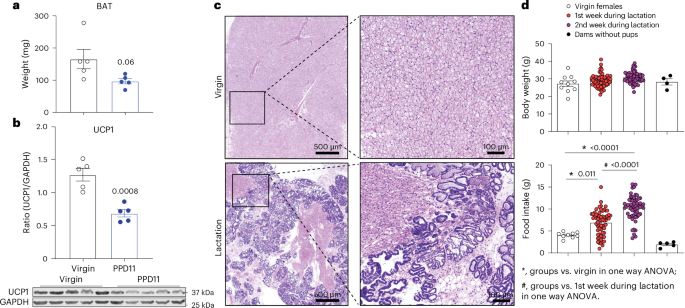
- Select a language for the TTS:
- UK English Female
- UK English Male
- US English Female
- US English Male
- Australian Female
- Australian Male
- Language selected: (auto detect) - EN
Play all audios:
ABSTRACT 17β-oestradiol (E2) inhibits overeating and promotes brown adipose tissue (BAT) thermogenesis, whereas prolactin (PRL) does the opposite. During lactation, the simultaneous decline
in E2 and surge in PRL contribute to maternal metabolic adaptations, including hyperphagia and suppressed BAT thermogenesis. However, the underlying neuroendocrine mechanisms remain unclear.
Here, we find that oestrogen receptor alpha (ERα)-expressing neurons in the medial basal hypothalamus (MBH), specifically the arcuate nucleus of the hypothalamus and the ventrolateral
subdivision of the ventromedial hypothalamus (vlVMH), are suppressed during lactation. Deletion of ERα from MBH neurons in virgin female mice induces metabolic phenotypes characteristic of
lactation, including hyperprolactinemia, hyperphagia and suppressed BAT thermogenesis. By contrast, activation of ERαvlVMH neurons in lactating mice attenuates these phenotypes. Overall, our
study reveals an inhibitory effect of E2–ERαvlVMH signalling on PRL production, which is suppressed during lactation to sustain hyperprolactinemia and metabolic adaptations. Access through
your institution Buy or subscribe This is a preview of subscription content, access via your institution ACCESS OPTIONS Access through your institution Access Nature and 54 other Nature
Portfolio journals Get Nature+, our best-value online-access subscription $29.99 / 30 days cancel any time Learn more Subscribe to this journal Receive 12 digital issues and online access to
articles $119.00 per year only $9.92 per issue Learn more Buy this article * Purchase on SpringerLink * Instant access to full article PDF Buy now Prices may be subject to local taxes which
are calculated during checkout ADDITIONAL ACCESS OPTIONS: * Log in * Learn about institutional subscriptions * Read our FAQs * Contact customer support SIMILAR CONTENT BEING VIEWED BY
OTHERS OXYTOCIN RECEPTOR INDUCES MAMMARY TUMORIGENESIS THROUGH PROLACTIN/P-STAT5 PATHWAY Article Open access 07 June 2021 OESTROGEN ENGAGES BRAIN MC4R SIGNALLING TO DRIVE PHYSICAL ACTIVITY
IN FEMALE MICE Article 13 October 2021 BETA CELL ADAPTATION TO PREGNANCY REQUIRES PROLACTIN ACTION ON BOTH BETA AND NON-BETA CELLS Article Open access 14 May 2021 DATA AVAILABILITY All data
supporting this publication are available in the Source Data published alongside the paper. A reporting summary for this article is available as a Supplementary Information file. Patch–seq
data are accessible from the NCBI GEO database (GSE284575). Source data are provided with this paper. REFERENCES * Yohkaichiya, T., O’Connor, A. & de Kretser, D. M. Circulating
immunoreactive inhibin, gonadotropin, and prolactin levels during pregnancy, lactation, and postweaning estrous cycle in the rat. _Biol. Reprod._ 44, 6–12 (1991). Article CAS PubMed
Google Scholar * Neville, M. C., McFadden, T. B. & Forsyth, I. Hormonal regulation of mammary differentiation and milk secretion. _J. Mammary Gland Biol. Neoplasia_ 7, 49–66 (2002).
Article PubMed Google Scholar * Kerlan, V., Nahoul, K., Le Martelot, M. T. & Bercovici, J. P. Longitudinal study of maternal plasma bioavailable testosterone and androstanediol
glucuronide levels during pregnancy. _Clin. Endocrinol. (Oxf.)_ 40, 263–267 (1994). Article CAS PubMed Google Scholar * Taya, K. & Greenwald, G. S. Peripheral blood and ovarian
levels of sex steroids in the lactating rat. _Endocrinol. Jpn._ 29, 453–459 (1982). Article CAS PubMed Google Scholar * Woodside, B., Budin, R., Wellman, M. K. & Abizaid, A. Many
mouths to feed: the control of food intake during lactation. _Front. Neuroendocrinol._ 33, 301–314 (2012). Article CAS PubMed Google Scholar * Chan, E. & Swaminathan, R. Role of
prolactin in lactation-induced changes in brown adipose tissue. _Am. J. Physiol._ 258, R51–R56 (1990). CAS PubMed Google Scholar * Krol, E. & Speakman, J. R. Switching off the
furnace: brown adipose tissue and lactation. _Mol. Asp. Med._ 68, 18–41 (2019). Article CAS Google Scholar * Ropero, A. B., Alonso-Magdalena, P., Quesada, I. & Nadal, A. The role of
estrogen receptors in the control of energy and glucose homeostasis. _Steroids_ 73, 874–879 (2008). Article CAS PubMed Google Scholar * Mauvais-Jarvis, F., Clegg, D. J. & Hevener, A.
L. The role of estrogens in control of energy balance and glucose homeostasis. _Endocr. Rev._ 34, 309–338 (2013). Article CAS PubMed PubMed Central Google Scholar * Lopez, M. &
Tena-Sempere, M. Estrogens and the control of energy homeostasis: a brain perspective. _Trends Endocrinol. Metab._ 26, 411–421 (2015). Article CAS PubMed Google Scholar * Bernard, V.,
Young, J. & Binart, N. Prolactin—a pleiotropic factor in health and disease. _Nat. Rev. Endocrinol._ 15, 356–365 (2019). Article CAS PubMed Google Scholar * Crowley, W. R.
