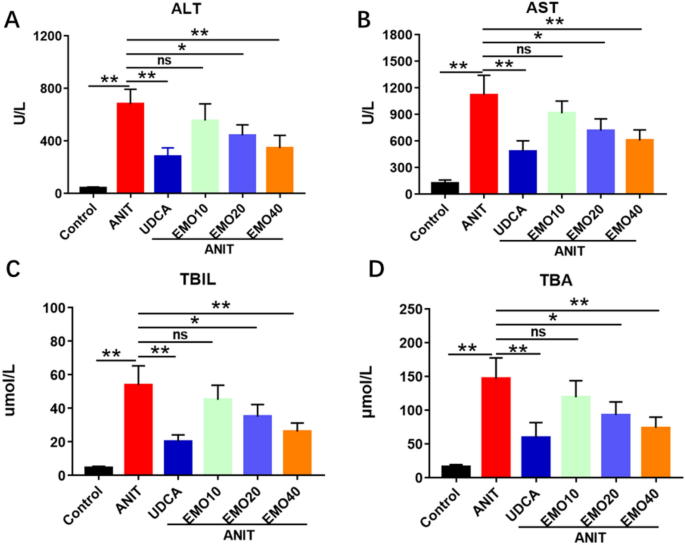
- Select a language for the TTS:
- UK English Female
- UK English Male
- US English Female
- US English Male
- Australian Female
- Australian Male
- Language selected: (auto detect) - EN
Play all audios:
ABSTRACT Emodin (EMO) has the effect of anti-cholestasis induced by alpha-naphthylisothiocyanate (ANIT). But its mechanism is still unclear. The farnesoid X receptor (Fxr) is the master bile
acid nuclear receptor. Recent studies have reported that Sirtuin 1 (Sirt1) can regulate the activities of Fxr. The purpose of the current study was to investigate the mechanism of EMO
against ANIT-induced liver injury based on Sirt1/Fxr signaling pathway. The ANIT-induced cholestatic rats were used with or without EMO treatment. Serum biochemical indicators, as well as
liver histopathological changes were examined. The genes expressions of _Sirt1, Fxr, Shp, Bsep and Mrp2_ were detected. The expressions of Sirt1, Fxr and their downstream related genes were
investigated in vitro. The results showed that EMO significantly alleviated ANIT-induced liver injury in rats, and increased _Sirt1, Fxr, Shp, Bsep and Mrp2_ gene expression in liver, while
decreased the expression of _Cyp7a1_. EMO significantly activated Fxr, while Sirt1 inhibitor and _Sirt1_ gene silencing significantly reduced Fxr activity in vitro. Collectively, EMO in the
right dose has a protective effect on liver injury induced by ANIT, and the mechanism may be through activation of Fxr by Sirt1, thus regulating bile acid metabolism, and reducing bile acid
load in hepatocytes. SIMILAR CONTENT BEING VIEWED BY OTHERS SODIUM AESCINATE-INDUCED HEPATOTOXICITY VIA ATF4/GSH/GPX4 AXIS-MEDIATED FERROPTOSIS Article Open access 07 January 2025
GINSENOSIDE RD PROTECTS AGAINST ACUTE LIVER INJURY BY REGULATING THE AUTOPHAGY NLRP3 INFLAMMASOME PATHWAY Article Open access 28 January 2025 ROSEOTOXIN B ALLEVIATES CHOLESTATIC LIVER
FIBROSIS THROUGH INHIBITING PDGF-B/PDGFR-Β PATHWAY IN HEPATIC STELLATE CELLS Article Open access 15 June 2020 INTRODUCTION In recent years, the incidence of cholestatic liver injury (CLI)
has increased, which is mainly characterized by metabolic disorders and excessive accumulation of intrahepatic bile acids (BAs)1. Without effective intervention, CLI can evolve into liver
fibrosis, cirrhosis and even lead to liver failure and death in severe cases. Meanwhile, CLI is a high risk factor for the occurrence of liver cancer and cholangiocarcinoma2. However,
Ursodesoxycholic acid (UDCA) and Obeticholic acid, the main FDA-approved drugs for the treatment of CLI, have limited efficacy, with more than one third of patients showing no response or
intolerance. There is still a lack of effective treatment in clinical practice3. Therefore, it is particularly important to further study the molecular mechanisms regulating BAs metabolism
and transport in search of new therapeutic strategies. BAs are endogenous substances that promote the emulsification and absorption of lipids. When metabolism is impaired, Accumulated BAs
can cause hepatocyte apoptosis and necrosis4. The abnormal expression and function of BAs metabolic enzymes and transporters may lead to BAs retention in the liver and eventually cause
cholestasis5. Previous studies have shown that the expression and function of BAs metabolic enzymes and transporters can be regulated by nuclear receptors (NRs)6,7. For example, farnesoid x
receptor (Fxr) plays important regulatory roles in repressing the cholesterol 7 α -hydroxylase (Cyp7a1), inhibiting the bile salt export pump (Bsep), and inducing the multi-drug
resistance-associated protein 2 (Mrp2)8,9. Therefore, Fxr is an important regulator of bile acid homeostasis10. Recent studies have reported that Sirtuin1 (Sirt1) can regulate the activities
of Fxr, indicating that Sirt1 is a transcription factor and plays an important role in BAs homeostasis11,12. Emodin (EMO) is a naturally active anthraquinone substance found in many
