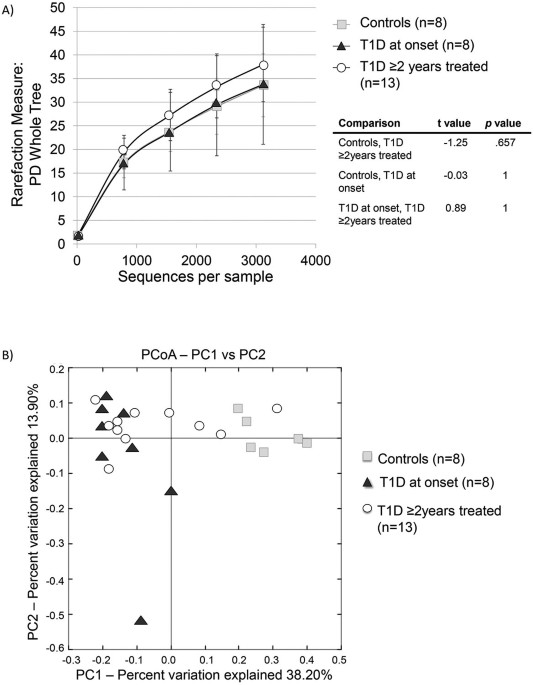
- Select a language for the TTS:
- UK English Female
- UK English Male
- US English Female
- US English Male
- Australian Female
- Australian Male
- Language selected: (auto detect) - EN
Play all audios:
ABSTRACT Dysbiosis of the intestinal microbiota affecting the gut barrier could be triggering Type 1 Diabetes (T1D), the second most frequent autoimmune disease in childhood. This study
compared the structure of the fecal microbiota in 29 mestizo children aged 7–18 years, including 8 T1D at onset, 13 T1D after 2 years treatment and 8 healthy controls. Clinical information
was collected, predisposing haplotypes were determined; the fecal DNA was extracted, the V4 region of the 16S rRNA gene amplified and 454-pyrosequenced. The newly diagnosed T1D cases had
high levels of the genus _Bacteroides_ (p < 0.004), whereas the control group had a gut microbiota dominated by _Prevotella_. Children with T1D treated for ≥2 years had levels of
_Bacteroides_ and _Prevotella_ compared to those of the control group. The gut microbiota of newly diagnosed T1D cases is altered, but whether it is involved in disease causation or is a
consequence of host selection remains unclear. SIMILAR CONTENT BEING VIEWED BY OTHERS FUNCTIONAL AND METABOLIC ALTERATIONS OF GUT MICROBIOTA IN CHILDREN WITH NEW-ONSET TYPE 1 DIABETES
Article Open access 26 October 2022 CHARACTERIZATION OF THE GUT BACTERIAL AND VIRAL MICROBIOTA IN LATENT AUTOIMMUNE DIABETES IN ADULTS Article Open access 09 April 2024 ISLET AUTOANTIBODY
SEROCONVERSION IN TYPE-1 DIABETES IS ASSOCIATED WITH METAGENOME-ASSEMBLED GENOMES IN INFANT GUT MICROBIOMES Article Open access 21 June 2022 INTRODUCTION Type 1 Diabetes (T1D) is a
pro-inflammatory autoimmune disorder that results in β-cells destruction, leading to insulin deficiency1. T1D is one of the most frequent autoimmune disorders in childhood, with a worldwide
prevalence of 1:200 to 1:300, which is higher than that reported a few decades ago2. The increasing incidence cannot be explained only by host genetic factors (HLA DR4-DQ8 and DR3-DQ2)3 and
could instead reflect changes in early childhood exposure to risk factors4, such as lack of breastfeeding5 and viral infections6. Reported protection factors include microbial exposure7,8
and selective antibiotic treatments in early life7,9. Associations between the intestinal microbiota alterations and B-cell autoimmunity have been described in HLA-DR/DQ genetically
predisposed humans with positive GAD/IA2 autoantibodies10,11 or with T1D diagnosis12. Autoimmunity can be triggered after the translocation of microbes through the intestinal epithelium as a
result of increased permeability13. Interestingly, the pancreas and gut endoderm share a common embryonic origin, which may create an immune link between both organs14. The aim of this
study was to evaluate the structure of the gut microbiota in genetically predisposed Mexican children with T1D at onset and after treatment for more than 2 years. RESULTS STUDY POPULATION
All subjects were Sonoran by birth, with at least 90% of the parents and grandparents born in northwest Mexico. A total of 21 T1D cases and 8 healthy controls were included. Eight cases were
newly diagnosed T1D cases (less than 2 months evolution) and 13 were controlled with insulin treatment (with more than 2 years evolution [T1D treated for ≥2 years]) and HbA1C levels <
8%. Four subjects in the control group were siblings of T1D cases. The subjects' mean age for the 3 groups was statistically similar (Table S1). CLINICAL INFORMATION The number of
antibiotic treatments per year, previous to diagnosis, was significantly higher (_p_ = 0.043) in the T1D cases when compared with the antibiotic frequency in the same period in the controls
(Table S1). The mean of antibiotic cycles per year in the newly diagnosed T1D cases was 4 cycles, while the T1D treated for more >2 year treatment group and controls had 6 and 3 cycles
