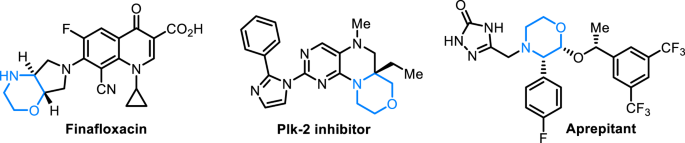
- Select a language for the TTS:
- UK English Female
- UK English Male
- US English Female
- US English Male
- Australian Female
- Australian Male
- Language selected: (auto detect) - EN
Play all audios:
ABSTRACT Morpholines and morpholinones are important building blocks in organic synthesis and pharmacophores in medicinal chemistry, however, C3-disubstituted morpholines/morpholinones are
extremely difficult to access. Here we show the ZnCl2-catalyzed cyclizative 1,2-rearrangement for the efficient synthesis of morpholinones bearing aza-quaternary stereocenters. A series of
structurally diverse C3-disubstituted morpholin-2-ones which are difficultly accessible by existing methods were efficiently constructed from readily available two achiral linear compounds.
Notably, mechanistic studies reveal that this reaction proceeds via an unusual sequence of direct formal [4 + 2] heteroannulation regioselectively delivering specific α-iminium/imine
hemiacetals followed by a 1,2-esters or amides shift process, which is different from the reported mechanism of the aza-benzilic ester rearrangements. SIMILAR CONTENT BEING VIEWED BY OTHERS
ASYMMETRIC SYNTHESIS OF _N_-BRIDGED [3.3.1] RING SYSTEMS BY PHOSPHONIUM SALT/LEWIS ACID RELAY CATALYSIS Article Open access 18 January 2022 STEREOSPECIFIC MOLECULAR REARRANGEMENT VIA
NUCLEOPHILIC SUBSTITUTION AT QUATERNARY STEREOCENTRES IN ACYCLIC SYSTEMS Article 19 March 2025 DESIGN, DEVELOPMENT AND APPLICATIONS OF COPPER-CATALYZED REGIOSELECTIVE (4 + 2) ANNULATIONS
BETWEEN DIARYLIODONIUM SALTS AND ALKYNES Article Open access 08 November 2022 INTRODUCTION C-substituted morpholines and morpholinones represent important building blocks1,2 in organic
synthesis and pharmacophores in medicinal chemistry, such as commercially available drugs of Finafloxacin3, Plk-2 inhibitor4 and Aprepitant5 (Fig. 1). Consequently, efficient access to these
structural targets have been in great demand. Thus far, there have been a number of methods for the synthesis of C-functionalized morpholines or morpholinones6,7,8 benefiting from
metal-catalyzed cyclization reactions9,10,11,12,13,14, allylic alkylation15, hydrogenations16,17 or other multi-step transformations18,19,20. On the contrary, approaches for the catalytic
preparation of C3-disubstituted morpholines/morpholin-2-ones are far less developed, which is due probably to the steric hindrance effect of the incoming aza-quaternary carbon and
availability of the corresponding branched starting materials21,22,23. Furthermore, asymmetric synthesis of such compounds was envisioned to be even more challenging, which must overcome the
formidable challenges in both reaction reactivity and stereoselectivity. In this regard, an elegant work by Carreira and coworkers dealing with ring expansion of 3-oxetanone-derived
spirocycles under In(III) catalysis is noteworthy (Fig. 2A)24. Mechanistically, the newly formed aza-quaternary carbon results from the Strecker reaction25 of oxazolidine with trimethylsilyl
cyanide, and a subsequent stereoselective desymmetrizing 6-_exo_-tet cyclization finally affords morpholine in high dr value. To the best of our knowledge, so far, this is the only example
of asymmetric synthesis of morpholine derivative with an aza-quaternary carbon center which is in situ formed rather than originates from a substrate. Thus, the highly useful and efficient
approaches for the de novo construction of structurally diverse C3-disubstituted morpholines/morpholin-2-ones from readily available linear compounds are highly desirable. Condensation of
arylglyoxals with vicinal amino alcohols delivering 2-acyloxazolidines which further isomerize to morpholinones is well-known26,27,28,29,30. Recently, this reaction has been applied to the
diastereoselective synthesis of morpholinones by Walczak group31. Very recently, we demonstrated an enantioselective aza-benzilic ester rearrangement for the synthesis of enantioenriched
morpholinones by chiral Brønsted acid (Fig. 2B)32. In both cases, however, only morpholinones bearing aza-tertiary stereocenters have been successfully synthesized while all efforts aimed at
the construction of C3-disubstituted morpholin-2-ones afford either oxazolidine intermediates or degradation at higher temperature31,32. To the best of our knowledge, 1,2-rearrangement for
the construction of morpholinones bearing aza-quaternary stereocenters has not been achieved in spite of the significant synthetic importance of the resulting compounds33,34. In connection