Neuroendocrine regulation of lactation and milk production. _Compr. Physiol._ 5, 255–291 (2015). Article PubMed Google Scholar * Brown, R. S. E. et al. Prolactin action in the medial
preoptic area is necessary for postpartum maternal nursing behavior. _Proc. Natl Acad. Sci. USA_ 114, 10779–10784 (2017). Article CAS PubMed PubMed Central Google Scholar *
Lopez-Vicchi, F. et al. Severe hyperprolactinemia promotes brown adipose tissue whitening and aggravates high fat diet induced metabolic imbalance. _Front. Endocrinol._ 13, 883092 (2022).
Article Google Scholar * Sauve, D. & Woodside, B. The effect of central administration of prolactin on food intake in virgin female rats is dose-dependent, occurs in the absence of
ovarian hormones and the latency to onset varies with feeding regimen. _Brain Res._ 729, 75–81 (1996). Article CAS PubMed Google Scholar * Moore, B. J., Gerardo-Gettens, T., Horwitz, B.
A. & Stern, J. S. Hyperprolactinemia stimulates food intake in the female rat. _Brain Res. Bull._ 17, 563–569 (1986). Article CAS PubMed Google Scholar * Xu, Y. et al. Distinct
hypothalamic neurons mediate estrogenic effects on energy homeostasis and reproduction. _Cell Metab._ 14, 453–465 (2011). Article CAS PubMed PubMed Central Google Scholar * Krause, W.
C. et al. Oestrogen engages brain MC4R signalling to drive physical activity in female mice. _Nature_ 599, 131–135 (2021). Article CAS PubMed PubMed Central Google Scholar * Martinez de
Morentin, P. B. et al. Estradiol regulates brown adipose tissue thermogenesis via hypothalamic AMPK. _Cell Metab._ 20, 41–53 (2014). Article CAS PubMed PubMed Central Google Scholar *
Musatov, S. et al. Silencing of estrogen receptor ⍺ in the ventromedial nucleus of hypothalamus leads to metabolic syndrome. _Proc. Natl Acad. Sci. USA_ 104, 2501–2506 (2007). Article CAS
PubMed PubMed Central Google Scholar * Ye, H. et al. An estrogen-sensitive hypothalamus–midbrain neural circuit controls thermogenesis and physical activity. _Sci. Adv._ 8, eabk0185
(2022). Article CAS PubMed PubMed Central Google Scholar * Grattan, D. R. 60 Years of neuroendocrinology: the hypothalamo-prolactin axis. _J. Endocrinol._ 226, T101–T122 (2015). Article
CAS PubMed PubMed Central Google Scholar * Grattan, D. R. & Kokay, I. C. Prolactin: a pleiotropic neuroendocrine hormone. _J. Neuroendocrinol._ 20, 752–763 (2008). Article CAS
PubMed Google Scholar * Asa, S. L., Kelly, M. A., Grandy, D. K. & Low, M. J. Pituitary lactotroph adenomas develop after prolonged lactotroph hyperplasia in dopamine D2
receptor-deficient mice. _Endocrinology_ 140, 5348–5355 (1999). Article CAS PubMed Google Scholar * Schuff, K. G. et al. Lack of prolactin receptor signaling in mice results in
lactotroph proliferation and prolactinomas by dopamine-dependent and -independent mechanisms. _J. Clin. Investig._ 110, 973–981 (2002). Article CAS PubMed PubMed Central Google Scholar
* Bernard, V. et al. Natural and molecular history of prolactinoma: insights from _a Prlr_−/− mouse model. _Oncotarget_ 9, 6144–6155 (2018). Article PubMed Google Scholar * Yip, S. H. et
al. Elevated prolactin during pregnancy drives a phenotypic switch in mouse hypothalamic dopaminergic neurons. _Cell Rep._ 26, 1787–1799.e5 (2019). Article CAS PubMed Google Scholar *
Lyons, D. J., Hellysaz, A. & Broberger, C. Prolactin regulates tuberoinfundibular dopamine neuron discharge pattern: novel feedback control mechanisms in the lactotrophic axis. _J.
Neurosci._ 32, 8074–8083 (2012). Article CAS PubMed PubMed Central Google Scholar * Grattan, D. R. et al. Feedback regulation of PRL secretion is mediated by the transcription factor,
signal transducer, and activator of transcription 5b. _Endocrinology_ 142, 3935–3940 (2001). Article CAS PubMed Google Scholar * Kelly, M. A. et al. Pituitary lactotroph hyperplasia and
chronic hyperprolactinemia in dopamine D2 receptor-deficient mice. _Neuron_ 19, 103–113 (1997). Article CAS PubMed Google Scholar * Brown, R. S. et al. Conditional deletion of the
prolactin receptor reveals functional subpopulations of dopamine neurons in the arcuate nucleus of the hypothalamus. _J. Neurosci._ 36, 9173–9185 (2016). Article CAS PubMed PubMed Central
Google Scholar * Buonfiglio, D. C. et al. Neuronal STAT5 signaling is required for maintaining lactation but not for postpartum maternal behaviors in mice. _Hormones Behav._ 71, 60–68
(2015). Article CAS Google Scholar * Mena, F., Enjalbert, A., Carbonell, L., Priam, M. & Kordan, C. Effect of suckling on plasma prolactin and hypothalamic monoamine levels in the
rat. _Endocrinology_ 99, 445–451 (1976). Article CAS PubMed Google Scholar * Silva, K. S. C. et al. Reduced dopaminergic tone during lactation is permissive to the hypothalamic stimulus
for suckling-induced prolactin release. _J. Neuroendocrinol._ 32, e12880 (2020). Article CAS PubMed Google Scholar * Kennett, J. E. & McKee, D. T. Oxytocin: an emerging regulator of
prolactin secretion in the female rat. _J. Neuroendocrinol._ 24, 403–412 (2012). Article CAS PubMed PubMed Central Google Scholar * Kam, K. et al. Effects of immobilization stress on
estrogen-induced surges of luteinizing hormone and prolactin in ovariectomized rats. _Endocrine_ 12, 279–287 (2000). Article CAS PubMed Google Scholar * Joubert-Bression, D., Brandi, A.