commonly used Chinese herbs, and is widely used as a traditional medicine in many countries, especially in Asia13. Emerging evidence shows that EMO possesses a wide spectrum of
pharmacological properties, including anticancer, hepatoprotective, antiinflammatory, antioxidant and antimicrobial activities14,15,16. EMO not only has the effect of anti-cholestasis
induced by alpha-naphthylisothiocyanate (ANIT), but also has been reported to cause liver injury, but its mechanism is still unclear17,18. Hence, we speculated that the therapeutic effect of
EMO on cholestasis may be related to its regulatory effect on Sirt1 to affect downstream NRs, consequently attenuating the imbalance of metabolism and transport of BAs. In the present
study, based on an assessment of the protective effect of EMO on ANIT-induced intrahepatic cholestasis, we systematically investigated the effect of EMO on BAs transporter expression and the
ability of this compound to reverse ANIT induced toxicity. This study demonstrates that EMO can improve cholestasis by activating Sirt1/Fxr signaling pathway to regulate BAs metabolism and
transport. RESULTS EMO ALLEVIATES ANIT-INDUCED CHOLESTATIC LIVER INJURY IN RATS As shown in Fig. 1, compared with the control group, the serum ALT, AST, TBA and TBIL levels of ANIT injured
rats were significantly increased. And compared with the ANIT group, the EMO (20 mg/kg, 40 mg/kg)-treated groups showed significant decreases in ALT, AST, TBA and TBIL levels. EMO (40 mg/kg)
induced similar effects as UDCA on ALT, AST and TBA. But there were no statistical difference between EMO (10 mg/kg) treated groups and ANIT group in the biochemical parameters (ALT, AST,
TBA and TBIL). We also found that when the dose of EMO reached 80 mg/kg, the liver injury induced by ANIT could not be alleviated, and the serum ALT, AST, TBA and TBIL in rats tended to
increase (supplement material 1). As shown in Fig. 2, We also observed that a certain dose of EMO mitigated liver damage caused by ANIT. In the control group, liver cells were arranged
radially along the central vein; the hepatocytes were uniform in size; the nuclear membrane was intact, and the nucleoli were clearly visible. In the ANIT group, the structure of hepatocytes
was obviously deformed; the boundary was unclear; the arrangement of hepatic cord was disordered; the nucleus was enlarged and the boundary was unclear. When the dose of EMO was 10 mg/kg,
the liver injury of rats did not alleviate. Compared with ANIT model group, liver cells in EMO (20 mg/kg, 40 mg/kg) group were arranged more regularly. And cell sizes were roughly equal;
edema was observably improved; inflammatory cell infiltration was reduced, and intrahepatic bile duct epithelial cell degeneration and necrosis were significantly reduced, and the extent of
the effect was dose-dependent. EMO DIRECTLY TARGETS WITH SIRT1 In order to predict the drug-target of EMO against ANIT-induced CLI, molecular docking assay was used. By molecular docking,
the binding energy of EMO towards to SIRT1 was −9.70 kcal/mol. Moreover, hydrogen bonding, hydrophobic effect and electrostatic interaction analysis were executed to clarify the dynamics
behind the strong binding affinity. Figure 3A showed the polyhydroxyl form hydrogen bond network with the amino acid residues in the binding pocket. The amino acid residues involved in the
formation of hydrogen bonds include Asparagine-156 (ASP-156) and arginine-158 (ARG-158). The formation of hydrophobic cavity by hydrophobic molecules fused ring and binding pocket included
Phenylalanine-157 (PHE-157), Leucine-195 (LEU-195) and Leucine-199 (LEU-199), which further enhanced the combination of EMO and SIRT1. EFFECT OF EMO ON BILE ACID HOMEOSTASIS RELATED GENE
EXPRESSION IN RAT LIVER To verify the protective effects of EMO in ANIT-induced cholestasis, this study measured the gene expression of Fxr and transcription factors associated with BAs
homeostasis in rat liver. As shown in Fig. 3B–G, compared with the control group, ANIT treatment significantly decreased _Sirt1_ (_P_ < 0.05), _Fxr_ (_P_ < 0.01), _Shp_ (_P_ <