with our ongoing research interest in developing catalytic asymmetric cyclizative rearrangement (CACR)32,35, we herein report the successful realization of this endeavor, which delivers a
wide range of C3-disubstituted morpholin-2-ones 3 from readily available linear 2,3-diketoesters 1 and vicinal amino alcohols 2 under the catalysis of ZnCl2 complex (Fig. 2C). Moreover, a
highly diastereoselective version of such transformation has also been achieved by employing chiral vicinal amino alcohols as reaction partners. More importantly, rather than previously
reported formal [4 + 1] oxazolidination/ring-expansive hemiacetalization/1,2-aryl or alkyl shift cascade (Fig. 2B)32,35, mechanistic investigations indicated that this transformation
proceeded via a completely different reaction pathway consisting of an initial direct [4 + 2] cyclization (INT) followed by a 1,2-ester/amide shift process (Fig. 2C)36. RESULTS REACTION
DEVELOPMENT With our on-going efforts to further examine its reactivity profile35,36, the multi-functionalizable vicinal tricarbonyl compounds37,38,39,40,41,42,43,44,45,46,47 were chosen as
the reaction partner with aminoethanols. Our studies commenced with a mixture of ethyl 2,3-dioxo-3-phenylpropanoate and its hydrate (1A, 1.0 equiv), 2-((4-methoxyphenyl)amino)ethan-1-ol 2A
(1.2 equiv) in 1,2-dichloroethane (_c_ 0.1 M) at 80 °C under argon. Initially, screening of various strong Brønsted acids, such as trifluoroacetic acid, trifluoromethanesulfonic acid, and
_p_-toluenesulfonic acid, failed to give the rearranged products (entries 1-3, Table 1). Additionally, even aqueous hydrochloric acid could not give the desired product. As typical
bifunctional catalysts, chiral phosphoric acids (CPA)48,49 could not give the rearranged product 3A as well. Boron trifluoride-diethyl etherate (BF3 ∙ Et2O) was also ineffective (entry 4).
Screening of a series of strong Lewis acids, such as Mg(OTf)2, InCl3, and Zn(II) complexes (entries 5-10), in the presence of tricarbonyl compound 1A and amino alcohol 2A at 80 °C resulted
in the formation of the desired C3-disubstituted morpholin-2-one 3A, and ZnCl2 gave the best result (55% yield). Using ZnCl2 as catalyst, the reaction conditions were further optimized by
varying the solvent (entries 11–13), catalyst loading (entry 14), and reaction temperature (entries 15–16). Overall, the best conditions consisted of performing the reaction in DCE (_c_ 0.1
M) at 100 °C in the presence of ZnCl2 (0.2 equiv). Under these conditions, C3-disubstituted morpholin-2-one 3A was isolated in 61% yield (entry 15). SUBSTRATE SCOPE EXPLORATION With the
optimized conditions in hand, this ZnCl2-catalyzed cyclizative 1,2-rearrangement was applicable to a wide range of substrates (Fig. 3). Regarding the aryl group of 3-aryl substituted
2,3-diketoesters, the presence of electron-neutral (H, Ph), electron-donating (MeO) groups and electron-withdrawing (F, CF3, CO2Et) at _para_ and _meta_ positions of the phenyl ring were
well tolerated (3A-3J). Unfortunately, an aryl group bearing an _ortho_ substituent failed to give the desired product which is due probably to the steric hinderance effect. The structure of
compound 3A was confirmed by X-ray crystallographic analysis (see Supplementary Data 1). In the presence catalytic amount of ZnCl2, (_S_)-(+)-Ibuprofen derived 2,3-diketoester could give
the corresponding C3-disubstituted morpholin-2-one 3G in moderate yield. Moreover, arylcoupled Aspirin could be smoothly functionalized with the present methodology (3H). 3,4-Dichlorophenyl,
2-naphthyl, and even heterocycles, such as benzo[_d_][1,3]dioxole, 3-thiophene and 2-thiophene, were transferred without event (3K-3O). To our delight, with aliphatic 2,3-diketoesters
(1P-1S), these rearrangement reactions efficiently afforded the corresponding product 3P-3S as well. Interestingly, instead of vicinal tricarbonyl compounds, 1,2-diketone bearing CF3 group
(1T)50 could also give the desired rearranged product (3T) with decreased yield. Furthermore, 2,3-diketoesters derived from different alcohols, such as methyl, isopropyl, and benzyl, were
all well-accepted substrates (3U-3W). Gratefully, 2,3-diketoamides participated in this ZnCl2-catalyzed cyclizative 1,2-rearrangement to provide the corresponding rearranged products 3X and
3Y in high yields. Performing the gram scale reactions of 1Y (6.78 mmol) and 2A (8.14 mmol) in the presence of ZnCl2 (20 mol%) afforded 3Y without obvious erosion of yield (75%).