M., Birman, P. & Peillon, F. Effect of oestradiol on dopamine receptors and protein kinase C activity in the rat pituitary: binding of oestradiol to pituitary membranes. _Ciba Found.
Symp._ 153, 156–168. Discussion 168–171 (1990). * Pasqualini, C., Bojda, F. & Kerdelhue, B. Direct effect of estradiol on the number of dopamine receptors in the anterior pituitary of
ovariectomized rats. _Endocrinology_ 119, 2484–2489 (1986). Article CAS PubMed Google Scholar * Maus, M. et al. Differential modulation of D1 and D2 dopamine-sensitive adenylate cyclases
by 17β-estradiol in cultured striatal neurons and anterior pituitary cells. _J. Neurochem._ 52, 410–418 (1989). Article CAS PubMed Google Scholar * Pelletier, G., Li, S., Phaneuf, D.,
Martel, C. & Labrie, F. Morphological studies of prolactin-secreting cells in estrogen receptor ⍺ and estrogen receptor β knockout mice. _Neuroendocrinology_ 77, 324–333 (2003). Article
CAS PubMed Google Scholar * Scully, K. M. et al. Role of estrogen receptor-⍺ in the anterior pituitary gland. _Mol. Endocrinol._ 11, 674–681 (1997). CAS PubMed Google Scholar *
Camilletti, M. A. et al. Role of GPER in the anterior pituitary gland focusing on lactotroph function. _J. Endocrinol._ 240, 99–110 (2019). Article CAS PubMed Google Scholar * Phillipps,
H. R., Khant Aung, Z. & Grattan, D. R. Elevated prolactin secretion during proestrus in mice: absence of a defined surge. _J. Neuroendocrinol._ 34, e13129 (2022). Article CAS PubMed
PubMed Central Google Scholar * Kawashima, K. et al. The estrogen-occupied estrogen receptor functions as a negative regulator to inhibit cell proliferation induced by insulin/IGF-1: a
cell context-specific antimitogenic action of estradiol on rat lactotrophs in culture. _Endocrinology_ 143, 2750–2758 (2002). Article CAS PubMed Google Scholar * Zaldivar, V. et al.
Estradiol increases the Bax/Bcl-2 ratio and induces apoptosis in the anterior pituitary gland. _Neuroendocrinology_ 90, 292–300 (2009). Article CAS PubMed Google Scholar * Zarate, S. et
al. Estrogens exert a rapid apoptotic action in anterior pituitary cells. _Am. J. Physiol. Endocrinol. Metab._ 296, E664–E671 (2009). Article CAS PubMed Google Scholar * Gorospe, W. C.
& Freeman, M. E. An ovarian role in prolonging and terminating the two surges of prolactin in pseudopregnant rats. _Endocrinology_ 108, 1293–1298 (1981). Article CAS PubMed Google
Scholar * Furudate, S. Effects of estradiol on the prolactin surges and the feedback mechanisms of gonadotropins in pseudopregnant rats. _Jikken Dobutsu_ 40, 203–208 (1991). CAS PubMed
Google Scholar * Furigo, I. C. et al. Prolactin-sensitive neurons express estrogen receptor-⍺ and depend on sex hormones for normal responsiveness to prolactin. _Brain Res._ 1566, 47–59
(2014). Article CAS PubMed Google Scholar * Steyn, F. J., Anderson, G. M. & Grattan, D. R. Expression of ovarian steroid hormone receptors in tuberoinfundibular dopaminergic neurones
during pregnancy and lactation. _J. Neuroendocrinol._ 19, 788–793 (2007). Article CAS PubMed Google Scholar * Olah, S. et al. Prolactin-induced and neuronal activation in the brain of
mother mice. _Brain Struct. Funct._ 223, 3229–3250 (2018). Article CAS PubMed Google Scholar * Blum, M., McEwen, B. S. & Roberts, J. L. Transcriptional analysis of tyrosine
hydroxylase gene expression in the tuberoinfundibular dopaminergic neurons of the rat arcuate nucleus after estrogen treatment. _J. Biol. Chem._ 262, 817–821 (1987). Article CAS PubMed
Google Scholar * Pasqualini, C., Guibert, B. & Leviel, V. Short-term inhibitory effect of estradiol on tyrosine hydroxylase activity in tuberoinfundibular dopaminergic neurons in vitro.
_J. Neurochem._ 60, 1707–1713 (1993). Article CAS PubMed Google Scholar * Demarest, K. T., Riegle, G. D. & Moore, K. E. Long-term treatment with estradiol induces reversible
alterations in tuberoinfundibular dopaminergic neurons: a decreased responsiveness to prolactin. _Neuroendocrinology_ 39, 193–200 (1984). Article CAS PubMed Google Scholar * Arita, J.
& Kimura, F. Direct inhibitory effect of long term estradiol treatment on dopamine synthesis in tuberoinfundibular dopaminergic neurons: in vitro studies using hypothalamic slices.
_Endocrinology_ 121, 692–698 (1987). Article CAS PubMed Google Scholar * DeMaria, J. E., Livingstone, J. D. & Freeman, M. E. Ovarian steroids influence the activity of neuroendocrine
dopaminergic neurons. _Brain Res._ 879, 139–147 (2000). Article CAS PubMed Google Scholar * Morel, G. R. et al. Estrogen inhibits tuberoinfundibular dopaminergic neurons but does not
cause irreversible damage. _Brain Res. Bull._ 80, 347–352 (2009). Article CAS PubMed PubMed Central Google Scholar * Lee, H. et al. Scalable control of mounting and attack by Esr1+
neurons in the ventromedial hypothalamus. _Nature_ 509, 627–632 (2014). Article CAS PubMed PubMed Central Google Scholar * Hashikawa, K. et al. Esr1+ cells in the ventromedial
hypothalamus control female aggression. _Nat. Neurosci._ 20, 1580–1590 (2017). Article CAS PubMed PubMed Central Google Scholar * Liu, M., Kim, D. W., Zeng, H. & Anderson, D. J.
