Dyer MJ, Cohen GM . Bcl-2 inhibitors: small molecules with a big impact on cancer therapy. _Cell Death Differ_ 2009; 16: 360–367.
Article CAS PubMed Central Google Scholar * Lawless C, Wang C, Jurk D, Merz A, Zglinicki T, Passos JF . Quantitative assessment of markers for cell senescence. _Exp Gerontol_ 2010; 45:
772–778. Article CAS Google Scholar * Torudd J, Protopopova M, Sarimov R, Nygren J, Eriksson S, Markova E _et al_. Dose–response for radiation-induced apoptosis, residual 53BP1 foci and
DNA-loop relaxation in human lymphocytes. _Int J Radiat Biol_ 2005; 81: 125–138. Article CAS PubMed Central Google Scholar * Meyn RE, Munshi A, Haymach JV, Milas L, Ang KK . Receptor
signaling as a regulatory mechanism of DNA repair. _Radiother Oncol_ 2009; 92: 316–322. Article CAS PubMed Central Google Scholar * Bulavin DV, Higashimoto Y, Popoff IJ, Gaarde WA,
Basrur V, Potapova O _et al_. Initiation of a G2/M checkpoint after ultraviolet radiation requires p38 kinase. _Nature_ 2001; 411: 102–107. Article CAS PubMed Central Google Scholar *
Manke IA, Nguyen A, Lim D, Stewart MQ, Elia AE, Yaffe MB . MAPKAP kinase-2 is a cell cycle checkpoint kinase that regulates the G2/M transition and S phase progression in response to UV
irradiation. _Mol Cell_ 2005; 17: 37–48. Article CAS PubMed Central Google Scholar * Martelli AM, Evangelisti C, Nyakern M, Manzoli FA . Nuclear protein kinase C. _Biochim Biophys Acta_
2006; 1761: 542–551. Article CAS PubMed Central Google Scholar * Xue Y, Ren J, Gao X, Jin C, Wen L, Yao X . GPS 2.0, a tool to predict kinase-specific phosphorylation sites in hierarchy.
_Mol Cell Proteomics_ 2008; 7: 1598–1608. Article CAS PubMed Central Google Scholar * Yoshida K . Protein kinase C, p53, and DNA damage In: Kazanietz MG (eds). _Protein Kinase C in
Cancer Signaling and Therapy_. Springer: Berlin, 2010 pp 253–265. Chapter Google Scholar * Yoshida K, Miki Y, Kufe D . Activation of SAPK/JNK signaling by protein kinase Cdelta in response
to DNA damage. _J Biol Chem_ 2002; 277: 48372–48378. Article CAS PubMed Central Google Scholar * Basu A, Pal D . Two faces of protein kinase Cdelta: the contrasting roles of PKCdelta in
cell survival and cell death. _Scientific World J_ 2010; 10: 2272–2284. Article CAS Google Scholar * Schmidt-Ullrich RK, Dent P, Grant S, Mikkelsen RB, Valerie K . Signal transduction
and cellular radiation responses. _Radiat Res_ 2000; 153: 245–257. Article CAS PubMed Central Google Scholar * Baskar R, Balajee AS, Geard CR, Hande MP . Isoform-specific activation of
protein kinase c in irradiated human fibroblasts and their bystander cells. _Int J Biochem Cell Biol_ 2008; 40: 125–134. Article CAS Google Scholar * Jacobs JP, Jones CM, Baille JP .
Characteristics of a human diploid cell designated MRC-5. _Nature_ 1970; 227: 168–170. Article CAS PubMed Central Google Scholar * Nichols WW, Murphy DG, Cristofalo VJ, Toji LH, Greene
AE, Dwight SA . Characterization of a new human diploid cell strain, IMR-90. _Science_ 1977; 196: 60–63. Article CAS PubMed Central Google Scholar * Franken NA, Rodermond HM, Stap J,
Haveman J, van Bree C . Clonogenic assay of cells _in vitro_. _Nat Protoc_ 2006; 1: 2315–2319. Article CAS PubMed Central Google Scholar * Dimri GP, Lee X, Basile G, Acosta M, Scott G,
Roskelley C _et al_. A biomarker that identifies senescent human cells in culture and in aging skin _in vivo_. _Proc Natl Acad Sci USA_ 1995; 92: 9363–9367. Article CAS PubMed Central
Google Scholar * Pawlak M, Schick E, Bopp MA, Schneider MJ, Oroszlan P, Ehrat M . Zeptosens’ protein microarrays: a novel high performance microarray platform for low abundance protein
analysis. _Proteomics_ 2002; 2: 383–393. Article CAS PubMed Central Google Scholar * Voshol H, Ehrat M, Traenkle J, Bertrand E, van Oostrum J . Antibody-based proteomics: analysis of
signaling networks using reverse protein arrays. _FEBS J_ 2009; 276: 6871–6879. Article CAS PubMed Central Google Scholar * Bevington PR . _Data Reduction and Error Analysis for the
Physical Sciences_ 3rd edn McGraw-Hill: New York, NY, 2002 p 352. Google Scholar * Saeed AI, Sharov V, White J, Li J, Liang W, Bhagabati N _et al_. TM4: a free, open-source system for
microarray data management and analysis. _Biotechniques_ 2003; 34: 374–378. Article CAS PubMed Central Google Scholar * Zar JH . _Biostatistical Analysis_ 5th edn Prentice-Hall: Upper
Saddle River, NJ, 2009 p 960. Google Scholar * Herrero-Yraola A, Bakhit SM, Franke P, Weise C, Schweiger M, Jorcke D _et al_. Regulation of glutamate dehydrogenase by reversible
ADP-ribosylation in mitochondria. _EMBO J_ 2001; 20: 2404–2412. Article CAS PubMed Central Google Scholar * Schaefer CF, Anthony K, Krupa S, Buchoff J, Day M, Hannay T _et al_. PID: the
Pathway Interaction Database. _Nucleic Acids Res_ 2009; 37: D674–D679. Article CAS PubMed Central Google Scholar * Kanehisa M, Goto S, Furumichi M, Tanabe M, Hirakawa M . KEGG for
representation and analysis of molecular networks involving diseases and drugs. _Nucleic Acids Res_ 2010; 38: D355–D360. Article CAS PubMed Central Google Scholar * Ihaka R, Genleman RR
. A language for data analysis and graphics. _J Comput Graph Stat_ 1996; 5: 299–314. Google Scholar * Szklarczyk D, Franceschini A, Kuhn M, Simonovic M, Roth A, Minguez P _et al_. The
STRING database in 2011: functional interaction networks of proteins, globally integrated and scored. _Nucleic Acids Res_ 2011; 39: D561–D568. Article CAS Google Scholar * Shannon P,
Markiel A, Ozier O, Baliga NS, Wang JT, Ramage D _et al_. Cytoscape: a software environment for integrated models of biomolecular interaction networks. _Genome Res_ 2003; 13: 2498–2504.