L-(-)-Menthol derived 2,3-diketoesters gave the corresponding product 3Z in good yield as well. Alternatively, other _N_-substituted amino alcohols bearing phenyl, 2-methylphenyl, methyl and
benzyl groups were also examined, affording the corresponding products 3AA-3AD without event. Notably, amino alcohol bearing electron-withdrawing protecting group on nitrogen, such as Ts
and Ns, failed to afford the desired product 3. Furthermore, _N_-unsubstituted amino alcohols could give the corresponding rearranged products (3AE-3AF) smoothly. STRATEGY EXTENSION
Asymmetric catalytic construction of multi-functionalized morpholin-2-ones bearing aza-quaternary stereocenters represents a formidable challenge. To further exploit the structural diversity
of the products, we divert our attention to the diastereoselective synthesis of C3-disubstituted morpholin-2-ones by employing optically pure _N_-substituted 2-aminoethan-1-ols. Gratefully,
without any modifications to the reaction conditions, this protocol was applicable to a wide range of chiral amino alcohols delivering structurally diverse products bearing
multi-stereocenters in high stereoselectivities (Fig. 4). Various _N-_substituted 2-aminoethan-1-ol, such as (_S_)-2-aminopropan-1-ol, (_R_)-2-amino-3-phenylpropan-1-ol and
(_R_)-2-amino-3-methylbutan-1-ol, underwent this rearrangement reaction smoothly affording the desired products 3AG-3AI in high diastereoselectivities. 2,3-Diketoamides 1AJ and 1AK were also
well tolerated leading to the formation of the products 3AJ and 3AK in good yields with excellent stereoselectivities (>20/1 d.r.). Moreover, C2-phenyl substituted amino alcohol 1AL
afforded the product 3AL in excellent d.r. value as well. _N_-benzyl substituted amino alcohol 1AM delivered decreased stereoselectivity (6/1 d.r.). Subsequently, Other more challenging
chiral amino alcohols were further examined. Interestingly, even _N_-PMP substituted (_S_)-1-aminopropan-2-ol 1AN where the original stereocenter was far away from the newly formed
aza-quaternary carbon could also afford the product 3AN in good diastereoselectivity (4/1 d.r.). Substrates 1AO and 1AP derived from cyclic amino alcohols underwent the cyclizative
1,2-rearrangement without event, which furnished the corresponding products (3AO-3AP) bearing three stereocenters in excellent and good diastereoselectivities. The absolute configurations of
the products 3AG, 3AM, 3AN and 3AO were determined to be (3_S_,5_S_), (3_R_,5_R_), (3_S_,6_S_), and (3 _R_,4a_R_,8a_R_), respectively (see Supplementary Data 2–5). Consequently, the
absolute configurations for other morpholinones (3AH-3AL and 3AP) were assigned accordingly (Fig. 4). Unfortunately, all efforts aimed at developing a catalytic enantioselective version of
this rearrangement gave almost racemic products (see Supplementary Table S1 for details). MECHANISTIC STUDIES A series of control experiments were performed to gain insight into the reaction
mechanism. In the presence of _p_-toluenesulfonic acid (_p_-TSA), a formal [3 + 2] reaction of 1A with 2A occurred at 80 °C affording an oxazolidine compound 4A in moderate yield (Fig. 5a).
Further treating intermediate 4A with catalytic amount of _p_-TSA (20 mol%) gave trace of the product 3A (Fig. 5a). To our surprise, however, resubmitting 4A to the standard reaction
conditions also afforded only trace of the rearranged product 3A with concurrent formation of a linear compound 5 in 43% yield and some decomposition species (Fig. 5b). As 2-aminoethan-1-ol
derivatives 2 are capable of serving as bidentate ligands to Zn(II)51, we further examine the reactivity of (2A)∙ZnCl2 complex. Under the catalysis of the in situ formed (2A)∙ZnCl2 complex,
the reaction of the intermediate 4A gave product 3A in very low yield (10%) and a linear compound 5 was formed as a main product (Fig. 5c). Alternatively, a set of experiments were performed
with 2,3-diketoester 1A and amino alcohol 2A under the standard conditions and the yields of both 3A and 4A were detected at different time. As it is seen clearly from the diagram shown in
Fig. 5d, the yield of 3A increased steadily as the reaction proceeded with the concurrent formation of the low yield of 4A. These results revealed that, in this case, oxazolidine
intermediate 4A resulted from formal [4 + 1] condensation of 1A and 2A is not a major reservoir for product 3A, which is different from the reported Brønsted acid catalyzed aza-benzilic