per year, respectively. At the time of the study, all subjects were on antibiotic-free scheme for at least 3 months prior to sampling. Other variables such as delivery mode, breastfeeding
time, lactation regimes and infections per year, were not statistically different between controls and T1D cases (_p_ ≥ 0.05). HLA TYPES All of the T1D cases (Table S1) had at least one HLA
risk allele. The HLA DR3-DQ2 (DRB1*03/DQA1*0501/DQB1*0201) haplotype was in 19% of the cases, followed by 14.3% HLA DR4-DQ8 (DRB1*04/DQA1*0301/DQB1*0302/3) (Table S1). In addition, another
9.52% were heterozygous for the HLA DQ2-DQ8 haplotype. Furthermore, 75% of HLA DR3-DQ2 cases of T1D, were carriers of a DQ8 allele. Regarding DR alleles, 95% of the T1D children presented
the DR4 variant (DRB1*04), while 66.6% were carriers of the DR3 allele (DRB1*03). In the control group, 12.5% were HLA DQ2. The rest of the control subjects were negative to DQ2 or DQ8;
however, 37.5% presented isolated alleles associated with DQ8. All the control children were negatives for the DR alleles. GUT MICROBIOTA A total of 245,134 rRNA16S reads were obtained from
the 29 samples, averaging 8,452 sequences per sample. The bacterial diversity was not significantly different between controls and the two T1D groups (Figures 1A, S1–2). However, beta
diversity analysis using principal coordinates indicate a separate clustering of the fecal communities in subjects with T1D at onset compared to controls (Figure 1B). The group of T1D cases
treated for 2 years did not show clustering and were spread along PC1 axis (Figures 1, 2, S3). Weighted UniFrac distances within groups were significantly smaller than between groups (_p_ =
0.000001) thus indicating group clustering (Figure 2). The major taxa explaining this clustering were not reflected at the phyla but at the genus level (Figure 3). Control children had a
fecal community dominated by _Prevotella_, while children with T1D at onset had higher representation of _Bacteroides_ (_p_ = 0.0037) showing substantially reduced proportions of
_Prevotella_ (_p_ = 0.0003), _Megamonas_ (_p_ = 0.0161) and _Acidaminococcus_ (_p_ = 0.0214), in comparison to controls. Children with T1D treated for 2 years showed an intermediate relative
abundance of these genera between the controls and T1D new cases (Figure 3, S4, S5). Dominant phyla were Bacteroidetes and Firmicutes, followed by Proteobacteria and Tenericutes, with no
differences between groups (_p_ > 0.05) and thus the ratio Bacteroidetes/Firmicutes was not significantly different between our groups. DISCUSSION T1D occurs typically in childhood or
adolescence and has been associated to host genetic factors with major histocompatibility complex (MHC) region harboring genes that contribute more than 50% of the risk, mediated by
HLADR3-DQ2 or DR4-DQ815. Our results show that these in T1D Mexican children had higher proportions of HLA DR4 (95%) and DR3 (66.6%) than Mexican-American T1D children (46% and 27%
respectively)16. In addition to genetic factors, environmental factors have also been epidemiologically associated to T1D. These include early life factors such as C-section17, lack of
breastfeeding5 and early infections and antibiotic exposure7, all factors that are known to alter microbial communities18,19. The higher frequency of antibiotic treatments prior to diagnosis
in T1D patients could influence the shift of the communities away from the controls; however, T1D at onset group had received fewer courses than the group of T1D treated for 2 years and
yet, had the most distinct community. There was higher dispersion in the structure of the gut microbiota in T1D cases, than in controls (Figure 2). This finding is consistent with those
found in previous studies related to autoimmunity and T1D10,12, suggesting instability of the microbiome associated with this condition. The fecal microbiota in Mexican healthy children of
our study had a similar composition to that reported in people from developing countries with high levels of _Prevotella_, in contrast with the _Bacteroides_-dominated microbiota of US