Make war not love: the neural substrate underlying a state-dependent switch in female social behavior. _Neuron_ 110, 841–856.e6 (2022). Article CAS PubMed PubMed Central Google Scholar
* Georgescu, T., Khant Aung, Z., Grattan, D. R. & Brown, R. S. E. Prolactin-mediated restraint of maternal aggression in lactation. _Proc. Natl Acad. Sci. USA_ 119, e2116972119 (2022).
Article CAS PubMed PubMed Central Google Scholar * Musatov, S., Chen, W., Pfaff, D. W., Kaplitt, M. G. & Ogawa, S. RNAi-mediated silencing of estrogen receptor ⍺ in the ventromedial
nucleus of hypothalamus abolishes female sexual behaviors. _Proc. Natl Acad. Sci. USA_ 103, 10456–10460 (2006). Article CAS PubMed PubMed Central Google Scholar * Spiteri, T. et al.
The role of the estrogen receptor ⍺ in the medial amygdala and ventromedial nucleus of the hypothalamus in social recognition, anxiety and aggression. _Behav. Brain Res_ 210, 211–220 (2010).
Article CAS PubMed Google Scholar * Villarroya, F., Felipe, A. & Mampel, T. Reduced noradrenaline turnover in brown adipose tissue of lactating rats. _Comp. Biochem. Physiol. A
Comp. Physiol._ 86, 481–483 (1987). Article CAS PubMed Google Scholar * Saito, K. et al. Visualizing estrogen receptor-⍺-expressing neurons using a new ER⍺-ZsGreen reporter mouse line.
_Metabolism_ 65, 522–532 (2016). Article CAS PubMed Google Scholar * & Yu, K. et al. 17β-estradiol promotes acute refeeding in hungry mice via membrane-initiated ERα signaling. _Mol.
Metab._ 42, 101053 (2020). Article CAS PubMed PubMed Central Google Scholar * van Veen, J. E. et al. Hypothalamic estrogen receptor alpha establishes a sexually dimorphic regulatory
node of energy expenditure. _Nat. Metab._ 2, 351–363 (2020). Article PubMed PubMed Central Google Scholar * Liu, X., Brown, R. S., Herbison, A. E. & Grattan, D. R. Lactational
anovulation in mice results from a selective loss of kisspeptin input to GnRH neurons. _Endocrinology_ 155, 193–203 (2014). Article CAS PubMed Google Scholar * VanHouten, J. N. &
Wysolmerski, J. J. Low estrogen and high parathyroid hormone-related peptide levels contribute to accelerated bone resorption and bone loss in lactating mice. _Endocrinology_ 144, 5521–5529
(2003). Article CAS PubMed Google Scholar * Mina, A. I. et al. CalR: a web-based analysis tool for indirect calorimetry experiments. _Cell Metab._ 28, 656–666.e1 (2018). Article CAS
PubMed PubMed Central Google Scholar * Broughton, D. E. & Moley, K. H. Obesity and female infertility: potential mediators of obesity’s impact. _Fertil. Steril._ 107, 840–847 (2017).
Article PubMed Google Scholar * Herber, C. B. et al. Estrogen signaling in arcuate Kiss1 neurons suppresses a sex-dependent female circuit promoting dense strong bones. _Nat. Commun._ 10,
163 (2019). Article PubMed PubMed Central Google Scholar * Kleinberg, D. L., Todd, J. & Babitsky, G. Inhibition by estradiol of the lactogenic effect of prolactin in primate mammary
tissue: reversal by antiestrogens LY 156758 and tamoxifen. _Proc. Natl Acad. Sci. USA_ 80, 4144–4148 (1983). Article CAS PubMed PubMed Central Google Scholar * Li, X. et al. Virus
injection to the pituitary via transsphenoidal approach and the innervation of anterior and posterior pituitary of rat. _Front Endocrinol. (Lausanne)_ 11, 546350 (2020). Article PubMed
Google Scholar * Lo, L. et al. Connectional architecture of a mouse hypothalamic circuit node controlling social behavior. _Proc. Natl Acad. Sci. USA_ 116, 7503–7512 (2019). Article CAS
PubMed PubMed Central Google Scholar * Kramer, D. J. et al. Generation of a DAT-P2A-Flpo mouse line for intersectional genetic targeting of dopamine neuron subpopulations. _Cell Rep._ 35,
109123 (2021). Article CAS PubMed PubMed Central Google Scholar * McIlvride, S. et al. A progesterone–brown fat axis is involved in regulating fetal growth. _Sci. Rep._ 7, 10671
(2017). Article PubMed PubMed Central Google Scholar * van den Berg, M. P., Verhoef, J. C., Romeijn, S. G. & Merkus, F. W. Uptake of estradiol or progesterone into the CSF following
intranasal and intravenous delivery in rats. _Eur. J. Pharm. Biopharm._ 58, 131–135 (2004). Article PubMed Google Scholar * Fujisawa, S. et al. Orexin A modulates prolactin production by
regulating BMP-4 activity in rat pituitary lactotorope cells. _Peptides_ 113, 35–40 (2019). Article CAS PubMed Google Scholar * Woodside, B. Prolactin and the hyperphagia of lactation.
_Physiol. Behav._ 91, 375–382 (2007). Article CAS PubMed Google Scholar * Macotela, Y., Triebel, J. & Clapp, C. Time for a new perspective on prolactin in metabolism. _Trends
Endocrinol. Metab._ 31, 276–286 (2020). Article CAS PubMed Google Scholar * Auffret, J. et al. Beige differentiation of adipose depots in mice lacking prolactin receptor protects against
high-fat-diet-induced obesity. _FASEB J._ 26, 3728–3737 (2012). Article CAS PubMed Google Scholar * Sharma, N., Baliarsingh, S. & Kaushik, G. G. Biochemical association of
hyperprolactinemia with hypothyroidism in infertile women. _Clin. Lab_ 58, 805–810 (2012). CAS PubMed Google Scholar * Tay, C. C., Glasier, A. F., Illingworth, P. J. & Baird, D. T.