ester rearrangements31,32. Notably, during the screening of the reaction conditions, another benzilic ester rearrangement product 636 was observed in the presence of catalytic amount of
Zn(OTf)2 (Fig. 6a). However, ZnCl2 complex only gave a trace of compound 6 (Fig. 6b). Furthermore, resubmitting 6 to our standard reaction conditions failed to give the desired product 3A
(Fig. 6c). These results indicate that an intramolecular aza-cyclization to the final product 3A via a possible in situ formed carbocation intermediate 6’ derived from dehydration of 6 could
be excluded. Subsequently, we realized that, at the first step of this tandem reaction, a direct formal [4 + 2] reaction might be competitive to the [4 + 1] cyclization of 1A and 2A. All
our efforts aimed at trapping the key intermediate of the rearrangement by employing various excessive nucleophilic reagents failed to give the corresponding products. To our delight, when
the reaction of 1A and _N_-unsubstituted amino alcohol 2AE was performed at lower temperature (60 °C), a unique α-imine hemiacetal intermediate 7 was successfully isolated in good yield
(Fig. 6d). Surprisingly, the imine moiety was formed from the condensation of primary amine with the carbonyl group linked to phenyl rather than with the middle one, which is also different
from the previous reports31,32,35. Notably, no oxazolidine intermediate 4AE was detected throughout the whole reaction process26,27,28,29,30. Interestingly, a predictable regioisomer 8
resulted from the formal [4 + 2] reaction was actually not observed as well, which is due probably to the relatively high thermodynamic stability of the α-imine hemiacetal 7. Importantly,
under the standard condition, the six-membered ring compound 7 underwent the rearrangement reaction smoothly, which delivered the corresponding product 3AE in high yield (Fig. 6e). In the
absence of ZnCl2 catalyst, intermediate 7 failed to give 3AE. The reaction of 1A with another branched amino alcohol 2AF gave similar results (Fig. 6f, g). The structure of the important
α-imine hemiacetal 9 was further confirmed by X-ray single crystal diffraction technique (see Supplementary Data 6). These results clearly suggested that a six-membered ring intermediate
resulting from a direct formal [4 + 2] cyclization should be the real reservoir for product 3. Moreover, treating compound 9 with catalytic amount of either _p_-TSA or (_R_)-TRIP-CPA at 100
°C failed to yield the product 3AF, which not only underlined the great importance of ZnCl2 catalyst in triggering the key 1,2-ester shift step but also highlighted the difference in the
reaction mechanism between current rearrangement and Brønsted acid-catalyzed benzilic ester-type rearrangement (Fig. 6h)31,32,35. Finally, the reaction of diphenyl-substituted 1,2-diketone
10 with the amino alcohol 2A gave neither the rearranged product nor any intermediates (Fig. 6i), which indicated that the extra ester group is of vital importance for this rearrangement.
For all NMR spectra of the new compounds, see Supplementary Data 7. As the conversion of 1 and 2 to the five-membered oxazolidine intermediates 4 is extremely low, and the transformation of
4 to the products 3 is also highly difficult (see Fig. 5b–d), the compound 4 should be not the preferred intermediates under the conditions. Moreover, the isolation of the only [4 + 2]
cyclization products 7 and 9 which could be smoothly transformed into the rearranged products 3AE and 3AF clearly indicates the real reaction pathway as well. Therefore, on the basis of the
mechanistic experiments, this rearrangement reaction is proposed to proceed via an unprecedent direct [4 + 2] cyclization/1,2-ester or amide migration sequence (Fig. 7a). Initially, the
2,3-diketoester 1A and amino alcohol 2A predominately undergo a formal [4 + 2] heteroannulation reaction to generate a cyclic α-iminium hemiacetal A. Subsequently, the key intermediate A
then participate in a 1,2-ester shift reaction under the catalysis of in situ formed (2A)∙ZnCl2 complex affording the final C3-disubstituted morpholin-2-one 3A. The in situ formed a small
amount of 4A is more inclined to generate compound 5 rather than the rearrangement product 3A, which is due probably to the driving force differences to these two compounds, respectively.