children and adults20,21. These differences are likely to be dietary, since _Bacteroides_ has been associated with high protein and fat diets, while carbohydrate rich diets, increase
_Prevotella_22. It is not clear if high dominance of _Bacteroides_ in the US is associated with higher risk of TD1, which is indeed higher in US than in Mexican children (11 to 17 vs 1.5 per
100,000, respectively)23. The incidence of T1D in the Sonora region -near the US-Mexican border- has doubled in the last 10 years24, suggesting that the factors underlying risks are
environmental and could be related to diet or lifestyle changes. _Bacteroides_-dominant gut communities were also observed in prediabetic Finish children who also showed decreased levels of
_Prevotella_ when compared to healthy controls25. Insulin treatment of T1D partially normalizes the microbiota profile towards _Prevotella_-dominant profile, indicating that physiological
changes related to T1D are driving the gut microbiota structure in T1D. It has been suggested that _Bacteroides_ activity in mucine-synthesis and degradation might be contributing to T1D
development by thinning of the mucus layer, leading to increased gut permeability and inflammation26. Lactate conversion to butyrate in the gut induces synthesis of mucins and consequently
favors a healthy epithelium. _Bacteroides_ would transform lactate into short chain fatty acids, such as propionate, acetate and succinate, which contribute to decrease mucin synthesis and
therefore the tight junctions could be affected25. Thus, the lactate model seems to be critical to maintain intestinal health and explain the way to autoimmunity in T1D. Bacterial antigens
and microorganisms toxins can be sensed by molecules related to epithelial cells' tight junctions like zonulin, claudin and occludin, altering their activity and consequently increasing
gut permeability and bacterial translocation27. In this context, some _Bacteroides sp._ with pathogen activity, such as _Bacteroides fragilis_, disrupts the tight junctions by proteolytic
degradation due to metalloprotease enterotoxins (e.g. fragilysin) increasing paracellular permeability with local inflammation, cell damage and loss of microvilli28. This process could lead
to loss of self-tolerance, causing aberrant immune responses, with an homeostatic imbalance of T-cells, gut inflammation and extra intestinal inflammatory infiltrate «insulitis» associated
with T1D29. The microbiota profile associated to T1D supports the idea of the existence of an “autoimmune” microbiome associated with diabetes10. However, this association does not
necessarily imply causality. Intervention studies where the gut microbiota structure could be normalized in children at high risk for T1D via fecal transplant, have not yet been performed,
but it could demonstrate an etiologic role of dysbiosis in T1D. METHODS STUDY POPULATION A cross-sectional case-control study was conducted in the Mexican Sonora state, between 2010 and
2012, recruiting children T1D patients at the Children's Hospital of the State of Sonora (HIES). The criteria for inclusion comprised being of 7 to 18 years of age, a T1D diagnose as
established according to the American Diabetes Association criteria1, a positive anti-GAD and/or anti-IA-2 auto-antibodies result, HbA1C levels < 8% and an antibiotic-free scheme for at
least 3 months prior to the sampling. Subjects presenting chronic and inflammatory gastrointestinal diseases were excluded from the study. In T1D patients, the insulin dose is currently
carefully calculated based on the carbohydrate content of the diet and the physical activity. In general, a high-fiber diet with low glycemic index is recommended30. All patients'
parents were informed about the nature of the study and were asked to provide written informed consent for their child's participation. All patients had nutritional counseling.