Abnormal twenty-four hour pattern of pulsatile luteinizing hormone secretion and the response to naloxone in women with hyperprolactinaemic amenorrhoea. _Clin. Endocrinol. (Oxf.)_ 39,
599–606 (1993). Article CAS PubMed Google Scholar * Camilletti, M. A., Abeledo-Machado, A., Faraoni, E. Y., Thomas, P. & Diaz-Torga, G. New insights into progesterone actions on
prolactin secretion and prolactinoma development. _Steroids_ 152, 108496 (2019). Article CAS PubMed Google Scholar * Auriemma, R. S. et al. The interplay between prolactin and
reproductive system: focus on uterine pathophysiology. _Front Endocrinol. (Lausanne)_ 11, 594370 (2020). Article PubMed Google Scholar * Inoue, S. et al. Periodic remodeling in a neural
circuit governs timing of female sexual behavior. _Cell_ 179, 1393–1408.e16 (2019). Article CAS PubMed PubMed Central Google Scholar * Feng, Y., Manka, D., Wagner, K. U. & Khan, S.
A. Estrogen receptor-⍺ expression in the mammary epithelium is required for ductal and alveolar morphogenesis in mice. _Proc. Natl Acad. Sci. USA_ 104, 14718–14723 (2007). Article CAS
PubMed PubMed Central Google Scholar * Hadsell, D. L. et al. In-silico QTL mapping of postpubertal mammary ductal development in the mouse uncovers potential human breast cancer risk
loci. _Mamm. Genome_ 26, 57–79 (2015). Article CAS PubMed PubMed Central Google Scholar * Orso, R. et al. How early life stress impact maternal care: a systematic review of rodent
studies. _Front. Behav. Neurosci._ 13, 197 (2019). Article PubMed PubMed Central Google Scholar * Wada, T. et al. Impact of central and peripheral estrogen treatment on anxiety and
depression phenotypes in a mouse model of postmenopausal obesity. _PLoS ONE_ 13, e0209859 (2018). Article CAS PubMed PubMed Central Google Scholar * Singh, P., Song, C. Y., Dutta, S.
R., Gonzalez, F. J. & Malik, K. U. Central CYP1B1 (cytochrome P450 1B1)-estradiol metabolite 2-methoxyestradiol protects from hypertension and neuroinflammation in female mice.
_Hypertension_ 75, 1054–1062 (2020). Article CAS PubMed Google Scholar * He, Y. et al. Estrogen receptor-⍺ expressing neurons in the ventrolateral VMH regulate glucose balance. _Nat.
Commun._ 11, 2165 (2020). Article CAS PubMed PubMed Central Google Scholar * Wang, C. et al. TAp63 contributes to sexual dimorphism in POMC neuron functions and energy homeostasis.
_Nat. Commun._ 9, 1544 (2018). Article CAS PubMed PubMed Central Google Scholar Download references ACKNOWLEDGEMENTS Investigators involved in this work were supported by grants from
the NIH (R01DK129548 and R56DK133776 to Yanlin He, R00DK107008, P30 DK020595 and R01DK123098 to P.X., R01 DK136627 and K01DK119471 to C.W., F32DK134121 to K.M.C.), USDA/CRIS
(3092-51000-062-04(B)S to C.W. and 3092-51000-056 to D.L.H), TCH-2023 Pediatric Pilot award to C.W., American Heart Association awards (20POST35120600 to Yang He and 20POST000204188 to L.T.)
and the DOD (Innovative Grant W81XWH-20-1-0075 to P.X.). E2 and progesterone were measured using liquid chromatography hyphenated with mass spectrometry at the Metabolomics Core at Baylor
College of Medicine. Patch–seq samples were processed and sequenced in the genetic core at Pennington Biomedical Research Center, which is funded by the COBRE grant P20 GM135002 from NIGMS.
H129-ΔTK-TT-dTomato virus was obtained from the Center for Neuroanatomy with Neurotropic Viruses, supported by NIH Virus Center grant no. P40 OD010996. AUTHOR INFORMATION Author notes *
These authors contributed equally: Meng Yu, Bing Feng, Jonathan C. Bean. AUTHORS AND AFFILIATIONS * Children’s Nutrition Research Center, Department of Pediatrics, Baylor College of
Medicine, Houston, TX, USA Meng Yu, Jonathan C. Bean, Yongjie Yang, Hailan Liu, Yongxiang Li, Benjamin P. Eappen, Hesong Liu, Longlong Tu, Kristine M. Conde, Mengjie Wang, Xi Chen, Na Yin,
Darah Ave Threat, Nathan Xu, Junying Han, Yi Zhu, Darryl L. Hadsell, Yang He & Chunmei Wang * Pennington Biomedical Research Center, Brain Glycemic and Metabolism Control Department,
Louisiana State University, Baton Rouge, LA, USA Bing Feng, Qianru Zhao, Peiyu Gao & Yanlin He * Division of Endocrinology, Diabetes, and Metabolism, Department of Medicine, The
University of Illinois at Chicago, Chicago, IL, USA Pingwen Xu Authors * Meng Yu View author publications You can also search for this author inPubMed Google Scholar * Bing Feng View author
publications You can also search for this author inPubMed Google Scholar * Jonathan C. Bean View author publications You can also search for this author inPubMed Google Scholar * Qianru Zhao
View author publications You can also search for this author inPubMed Google Scholar * Yongjie Yang View author publications You can also search for this author inPubMed Google Scholar *
Hailan Liu View author publications You can also search for this author inPubMed Google Scholar * Yongxiang Li View author publications You can also search for this author inPubMed Google
Scholar * Benjamin P. Eappen View author publications You can also search for this author inPubMed Google Scholar * Hesong Liu View author publications You can also search for this author
inPubMed Google Scholar * Longlong Tu View author publications You can also search for this author inPubMed Google Scholar * Kristine M. Conde View author publications You can also search
for this author inPubMed Google Scholar * Mengjie Wang View author publications You can also search for this author inPubMed Google Scholar * Xi Chen View author publications You can also
search for this author inPubMed Google Scholar * Na Yin View author publications You can also search for this author inPubMed Google Scholar * Darah Ave Threat View author publications You
can also search for this author inPubMed Google Scholar * Nathan Xu View author publications You can also search for this author inPubMed Google Scholar * Junying Han View author
publications You can also search for this author inPubMed Google Scholar * Peiyu Gao View author publications You can also search for this author inPubMed Google Scholar * Yi Zhu View author
publications You can also search for this author inPubMed Google Scholar * Darryl L. Hadsell View author publications You can also search for this author inPubMed Google Scholar * Yang He
View author publications You can also search for this author inPubMed Google Scholar * Pingwen Xu View author publications You can also search for this author inPubMed Google Scholar *
Yanlin He View author publications You can also search for this author inPubMed Google Scholar * Chunmei Wang View author publications You can also search for this author inPubMed Google
Scholar CONTRIBUTIONS M.Y. performed surgeries and monitored metabolic phenotypes. B.F., Q.Z. and Yang He performed electrophysiological studies and patch–seq sample collection. J.B.