The side product 5 might result from the carbon-carbon cleavage of intermediate B to produce a carbene species which is quenched by water and a potential hydride transfer reagent 2A52,53. A
plausible pathway leading to the observed stereochemical outcome is also illustrated in Fig. 7b. Because the ester moiety of the intermediate A1 is remote from benzyl group, the _Re_ face of
the iminium moiety is open to the ester group 1,2-migration, thus favorably affording 3AH. In contrast, the _Si_ face of the iminium moiety is shielded by benzyl group in A2, thus making
the 1,2-ester shift disfavored and resulting in the formation of the enantiomer _ent_-3AH in a minor amount. This scenario is in accordance with the configuration of the products as
established by X-ray crystal structure analysis of four representative morpholines (3AG, 3AM, 3AN and 3AO, see Fig. 4). In conclusion, we have developed the first examples of ZnCl2-catalyzed
cyclizative 1,2-rearrangement for the asymmetric synthesis of morpholinones bearing aza-quaternary stereocenters which are far more challenging to obtain efficiently by existing methods. A
series of structurally diverse C3-disubstituted morpholin-2-ones were efficiently constructed from readily available two achiral linear compounds. Importantly, on the basis of mechanistic
studies, this rearrangement proceeds via an unprecedent sequence of direct formal [4 + 2] heteroannulation followed by a 1,2-ester or amide migration process, which is highly distinct from
the reported reaction mechanism of aza-benzilic acid-type rearrangements. METHODS (SUPPLEMENTARY METHODS IN THE SI) GENERAL PROCEDURE FOR THE SYNTHESIS OF 3 To a mixture of 1 (0.1 mmol, 1.0
equiv), 2 (0.12 mmol, 1.2 equiv), and ZnCl2 (0.02 mmol, 0.2 equiv) was added 1,2-dichloroethane (1.0 mL) under argon. The reaction mixture was stirred at 100 °C for indicated hours. After
completion of the reaction (monitored by TLC), the solvent was removed under vacuum. The residue was purified by column chromatography on silica gel, eluting with petroleum ether/ethyl
acetate to afford the products 3 in moderate to high yields. DATA AVAILABILITY Cif. files (see Supplementary Data 1–6); all NMR spectra of new compounds (see Supplementary Data 7). All data
generated and analyzed during this study are included in this article, its Supplementary Information, and also available from the authors upon reasonable request. The X-ray crystallographic
coordinates for structures reported in this Article have been deposited at the Cambridge Crystallographic Data Centre (CCDC), under deposition number CCDC 2225996, 2246927, 2246928, 2246926,
2246925 and 2266070 (see Supplementary Notes and Supplementary Tables S2-43 for details). These data can be obtained free of charge from The Cambridge Crystallographic Data Centre via
www.ccdc.cam.ac.uk/data request/cif. REFERENCES * Enders, D., Meyer, O., Raabe, G. & Runsink, J. (_S,S_)-3,5-Dimethylmorpholine, a novel _C_2-Symmetric auxiliary. First application in
[4+2]-Cycloadditions leading to 4-Oxohexahydropyridazine derivatives. _Synthesis_ 1994, 66–72 (1994). Article Google Scholar * Baldoli, C. et al. The first chiral racemic Fischer-type
aminocarbene complex bearing a C2 symmetry amine: stereochemical characteristics and application to Michael addition reactions. _J. Organomet. Chem._ 486, 279–282 (1995). Article CAS
Google Scholar * Flick, A. C. et al. Synthetic approaches to the 2014 new drugs. _Bioorg. Med. Chem._ 24, 1937–1980 (2016). Article CAS PubMed Google Scholar * Aubele, D. L. et al.
Selective and brain-permeable polo-like Kinase-2 (Plk-2) inhibitors that reduce a-Synuclein phosphorylation in rat brain. _ChemMedChem_ 8, 1295–1313 (2013). Article CAS PubMed Google
Scholar * Nelson, T. D. Synthesis of Aprepitant. In _Strategies and Tactics in Organic Synthesis_; Harmata, M., Ed.; Academic Press, 2005; Vol. _6_, pp 321–351. * Wijtmans, R. et al.
Biological relevance and synthesis of C-Substituted morpholine derivatives. _Synthesis_ 2004, 641–662 (2004). Article Google Scholar * Trstenjak, U., Ilaš, J. & Kikelj, D. Advances in
the synthesis of Morpholin-3-ones and morpholin-2-ones. _Synthesis_ 44, 3551–3578 (2012). Article CAS Google Scholar * Yamada, R., Sakata, K. & Yamada, T. Electrochemical synthesis of
substituted morpholines via a decarboxylative intramolecular etherification. _Org. Lett._ 24, 1837–1841 (2022). Article CAS PubMed Google Scholar * Uozumi, Y., Tanahashi, A. &
Hayashi, T. Catalytic asymmetric construction of morpholines and piperazines by palladium-catalyzed tandem allylic substitution reactions. _J. Org. Chem._ 58, 6826–6832 (1993). Article CAS
Google Scholar * Wilkinson, M. C. Asymmetric synthesis of an aminomethyl morpholine via double allylic substitution. _Tetrahedron Lett._ 46, 4773–4775 (2005). Article CAS Google Scholar
* Bandini, M., Monari, M., Romaniello, A. & Tragni, M. Gold catalyzed direct activation of allylic alcohols in the stereoselective synthesis of functionalized 2-Vinyl-Morpholines.
_Chem. Eur. J._ 16, 14272–14277 (2010). Article CAS PubMed Google Scholar * Wang, Y., Zhang, W.-Y. & You, S.-L. Ketones and aldehydes as O-Nucleophiles in iridium-catalyzed
intramolecular asymmetric allylic substitution reaction. _J. Am. Chem. Soc._ 141, 2228–2232 (2019). Article CAS PubMed Google Scholar * Zhai, H., Borzenko, A., Lau, Y. Y., Ahn, S. H.