Information about birth delivery mode, breastfeeding, complementary feeding patterns in the first year of life, infections and antibiotic treatments prior to T1D diagnosis was collected in
an interview with the parent. Clinical records were revised and the protocol was approved by the Ethics Committee of the Centre for Food Research and Development and the HIES Learning and
Research Board. SAMPLE COLLECTION Peripheral blood (2 mL) was collected from all patients and stored in EDTA tubes at 4°C for haplotype analysis. Stool samples were obtained from every
subject and immediately placed on ice for transportation to the laboratory. The DNA extraction was performed within the first 3 h after collection. GDNA EXTRACTION Genomic DNA was extracted
from whole blood samples using the QIAamp DNA Blood Mini Kit (QIAGEN®), following manufacturer's instructions. For stool samples the QIAamp DNA Stool Mini Kit (QIAGEN®) was used. The
concentration and purity were evaluated using a Nanodrop ® spectrophotometer (Thermo Scientific, Wilmington, DE, USA). Extracted DNA was stored at −20°C. HLA TYPING The HLA DR3-DQ2 and
DR4-DQ8 typing was performed from blood gDNA through polymerase chain reaction (PCR) using sequence specific primers31 for DRB1*03, DRB1*04, DQA1*0501, DQA1*0301, DQB1*0201 and DQB1*0302/3
alleles (IDT-Integrated DNA Technologies, Tucson-AZ, USA). The amplicons obtained were resolved on 1.8% agarose gel, stained with GelRed™ (Biotium Inc., Hayward, CA, USA) and visualized
under ultraviolet light (Molecular Imager®Bio-Rad, Hercules, CA, USA). The allele HLA frequencies in both clinical groups were calculated using the direct counting method. MICROBIOTA
DETERMINATION The V4 region of the bacterial 16S rRNA gene was amplified from fecal gDNA with 357F/926R primers containing the A and B adaptors from 454 Life Sciences for pyrosequencing and
a unique 12 base pair error correcting Golay barcode, allowing multiplexing of samples in one single run. Replicate amplicons were pooled and purified using the UltraClean –htp 96 well PCR
clean up kit (MO BIO). The concentration of samples was measured and samples were combined in equimolar ratios into a single tube. Pyrosequencing was carried out on a 454 Life Sciences
Genome Sequencer FLX instrument (Roche) by the Human Genome Sequencing Center at Baylor College of Medicine, in Houston, Texas, USA, using their standard protocol. BIOINFORMATICS AND
COMMUNITY COMPARISONS The sequence and microbial communities diversity analysis was performed with the QIIME software package (Quantitative Insights Into Microbial Ecology)32. Sequences were
removed from the analysis if they were <200 nt, had a quality score < 25, contained ambiguous characters, contained an uncorrectable barcode, or did not contain the primer sequence.
Sequences were assigned to samples by examining their individual 12-nt barcode. Sequences were aligned using PyNAST, clustered into OTUs and taxonomy assigned using the Ribosomal Database
Project (RDP) as a reference base. The phylogenetic tree was built with FastTree. QIIME and UniFrac were used for analyses of bacterial communities and group comparisons33. Differences
between groups were assessed using ANOVA and Tukey-Kramer multiple-comparison test. REFERENCES * American Diabetes Association. Standards of medical care in diabetes 2013. Diabetes Care. 36,
S11–S66 (2013). * Fowler, M. J. Diabetes: magnitude and mechanisms. Clinical Diabetes. 25, 25–28 (2007). Article Google Scholar * Tollefsen, S. et al. Structural and functional studies of
trans-encoded HLA-DQ2.3 (DQA1*03:01/DQB1*02:01) protein molecule. J. Biol. Chem. 287, 13611–13619 (2012). Article CAS Google Scholar * Okada, H., Kuhn, C., Feillet, H. & Bach, J. F.
The ‘hygiene hypothesis’ for autoimmune and allergic diseases: an update. Clin. Exp. Immunol. 160, 1–9 (2010). Article CAS Google Scholar * Patelarou, E. et al. Current evidence on the
associations of breastfeeding, infant formula and cow's milk introduction with type 1 diabetes mellitus: a systematic review. Nutr. Rev. 70, 509–519 (2012). Article Google Scholar *
Spagnuolo, I. et al. The case for virus-induced type 1 diabetes. Curr. Opin. Endocrinol. Diabetes Obes. 20, 292–298 (2013). Article CAS Google Scholar * Abela, A. G. & Fava, S.