performed the data analysis of patch–seq and secondary analysis of scRNA-seq results. Y.Y., Hailan Liu, Y.L. and B.E. assisted with data collection. Hesong Liu, L.T., K.C., M.W., X.C., N.Y.,
D.T., N.X., J.H., Q.Z. and P.G. assisted with the experiments. D.H. performed mammary gland whole-mount analysis. D.H., Y.Z., Yang He, P.X. and Yanlin He assisted with the manuscript
writing. Yanlin He supervised electrophysiological studies. C.W. performed the stereotaxic injection of the virus and supervised the metabolic studies. Yanlin He and C.W. conceived, designed
and supervised the project. CORRESPONDING AUTHORS Correspondence to Yanlin He or Chunmei Wang. ETHICS DECLARATIONS COMPETING INTERESTS The authors declare no competing interests. PEER
REVIEW PEER REVIEW INFORMATION _Nature Metabolism_ thanks the anonymous reviewers for their contribution to the peer review of this work. Primary Handling Editors: Jean Nakhle and Ashley
Castellanos-Jankiewicz, in collaboration with the _Nature Metabolism_ team. ADDITIONAL INFORMATION PUBLISHER’S NOTE Springer Nature remains neutral with regard to jurisdictional claims in
published maps and institutional affiliations. EXTENDED DATA EXTENDED DATA FIG. 1 LACTATIONAL HYPERPHAGIA AND HYPERPROLACTINEMIA. (A) Serum levels of PRL in virgin and lactating female mice
(n = 6). (B-E) The correlation between the number of pups and food intake (B), PRL levels (C), or BAT size (D), or UCP1 (E) in the BAT in lactating dams (B, n = 44; C, n = 7; D, n = 5; E, n
= 5). Data are presented as mean ± SEM and/or individual data points. A, two-tailed unpaired t-tests. B-E, linear regression with 95% confidence. P values are shown in figures. Source data
EXTENDED DATA FIG. 2 LACTATIONAL SUPPRESSION OF ERΑMBH NEURONS. (A) Spontaneous firing frequency and resting membrane potential of ERαvlVMH neurons in virgin (n = 37), pregnancy (gestation
day 16-18, n = 18) and lactating mice on PPD1 (n = 37), PPD 5 (n = 31), PPD 10 (n = 27) and PPD 20 (n = 22). Neurons from 3 mice in each group. (B-E) Individual ERαvlVMH neurons (B), ERαARH
neurons (C), ERαPOA neurons (D), ERαPVN neurons (E), responses to PPT as indicated by the increased firing frequency and resting membrane potential in virgin, late pregnancy (G18) and
lactating mice on PPD2 and 5. Fractions indicate the responsive neuron number out of total recorded neurons. Neurons from 3 mice in each group. (F) Percentage of spontaneous
firing/PPT-responsive, firing/PPT-irresponsive, silent/PPT-responsive and silent/PPT-irresponsive ERαvlVMH subpopulations from virgin, gestation day 18 and PPD5 mice. (G) Representative
traces of ERαvlVMH neurons excited by PPT in virgin and lactating female mice. (H) The percentage of ERα, ERβ, and GPER neurons in total VMH neurons from female mice indicated by secondary
analysis of scRNA-Seq data60,67. (I) Serum levels of E2 in females during peri-parturition and lactation (peri-parturition, n = 5; PPD2, n = 4; PPD5, n = 4; PPD9, n = 4; virgin, n = 3). Data
are presented as mean ± SEM and/or individual data points (A-E and I), or as part of whole bar (F) or Pie graphs (H). A, groups vs Virgin in ordinary one-way ANOVA followed by Dunnett’s
multiple comparisons test. I, ordinary one-way ANOVA followed by Šídák’s multiple comparisons test. B-E, “n” is indicated in figures. P values are shown in figures. Source data EXTENDED DATA
FIG. 3 DELETION OF ERΑ FROM MBH NEURONS CAUSES HYPERPROLACTINEMIA AND METABOLIC CHANGES. (A) Immunostaining of ERα in virgin female control and ERαMBH-KO mice with deletion of ERα from the
entire vlVMH but not the ARH. (B–C) Body size (B) and food intake (C) of virgin female control (n = 6) and ERαMBH-KO mice (n = 7). (D) Food efficiency (body weight gain/accumulated food
intake) of virgin female control and ERαMBH-KO mice by 16 days after virus injection (n = 6). (E-F) Body weight (E) and food intake (F) in virgin female control and ERαMBH-KO mice before
they entered the TSE metabolic cages, 2 weeks after virus injection (control, n = 8; ERαMBH-KO, n = 7). (G-I) Temporal levels of O2 consumption during the dark cycle and light cycle within 3
days (G), ANCOVA analysis of O2 consumption body weight as a covariant66,70 (H) and predicted O2 consumption at a presumed body weight (I) in virgin female control and ERαMBH-KO mice
measured by the TSE PhenoMaster metabolic cages (control, n = 8; ERαMBH-KO, n = 6). (J-L) Temporal levels of CO2 consumption during the dark cycle and light cycle within 3 days (J), ANCOVA
analysis of CO2 consumption body weight as a covariant (K) and predicted CO2 consumption at a presumed body weight (L) in virgin female control and ERαMBH-KO mice measured by the TSE
PhenoMaster metabolic cages66,70 (control, n = 8; ERαMBH-KO, n = 6). (M-O) Serum PRL levels (M), body weight (N) and food intake (O) of control WT mice receiving AAV-Cre or AAV-GFP virus
into the vlVMH (n = 5). Data are presented as mean ± SEM and/or individual data points. D, E, I and L, two-tailed unpaired t-tests. H and K, ANCOVA analysis by CalR. P values are shown in