& Schafer, L. L. Catalytic asymmetric synthesis of substituted morpholines and piperazines. _Angew. Chem., Int. Ed._ 51, 12219–12223 (2012). Article CAS Google Scholar * Luescher, M.
U. & Bode, J. W. Catalytic synthesis of _N_-unprotected piperazines, morpholines, and thiomorpholines from aldehydes and SnAP reagents. _Angew. Chem., Int. Ed._ 54, 10884–10888 (2015).
Article CAS Google Scholar * Numajiri, Y., Jiménez-Osés, G., Wang, B., Houk, K. N. & Stoltz, B. M. Enantioselective synthesis of dialkylated _N_-heterocycles by palladium-catalyzed
allylic alkylation. _Org. Lett._ 17, 1082–1085 (2015). Article CAS PubMed PubMed Central Google Scholar * Kuwano, R., Karube, D. & Ito, Y. Catalytic asymmetric hydrogenation of
1-aza-2-cycloalkene-2-carboxylates catalyzed by a _trans_-chelating chiral diphosphine PhTRAP-rhodium complex. _Tetrahedron Lett._ 40, 9045–9049 (1999). Article CAS Google Scholar * Li,
M. et al. Asymmetric hydrogenation for the synthesis of 2-substituted chiral morpholines. _Chem. Sci._ 12, 15061–15066 (2021). Article CAS PubMed PubMed Central Google Scholar * Lau, Y.
Y., Zhai, H. & Schafer, L. L. Catalytic asymmetric synthesis of morpholines. Using mechanistic insights to realize the enantioselective synthesis of piperazines. _J. Org. Chem._ 81,
8696–8709 (2016). Article CAS PubMed Google Scholar * Leathen, M. L., Rosen, B. R. & Wolfe, J. P. New strategy for the synthesis of substituted morpholines. _J. Org. Chem._ 74,
5107–5110 (2009). Article CAS PubMed PubMed Central Google Scholar * Palchykov, V. A. & Chebanov, V. A. Recent progress in the synthesis of morpholines. _Chem. Heterocycl. Compd._
55, 324–332 (2019). Article CAS Google Scholar * Siau, W.-Y. & Bode, J. W. One-step synthesis of saturated spirocyclic N-heterocycles with stannyl amine protocol (SnAP) reagents and
ketones. _J. Am. Chem. Soc._ 136, 17726–17729 (2014). Article CAS PubMed Google Scholar * Narczyk, A. & Stecko, S. An entry to non-racemic β-tertiary-β-amino alcohols, building
blocks for the synthesis of aziridine, piperazine, and morpholine scaffolds. _Org. Biomol. Chem._ 18, 5972–5981 (2020). The structural diversity of the substrates derived from natural amino
acids is extremely limited, especially for those bearing aza-quaternary stereocenters. Article CAS PubMed Google Scholar * Brands, K. M. J. et al. Efficient synthesis of NK1 receptor
antagonist aprepitant using a crystallization-induced diastereoselective transformation._J. Am. Chem. Soc._ 125, 2129–2135 (2003). Article CAS PubMed Google Scholar * Ruider, S. A.,
Müller, S. & Carreira, E. M. Ring expansion of 3-Oxetanone-derived spirocycles: facile synthesis of saturated nitrogen heterocycles. _Angew. Chem., Int. Ed._ 52, 11908–11911 (2013).
Article CAS Google Scholar * Strecker, A. Ueber die künstliche Bildung der Milchsäure und einen neuen, dem Glycocoll homologen Körper. _Ann. Chem. Pharm._ 75, 27–45 (1850). Article
Google Scholar * Ukaji, Y., Yamamoto, K., Fukui, M. & Fujisawa, T. Stereodivergent synthesis of optically active tertiary alcohols via addition reaction of Chiral 2-Acyl oxazolidine
with organometallics. _Tetrahedron Lett._ 32, 2919–2922 (1991). Article CAS Google Scholar * Agami, C., Couty, F. & Lequesne, C. _N_-Boc 2-Acyloxazolidines: useful precursors to
enantiopure 1,2-Diols via highly diastereoselective nucleophilic additions. _Tetrahedron_ 51, 4043–4056 (1995). Article CAS Google Scholar * Polyak, F., Dorofeeva, T., Zelchans, G. &
Shustov, G. Regio- and stereoselectivity of the formation of 1,3-Oxazolidines in the reaction of L-Ephedrine with phenylglyoxal. unexpected rearrangement of
2-Benzoyl-3,4-dimethyl-5-phenyl-l,3-oxazolidine to 4,5-Dimethyl-3,6- diphenylmorpholin-2-one. _Tetrahedron Lett._ 37, 8223–8226 (1996). Article CAS Google Scholar * Mlostón, G.,
Wróblewska, A., Linden, A. & Heimgartner, H. Studies on the reaction of Aryl Glyoxals with L-Prolinol: unexpected formation of Morpholin-2-one derivatives and stereoselective
trifluoromethylation of the bicyclic system. _Asian J. Org. Chem._ 4, 770–777 (2015). Article Google Scholar * Zhao, M. M. et al. Practical asymmetric synthesis of aprepitant, a potent
human NK-1 receptor antagonist, via a stereoselective Lewis acid catalyzed trans acetalization reaction. _J. Org. Chem._ 67, 6743–6747 (2002). Article CAS PubMed Google Scholar * Powell,
W. C. & Walczak, M. A. Asymmetric synthesis of chiral 1,2-Amino alcohols and Morpholin-2-ones from arylglyoxals. _J. Org. Chem._ 83, 10487–10500 (2018). Article CAS PubMed Google
Scholar * He, Y., Wu, H., Wang, Q. & Zhu, J. Catalytic enantioselective synthesis of morpholinones enabled by aza-benzilic ester rearrangement. _J. Am. Chem. Soc._ 143, 7320–7325
(2021). Article CAS PubMed Google Scholar * Wang, B. & Tu, Y.-Q. Stereoselective construction of quaternary carbon stereocenters via a semipinacol rearrangement strategy. _Acc. Chem.