Association of incidence of type 1 diabetes with mortality from infectious disease and with antibiotic susceptibility at a country level. Acta Diabetol. 1007/s00592-013-0464-z (2013). *
Muirhead, C. R., Cheetham, T. D., Court, S., Begon, M. & McNally, R. J. Q. How do childhood diagnoses of type 1 diabetes cluster in time? PLoS ONE 8, e60489 (2013). Article CAS ADS
Google Scholar * Brugman, S. et al. Antibiotic treatment partially protects against type 1 diabetes in the Bio-Breeding diabetes-prone rat. Is the gut flora involved in the development of
type 1 diabetes? Diabetologia 49, 2105–2108 (2006). Article CAS Google Scholar * Giongo, A. et al. Toward defining the autoimmune microbiome for type 1 diabetes. ISME J. 5, 82–91 (2011).
Article CAS Google Scholar * de Goffau, M. C. et al. Fecal microbiota composition differs between children with β-cell autoimmunity and those without. Diabetes 62, 1238–1244 (2013).
Article CAS Google Scholar * Murri, M. et al. Gut microbiota in children with type 1 diabetes differs from that in healthy children: a case-control study. BMC Med. 11, 46 (2013). Article
ADS Google Scholar * Vaarala, O. Is the origin of type 1 diabetes in the gut? Immunol. Cell Biol. 90, 271–276 (2012). Article CAS Google Scholar * Oliver-Krasinski, J. M. &
Stoffers, D. A. On the origin of the β cell. Genes Dev. 22, 1998–2021 (2008). Article CAS Google Scholar * Kumar, N., Kaur, G., Tandon, N., Kanga, U. & Mehra, N. K. Genomic evaluation
of HLA-DR3+ haplotypes associated with type 1 diabetes. Ann. N. Y. Acad. Sci. 1283, 91–96 (2013). Article CAS ADS Google Scholar * Vadheim, C. M. et al. Different HLA haplotypes in
mexican americans with IDDM. Diabetes Care 12, 497–500 (1989). Article CAS Google Scholar * Neu, J. & Rushing, J. Cesarean versus vaginal delivery: long-term infant outcomes and the
hygiene hypothesis. Clin. Perinatol. 38, 321–331(2011). Article Google Scholar * Boerner, B. P. & Sarvetnick, N. E. Type 1 diabetes: role of intestinal microbiome in humans and mice.
Ann. N. Y. Acad. Sci. 1243, 103–118 (2011). Article CAS ADS Google Scholar * Atkinson, M. A. & Chervonsky, A. Does the gut microbiota have a role in type 1 diabetes? Early evidence
from humans and animal models of the disease. Diabetologia 55, 2868–2877 (2012). Article CAS Google Scholar * Yatsunenko, T. et al. Human gut microbiome viewed across age and geography.
Nature 486, 222–227 (2012). Article CAS ADS Google Scholar * Lin, A. et al. Distinct distal gut microbiome diversity and composition in healthy children from Bangladesh and the United
States. PLoS ONE 8, e53838 (2013). Article CAS ADS Google Scholar * Wu, G. D. et al. Linking long-term dietary patterns with gut microbial enterotypes. Science 334, 105–108(2011).
Article CAS ADS Google Scholar * Karvonen, M. et al. Incidence of childhood type 1 diabetes worldwide. Diabetes Mondiale (DiaMond) Project Group. Diabetes Care 23, 1516–1526 (2000).
Article CAS Google Scholar * Enríquez-Leal, M. C. et al. Incidence, clinical characteristics and nutritional status in diabetic Mexican children and adolescents. Interciencia 35, 455–460
(2010). Google Scholar * Brown, C. T. et al. Gut microbiome metagenomics analysis suggests a functional model for the development of autoimmunity for type 1 diabetes. PLoS ONE 6, e25792
(2011). Article CAS ADS Google Scholar * Tlaskalova-Hogenova, H. et al. The role of gut microbiota (commensal bacteria) and the mucosal barrier in the pathogenesis of inflammatory and
autoimmune diseases and cancer: contribution of germ-free and gnotobiotic animal models of human diseases. Cell. Mol. Immunol. 8, 110–120 (2011). Article CAS Google Scholar * Vaarala, O.