figures. Source data EXTENDED DATA FIG. 4 DELETION OF ERΑ FROM MBH NEURONS CAUSES HYPERPROLACTINEMIA AND NEUROENDOCRINOLOGY CHANGES. (A) Immunohistochemistry staining of pSTAT5 in the
hypothalamus of virgin female control and ERαMBH-KO mice. 3 V, third ventricle. (B) H&E staining of the pituitary and immunofluorescence of PRL in the pituitary of virgin female control
and ERαMBH-KO mice. The scale bar is 200 μm. (C) Boxed areas of (B) are magnified as indicated. The scale bar is 50 μm. (D) Immunohistochemistry staining of ERα in the hypothalamus of virgin
female control and ERαMBH-KO mice 4 months (2 months chow diet followed by 2 months HFD) after surgery. The scale bar is 200 μm. (E-H) Serum levels of GH (E), IGF1 (F), ACTH (G) and TSH (H)
in virgin female control (n = 6) and ERαMBH-KO (E, n = 8; F, G, H, n = 7) mice 4 months (2 months chow diet followed by 2 months HFD) after surgery. (I) Serum levels of TSH in virgin female
control and ERαMBH-KO mice on chow diet 6 weeks after surgery (n = 8). (J) H&E staining of iWAT in virgin female control and ERαMBH-KO mice by 4 months after surgery. The scale bar is
500 or 100 μm. (K-M) Serum levels of P4 (K), LH (L) and FSH (M) in virgin female control (K, n = 4; L, n = 6; M, n = 6) and ERαMBH-KO (K, n = 6; L, n = 7; M, n = 7) mice 4 months (2 months
chow diet followed by 2 months HFD) after surgery. Data are presented as mean ± SEM and/or individual data points. H, two-tailed unpaired t-tests. P value is shown in the figure. Source data
EXTENDED DATA FIG. 5 DELETION OF ERΑ FROM VLVMH NEURONS INCREASES PRL LEVELS. (A) Immunofluorescence staining of ERα in the hypothalamus of control side and ¼ ERαvlVMH-KO side from one
virgin female mouse. The scale bar is 200 μm. (B) Quantification of ERα deletion in the half vlVMH KO side compared to the control side (n = 5). (C) The appearance of mammary glands in
female virgin control and virgin ¼ ERαvlVMH-KO mice. (D) Body weight at 12 weeks after virus injection and (E) average of 4 days-food intake of virgin control and ¼ ERαvlVMH-KO mice by 12
weeks after virus injection (n = 5). (F) Serum PRL level of virgin control and ¼ ERαvlVMH-KO mice at 6 weeks and 12 weeks after virus injection (n = 5). Data are presented as mean ± SEM and
individual data points. F, ordinary one-way ANOVA followed by Dunnett’s multiple comparisons test. P values are shown in the figure. Source data EXTENDED DATA FIG. 6 ACTIVATION OF ERΑVLVMH
NEURONS INHIBITS LACTATIONAL HYPERPROLACTINEMIA AND HYPERPHAGIA. (A) Bright-field illumination and fluorescence for mCherry of a recorded ERαvlVMH neuron in a brain slice of ERαvlVMH-hM3Dq
mouse (up), and a representative trace of ERαvlVMH neuron in ERαvlVMH-hM3Dq mouse treated with CNO (down). The scale bar is 20 μm. (B) The spontaneous firing frequency (n = 6) and resting
membrane potential (n = 8) of ERαvlVMH neurons before and after treatment of CNO, and after the wash out of CNO. (C) c-fos expression in the MBH of a ERα-Cre mouse that received the
injection AAV-DIO-hM3Dq-mCherry virus into one side of the vlVMH (hM3Dq-mCherry) and the injection of control AAV-DIO-mCherry virus into the other side of the vlVMH. Increased c-fos
expression in the hM3Dq-mCherry side indicates activation of ERαvlVMH neurons, compared to the control mCherry side 2 hours after injection of CNO. Black arrows indicate magnified images.
White arrows indicate neurons that co-express hM3Dq-mCherry and c-fos in the magnified picture of the white boxed area. Scale bar is 200 or 100 μm. (D) The c-fos quantification results of
(C) (n = 4). (E) Serum PRL levels of control (n = 12) and ERαvlVMH-hM3Dq (n = 14) lactating dams on PPD2. (F) Serum PRL levels of control (n = 12) and ERαvlVMH-hM3Dq (n = 10) lactating dams
on PPD9. (G-I) Body weight (G), daily food intake (H) and BAT temperature (I) of control (G, n = 15; H, n = 12; I, n = 5) and ERαvlVMH-hM3Dq (G and H, n = 11; I, n = 5) mice during pregnancy
before CNO injection. (J-M) Body weight (J), daily food intake (K), BAT temperature (L) and averaged body weight of pups (M) in control (J, n = 15; K, n = 14; L, n = 10; M, n = 8) and
ERαvlVMH-hM3Dq (J, n = 21; K, n = 15; L, n = 7; M, n = 9) mice following the injection of CNO during lactation (excluding those with loss of all pups). (N-P) Maternal care time spent on
nursing (N), grooming (O) and nest building (P) of control (n = 5) and ERαvlVMH-hM3Dq lactating dams (n = 4) on PPD5. Data are presented as mean ± SEM and/or individual data points. B,
two-tailed paired t-tests. D, two-tailed unpaired t-tests. E and F, two-way RM ANOVA by multiple comparisons test with uncorrected Fisher’s LSD. K, two-way ANOVA followed by Šídák’s multiple
comparisons test with Geisser-Greenhouse correction. P values are shown in figures. Source data EXTENDED DATA FIG. 7 METABOLIC CHANGES INDUCED BY CENTRAL OR SYSTEMIC SUPPLEMENT OF E2 DURING
LACTATION. (A-B) The number of pups survived with systemic (A) or central supplement (B) of E2. (C-D) Body weight of lactating dams with systemic (C) or central (D) supplement of E2. (E-F)
Daily food intake of lactating dams with systemic (E) or central (F) supplement of E2. (G-H) The average body weight of pups raised by lactating dams with systemic (G) or central (H)