Res._ 44, 1207–1222 (2011). Article CAS PubMed Google Scholar * Wu, H., Wang, Q. & Zhu, J. Recent advances in catalytic enantioselective rearrangement. _Eur. J. Org. Chem_. 2019,
1964–1980 (2019). * He, Y., Quan, R., Li, X., Zhu, J. & Wu, H. Asymmetric construction of α,α-disubstituted piperazinones enabled by benzilic amide rearrangement. _Angew. Chem., Int.
Ed._ 62, e202217954 (2023). Article CAS Google Scholar * Wu, H., Wang, Q. & Zhu, J. Catalytic enantioselective benzilic ester rearrangement. _Angew. Chem. Int. Ed._ 59, 7261–7265
(2020). Article CAS Google Scholar * Rubin, M. B. Chemistry of vicinal polyketones. _Chem. Rev._ 75, 177–202 (1975). Article CAS Google Scholar * Wasserman, H. H. & Han, W. T.
Vicinal Tricarbonyl products from singlet oxygen reactions.: application to the synthesis of carbacephams. _Tetrahedron Lett._ 25, 3743–3746 (1984). Article CAS Google Scholar *
Wasserman, H. H. & Han, W. T. A synthesis of antibiotic (±)-PS-5. _Tetrahedron Lett._ 25, 3747–3750 (1984). Article CAS Google Scholar * Wasserman, H. H. & Han, W. T. Penem
synthesis through C3-N ring closure of a β-lactam precursor. _J. Am. Chem. Soc._ 107, 1444–1446 (1985). Article CAS Google Scholar * Wasserman, H. H. et al. The chemistry of vicinal
tricarbonyls. A stable vinyl tricarbonyl hydrate as a di- and trielectrophile. _J. Am. Chem. Soc._ 111, 371–372 (1989). Article CAS Google Scholar * Wasserman, H. H. & Blum, C. A. The
chemistry of vicinal tricarbonyls. Use of vinyl and acetylenic derivatives in the synthesis of substituted indoles. _Tetrahedron Lett._ 35, 9787–9790. (1994). Article CAS Google Scholar
* Rubin, M. B. & Gleiter, R. The chemistry of vicinal polycarbonyl compounds. _Chem. Rev._ 100, 1121–1164 (2000). Article CAS PubMed Google Scholar * Wasserman, H. H. & Parr, J.
The chemistry of vicinal tricarbonyls and related systems. _Acc. Chem. Res._ 37, 687–701 (2004). Article CAS PubMed Google Scholar * Truong, P. M., Zavalij, P. Y. & Doyle, M. P.
Highly enantioselective carbonyl-ene reactions of 2,3-Diketoesters: efficient and atom-economical process to functionalized chiral α-Hydroxy-β-Ketoesters. _Angew. Chem. Int. Ed._ 53,
6468–6472 (2014). Article CAS Google Scholar * Wang, L., Zhong, J. & Lin, X. Atroposelective phosphoric acid catalyzed three-component cascade reaction: enantioselective synthesis of
axially chiral N-Arylindoles. _Angew. Chem. Int. Ed._ 58, 15824–15828 (2019). Article CAS Google Scholar * Chen, Z. et al. Organocatalytic enantioselective synthesis of axially chiral
_N,N’_-Bisindoles. _Angew. Chem. Int. Ed._ 62, e202300419 (2023). Article CAS Google Scholar * Akiyama, T., Itoh, J., Yokota, K. & Fuchibe, K. Enantioselective mannich-type reaction
catalyzed by a chiral brønsted acid. _Angew. Chem. Int. Ed._ 43, 1566–1568 (2004). Article CAS Google Scholar * Uraguchi, D. & Terada, M. Chiral brønsted acid-catalyzed direct mannich
reactions via electrophilic activation. _J. Am. Chem. Soc._ 126, 5356–5357 (2004). Article CAS PubMed Google Scholar * Wang, P. et al. Asymmetric 1,2-Perfluoroalkyl migration: easy
access to enantioenriched α-Hydroxy-α-perfluoroalkyl esters. _J. Am. Chem. Soc._ 137, 4626–4629 (2015). Article CAS PubMed Google Scholar * Liu, R. et al. Lewis-acid-catalyzed asymmetric
alkynylation of alkynyl 1,2-Diketones: controllable formation of 3(2_H_)‑furanones and α‑Hydroxy ketones. _Org. Lett._ 22, 6948–6953 (2020). Article CAS PubMed Google Scholar * Peng, B.