Human intestinal microbiota and type 1 diabetes. Curr. Diab. Rep. 13, 1–7 (2013). Article Google Scholar * Berkes, J. Intestinal epithelial responses to enteric pathogens: effects on the
tight junction barrier, ion transport and inflammation. Gut. 52, 439–451 (2003). Article CAS Google Scholar * Zipris, D. The interplay between the gut microbiota and the immune system in
the mechanism of type 1 diabetes. Curr. Opin. Endocrinol. Diabetes Obes. 20, 265–270 (2013). Article CAS Google Scholar * American Diabetes Association. Nutrition recommendations and
interventions for diabetes–2006: a position statement of the American Diabetes Association. Diabetes Care 29, 2140–2157(2006). * Olerup, O., Aldener, A. & Fogdell, A. HLA-DQB1 and -DQA1
typing by PCR amplification with sequence-specific primers (PCR-SSP) in 2 hours. Tissue Antigens. 41, 119–134 (1993). Article CAS Google Scholar * Caporaso, J. G. et al. QIIME allows
analysis of high-throughput community sequencing data. Nature Methods 7, 335–336 (2010). Article CAS Google Scholar * Lozupone, C. & Knight, R. UniFrac: a new phylogenetic method for
comparing microbial communities. Appl. Environ. Microbiol. 71, 8228–8235 (2005). Article CAS Google Scholar Download references ACKNOWLEDGEMENTS The work had partial support from the
Mexican Council for Science and Technology (CONACYT), grant S0008-2009-01-115212. Authors are grateful to Dr. V. Mata Haro, from CIAD for her advice on the study design and MD. G. Garcia
Galvan, Pediatric Endocrinologist from HIES, for her support during subjects' recruitment. Thanks also to S.M. Rodriguez Rivera from University of Puerto Rico Department of Biology, for
her assistance in sequence analysis and to E. Hyde from Baylor College of Medicine Alkek Center for Metagenomics and Microbiome Research, for her comments to the manuscript. AUTHOR
INFORMATION AUTHORS AND AFFILIATIONS * Department of Nutrition and Metabolism, Centro de Investigación en Alimentación y Desarrollo, A.C., Hermosillo, Sonora, México María Esther Mejía-León
& Ana María Calderón de la Barca * Alkek Center for Metagenomics and Microbiome Research, Department of Molecular Virology and Microbiology, Baylor College of Medicine, Houston, Texas,
USA Joseph F. Petrosino & Nadim Jose Ajami * Laboratory of Microbial Ecology, Department of Biology, University of Puerto Rico, San Juan, Puerto Rico María Gloria Domínguez-Bello *
Department of Medicine, New York University School of Medicine, New York, NY, USA María Gloria Domínguez-Bello Authors * María Esther Mejía-León View author publications You can also search
for this author inPubMed Google Scholar * Joseph F. Petrosino View author publications You can also search for this author inPubMed Google Scholar * Nadim Jose Ajami View author publications
You can also search for this author inPubMed Google Scholar * María Gloria Domínguez-Bello View author publications You can also search for this author inPubMed Google Scholar * Ana María
Calderón de la Barca View author publications You can also search for this author inPubMed Google Scholar CONTRIBUTIONS M.E.M. and A.M.C. designed and conducted the study, collected samples,
analyzed haplotypes, extracted DNA, analyzed data and wrote the main manuscript text, J.F.P. and N.J.A. run the microbiota pyrosequence and suggested data analyses methods and M.D.B.
supervised the analysis data and wrote the main manuscript text. All authors reviewed the manuscript. ETHICS DECLARATIONS COMPETING INTERESTS The authors declare no competing financial
interests. ELECTRONIC SUPPLEMENTARY MATERIAL SUPPLEMENTARY INFORMATION Supplementary Information RIGHTS AND PERMISSIONS This work is licensed under a Creative Commons Attribution 3.0
Unported License. To view a copy of this license, visit http://creativecommons.org/licenses/by/3.0/ Reprints and permissions ABOUT THIS ARTICLE CITE THIS ARTICLE Mejía-León, M., Petrosino,
J., Ajami, N. _et al._ Fecal microbiota imbalance in Mexican children with type 1 diabetes. _Sci Rep_ 4, 3814 (2014). https://doi.org/10.1038/srep03814 Download citation * Received: 23
October 2013 * Accepted: 02 January 2014 * Published: 22 January 2014 * DOI: https://doi.org/10.1038/srep03814 SHARE THIS ARTICLE Anyone you share the following link with will be able to
read this content: Get shareable link Sorry, a shareable link is not currently available for this article. Copy to clipboard Provided by the Springer Nature SharedIt content-sharing
initiative