Article CAS PubMed Central Google Scholar * Schneider CA, Rasband WS, Eliceiri KW . NIH Image to ImageJ: 25 years of image analysis. _Nat Methods_ 2012; 9: 671–675. Article CAS PubMed
Central Google Scholar * Riccardi C, Nicoletti I . Analysis of apoptosis by propidium iodide staining and flow cytometry. _Nat Protoc_ 2006; 1: 1458–1461. Article CAS PubMed Central
Google Scholar Download references ACKNOWLEDGEMENTS F Freimoser (University of Zurich) provided editorial assistance and critical input during the writing. We thank M Ehrat, G Balciunaite
and J Grognux for technical assistance with protein arrays. This work was supported in part by the Kanton of Zurich (to MOH), Oncosuisse (KLS 02396-02-2009) and the UBS foundation. AUTHOR
CONTRIBUTIONS AB, NK, JT and MOH designed the experiments; AB, NK and KM performed and analyzed the experiments; and JvO, HR, MB and MOH supervised the study. All authors contributed to the
preparation of the manuscript. AUTHOR INFORMATION AUTHORS AND AFFILIATIONS * Institute of Veterinary Biochemistry and Molecular Biology (IVBMB), University of Zurich, Winterthurerstrasse
190, Zurich, Switzerland A Bluwstein, K Léger & M O Hottiger * Cancer Biology PhD Program, Life Science Zurich Graduate School, University of Zurich, Winterthurerstrasse 190, Zürich,
Switzerland A Bluwstein & K Léger * Institute for Molecular Life Science (IMLS) and Swiss Institute of Bioinformatics (SIB), University of Zurich, Winterthurerstrasse 190, Zurich,
Switzerland N Kumar & M Baudis * Bayer Technology Services GmbH, Zeptosens Platform, Leverkusen, Germany J Traenkle * Luxembourg Clinical Proteomics Center, CRP-Santé, Strassen,
Luxembourg J van Oostrum * Functional Genomics Center Zurich (FGCZ), University of Zurich, Zurich, Switzerland H Rehrauer Authors * A Bluwstein View author publications You can also search
for this author inPubMed Google Scholar * N Kumar View author publications You can also search for this author inPubMed Google Scholar * K Léger View author publications You can also search
for this author inPubMed Google Scholar * J Traenkle View author publications You can also search for this author inPubMed Google Scholar * J van Oostrum View author publications You can
also search for this author inPubMed Google Scholar * H Rehrauer View author publications You can also search for this author inPubMed Google Scholar * M Baudis View author publications You
can also search for this author inPubMed Google Scholar * M O Hottiger View author publications You can also search for this author inPubMed Google Scholar CORRESPONDING AUTHOR
Correspondence to M O Hottiger. ETHICS DECLARATIONS COMPETING INTERESTS JT was Zeptosens Technology Manager at Bayer Technology Services GmbH and is currently Group Head for Process
Analytical Technologies at the same company. JvO was Head of Business Development at Zeptosens, a division of Bayer (Schweiz) AG. All the other authors declare no conflict of interest.
ADDITIONAL INFORMATION Edited by A Stephanou Supplementary Information accompanies the paper on Cell Death and Disease website SUPPLEMENTARY INFORMATION SUPPLEMENTARY FIGURES (PDF 5930 KB)
SUPPLEMENTARY INFORMATION (XLS 282 KB) RIGHTS AND PERMISSIONS This work is licensed under the Creative Commons Attribution-NonCommercial-No Derivative Works 3.0 Unported License. To view a
copy of this license, visit http://creativecommons.org/licenses/by-nc-nd/3.0/ Reprints and permissions ABOUT THIS ARTICLE CITE THIS ARTICLE Bluwstein, A., Kumar, N., Léger, K. _et al._ PKC
signaling prevents irradiation-induced apoptosis of primary human fibroblasts. _Cell Death Dis_ 4, e498 (2013). https://doi.org/10.1038/cddis.2013.15 Download citation * Received: 26
September 2012 * Revised: 21 December 2012 * Accepted: 02 January 2013 * Published: 14 February 2013 * Issue Date: February 2013 * DOI: https://doi.org/10.1038/cddis.2013.15 SHARE THIS
ARTICLE Anyone you share the following link with will be able to read this content: Get shareable link Sorry, a shareable link is not currently available for this article. Copy to clipboard
Provided by the Springer Nature SharedIt content-sharing initiative KEYWORDS * apoptosis * DNA damage response * PKC signaling * primary human fibroblast * radiation sensitivity * reverse
phase protein array