& Maulide, N. The redox-neutral approach to C-H functionalization. _Chem. Eur. J._ 19, 13274–13287 (2013). Article CAS PubMed Google Scholar * F. G. West, B. N. Naidu, Applications
of Stevens [1,2]-shifts of cyclic ammonium ylides. A Route to Morpholin-2-ones, J. Org. Chem. 1994, 59, 6051–6056. Download references ACKNOWLEDGEMENTS Financial support for this work was
provided by the National Natural Science Foundation of China (Grant No. 22101172, 22371183), and startup funding from Shanghai Jiao Tong University (SJTU). Dr. Y.-P. He thanks the China
Postdoctoral Science Foundation (grant no. 2022M710094). AUTHOR INFORMATION AUTHORS AND AFFILIATIONS * Shanghai Frontiers Science Center for Drug Target Identification and Delivery, and
Shanghai Key Laboratory for Molecular Engineering of Chiral Drugs, School of Pharmaceutical Sciences, Shanghai Jiao Tong University, 800 Dongchuan Road, Minhang District, Shanghai, 200240,
China Xing-Zi Li, Yu-Ping He & Hua Wu Authors * Xing-Zi Li View author publications You can also search for this author inPubMed Google Scholar * Yu-Ping He View author publications You
can also search for this author inPubMed Google Scholar * Hua Wu View author publications You can also search for this author inPubMed Google Scholar CONTRIBUTIONS X.L. Y.H. and H.W.
conceived the work, designed the experiments and analyzed the data. X.L. optimized the reaction conditions and performed the experiments. H.W. conceived and directed the project and wrote
the paper. All the authors discussed the results and commented on the manuscript. CORRESPONDING AUTHOR Correspondence to Hua Wu. ETHICS DECLARATIONS COMPETING INTERESTS The authors declare
no competing interests. PEER REVIEW PEER REVIEW INFORMATION _Communications Chemistry_ thanks the anonymous reviewers for their contribution to the peer review of this work. ADDITIONAL
INFORMATION PUBLISHER’S NOTE Springer Nature remains neutral with regard to jurisdictional claims in published maps and institutional affiliations. SUPPLEMENTARY INFORMATION SUPPLEMENTARY
INFORMATION DESCRIPTION OF ADDITIONAL SUPPLEMENTARY FILES SUPPLEMENTARY DATA 1 SUPPLEMENTARY DATA 2 SUPPLEMENTARY DATA 3 SUPPLEMENTARY DATA 4 SUPPLEMENTARY DATA 5 SUPPLEMENTARY DATA 6
SUPPLEMENTARY DATA 7 RIGHTS AND PERMISSIONS OPEN ACCESS This article is licensed under a Creative Commons Attribution 4.0 International License, which permits use, sharing, adaptation,
distribution and reproduction in any medium or format, as long as you give appropriate credit to the original author(s) and the source, provide a link to the Creative Commons licence, and
indicate if changes were made. The images or other third party material in this article are included in the article’s Creative Commons licence, unless indicated otherwise in a credit line to
the material. If material is not included in the article’s Creative Commons licence and your intended use is not permitted by statutory regulation or exceeds the permitted use, you will
need to obtain permission directly from the copyright holder. To view a copy of this licence, visit http://creativecommons.org/licenses/by/4.0/. Reprints and permissions ABOUT THIS ARTICLE
CITE THIS ARTICLE Li, XZ., He, YP. & Wu, H. Zinc chloride-catalyzed cyclizative 1,2-rearrangement enables facile access to morpholinones bearing aza-quaternary carbons. _Commun Chem_ 6,
216 (2023). https://doi.org/10.1038/s42004-023-01016-y Download citation * Received: 19 July 2023 * Accepted: 29 September 2023 * Published: 07 October 2023 * DOI:
https://doi.org/10.1038/s42004-023-01016-y SHARE THIS ARTICLE Anyone you share the following link with will be able to read this content: Get shareable link Sorry, a shareable link is not
currently available for this article. Copy to clipboard Provided by the Springer Nature SharedIt content-sharing initiative