supplement of E2. (systemic: vehicle, n = 15; E2 0.5 ug/day, n = 7; E2 2.4 ug/day, n = 10. central: n = 5). (I-J) H&E staining of mammary glands enmeshed in the iWAT of lactating dams
with systemic supplement of vehicle (I) or E2 (J). Data are presented as mean ± SEM. A, E and G, two-way ANOVA followed by Šídák’s multiple comparisons test with Geisser-Greenhouse
correction. P values are shown in figures. Source data EXTENDED DATA FIG. 8 LACTATIONAL REMODULATION OF GENE PROFILES OF ERΑVLVMH NEURONS. (A) Electrophysiology recordings of the three
ERαvlVMH subpopulations (virgin firing vs PPD5 firing vs PPD5 silent) subjected to Patch-Seq. Resting membrane potential (left) and firing frequency (right) of these three ERαvlVMH
subpopulations and their responsiveness to PPT (n = 10, neurons from 3 mice per subpopulation). (B-C) Volcano plot and (B) GO analysis (C) of the differentially expressed genes between
virgin firing ERαvlVMH neurons and PPD5 firing ERαvlVMH subpopulation. (D-E) Volcano plot and (D) GO analysis (E) of the differentially expressed genes between PPD5 firing subpopulation and
PPD5 silent ERαvlVMH subpopulation. (F) Heatmap of Patch-Seq-identified genes that potentially mediate ERα downstream signal pathways. (G) Secondary analysis of published scRNA-Seq data of
VMH neurons60 identified 4 potassium channels that displayed the same changes as Patch-Seq data. These 4 genes were expressed at higher levels in lactating ERαVMH neurons compared to virgin
ERαVMH neurons or lactating non-ERαVMH neurons (virgin ERα+, n = 3932; lactating ERα+, n = 5462; virgin ERα-, n = 9373; lactating ERα-, n = 9776; all information in the SUPPLEMENTARY TABLE
1). (H) Percentage of ERαVMH neurons out of the whole VMH neurons in virgin and lactating female mice based on the secondary analysis of published scRNA-Seq data60(n = 7). (I) Heatmap of
Patch-Seq-identified inhibitory channels between OVX-V and OVX-E mice by reanalyzing published RNA-Seq data from the VMH of OVX-V and OVX-E mice18. Data are presented as mean ± SEM and/or
individual data points. A-bar graphs, one-way ANOVA followed by Dunnett’s multiple comparisons test or two-tailed unpaired t-tests. G, Wald test followed by Bonferroni correction. P values
are shown in figures. Source data EXTENDED DATA FIG. 9 LACTATIONAL REMODULATION OF PRL SIGNALING IN ERΑVLVMH NEURONS. (A-B) Secondary analysis of the population (A) and expression (B) of
Prlr in the VMH60(n = 7). CPM, counts per million. (C) Secondary analysis of the expression of Prlr in OVX-V (n = 3) vs OVX-E2 (n = 4) female mice18. Data are presented as mean ± SEM and
individual data points. (D) The expression of Prlr, Stat5a, Stat5b and receptors of other sex hormones in ERαVMH neurons from our Patch-Seq data. (E) Graphic summary. Lactational suppression
of ERαvlVMH neurons suppresses BAT thermogenesis, promotes feeding and maintains lactational hyperprolactinemia. Created in BioRender. Wang, C. (2025) https://BioRender.com/i72c521. Source
data SUPPLEMENTARY INFORMATION SUPPLEMENTARY INFORMATION Supplementary Table 1. REPORTING SUMMARY SOURCE DATA SOURCE DATA FIG. 1 Statistical Source Data. SOURCE DATA FIG. 1 Unprocessed
western blot image. SOURCE DATA FIG. 2 Statistical Source Data. SOURCE DATA FIG. 3 Statistical Source Data. SOURCE DATA FIG. 4 Statistical Source Data. SOURCE DATA FIG. 5 Statistical Source
Data. SOURCE DATA FIG. 6 Statistical Source Data. SOURCE DATA FIG. 8 Statistical Source Data. SOURCE DATA EXTENDED DATA FIG. 1 Statistical Source Data. SOURCE DATA EXTENDED DATA FIG. 2
Statistical Source Data. SOURCE DATA EXTENDED DATA FIG. 3 Statistical Source Data. SOURCE DATA EXTENDED DATA FIG. 4 Statistical Source Data. SOURCE DATA EXTENDED DATA FIG. 5 Statistical
Source Data. SOURCE DATA EXTENDED DATA FIG. 6 Statistical Source Data. SOURCE DATA EXTENDED DATA FIG. 7 Statistical Source Data. SOURCE DATA EXTENDED DATA FIG. 8 Statistical Source Data.
SOURCE DATA EXTENDED DATA FIG. 9 Statistical Source Data. RIGHTS AND PERMISSIONS Springer Nature or its licensor (e.g. a society or other partner) holds exclusive rights to this article
under a publishing agreement with the author(s) or other rightsholder(s); author self-archiving of the accepted manuscript version of this article is solely governed by the terms of such
publishing agreement and applicable law. Reprints and permissions ABOUT THIS ARTICLE CITE THIS ARTICLE Yu, M., Feng, B., Bean, J.C. _et al._ Suppression of hypothalamic oestrogenic signal
sustains hyperprolactinemia and metabolic adaptation in lactating mice. _Nat Metab_ 7, 759–777 (2025). https://doi.org/10.1038/s42255-025-01268-z Download citation * Received: 28 June 2024 *
Accepted: 07 March 2025 * Published: 10 April 2025 * Issue Date: April 2025 * DOI: https://doi.org/10.1038/s42255-025-01268-z SHARE THIS ARTICLE Anyone you share the following link with
will be able to read this content: Get shareable link Sorry, a shareable link is not currently available for this article. Copy to clipboard Provided by the Springer Nature SharedIt
content-sharing initiative