0.05), _Bsep_ (_P_ < 0.01) and _Mrp2_ (_P_ < 0.05) mRNA levels and increased _Cyp7a1_ (_P_ < 0.01) mRNA level. As shown in Fig. 4, similarly, compared with the control group, the
protein expression levels of the above genes also showed the same trend under ANIT treatment. Compared with the ANIT group, protein levels of SIRT1, FXR, BSEP and MRP2 were significantly
increased in EMO treatment, while those of CYP7A1 were significantly decreased. This finding suggests that the protective effect of EMO against cholestasis may be the result of reduced BAs
synthesis and increased BAs efflux, which is possibly mediated via Fxr or Sirt1 activation. EMO ACTIVATED FXR THROUGH SIRT1 IN HEPG2 CELLS As Fxr pathway plays an important role in
regulating BAs homeostasis, we investigated whether EMO exerted protective effects against cholestasis through modulating Fxr activities. Interestingly, as shown in Fig. 5A, SRT1720 (a
selective activator of Sirt1) and EX-527 (a potent and selective Sirt1 inhibitor) can significantly increase and inhibit _Fxr_ mRNA expression, respectively. EMO can increase _Fxr_ and _Shp_
mRNA expression in a dose-dependent manner (Fig. 5A, B). Similarly, EMO dose-dependent increases the protein expression of FXR and SHP (Fig. 5C). As shown in Fig. 5D, E, EMO significantly
increased _Sirt1_ and _Fxr_ mRNA levels, but Fxr selective activator (GW4064) and selective inhibitor (Z-Guggulsterone, GU) did not significantly affect _Sirt1_ mRNA levels in HepG2 cells.
Similarly, EMO significantly increased the protein expression of SIRT1 and FXR. But GW4064 and GU did not significantly affect the protein expression of SIRT1 in HepG2 cells. Compared with
EMO group, there was no significant difference in SIRT1 protein expression after GW or GU combined with EMO intervention, while FXR protein expression changed significantly (Fig. 5F). The
results suggest that EMO may affect the expression of _Fxr_ mRNA and protein by regulating Sirt1. EMO REGULATED FXR ACTIVITY AND FXR TARGET GENE EXPRESSION VIA SIRT1 IN HEPG2 CELLS As shown
in Fig. 6A, B, compared with the empty plasmid (NC) group, _Sirt1_ mRNA expression was significantly down-regulated after si-Sirt1 transfection in HepG2 cells (_P_ < 0.01), and the mRNA
expressions of _Fxr, Shp, Bsep and Mrp2_ were significantly decreased, while the mRNA expression of _Cyp7a1_ was increased (_P_ < 0.01). EMO (0.2 or 0.8 μM) could dose-dependently affect
the expressions of _Sirt1, Fxr, Shp, Bsep, Mrp2_, and _Cyp7a1_ mRNA in HepG2 cells transfected with empty plasmid (_P_ < 0.01). Silencing Sirt1 significantly weakened the effect of EMO
(0.2 or 0.8 μM) on _Sirt1, Fxr, Shp, Bsep, Mrp2_ and _Cyp7a1_ mRNA. The results showed that by silencing Sirt1 gene, the protein expressions of SIRT1, FXR, SHP, BSEP and MRP2 in HepG2 cells
were decreased, while the protein expressions of CYP7A1 were increased, and these changes were statistically significant (Fig. 6C). EMO ALLEVIATES ANIT-INDUCED PRIMARY HEPATOCYTE INJURY
THROUGH MODULATION OF SIRT1/FXR SIGNALING PATHWAY IN RAT PRIMARY HEPATOCYTE As illustrated in Fig. 7A–C, compared with ANIT group, EMO improved cell survival rate and decreased ALT and AST,
indicating that EMO markedly alleviated the damage of liver cells induced by ANIT. The intervention of Sirt1 specific antagonist EX-527 attenuates the protection of EMO against ANIT-damaged
hepatocytes. Next, we evaluated the protective effect of EMO against EX-527 by transporters and Fxr in rat primary hepatocyte. EMO can significantly increase the mRNA and protein expression
levels of Sirt1 and Fxr, which are observably reduced by EX-527. Then, we examined the mRNA and protein levels of downstream _Fxr_ genes, such as _Shp, Bsep, Mrp2 and Cyp7a1_. EMO
significantly increased the expression of _Shp, Bsep_ and _Mrp2_ mRNA, and decreased the expression of _Cyp7a1_ mRNA. The above effects of EMO were inhibited when EX-527 was administered
(Fig. 7D–I). Similar changes can be observed by measuring the corresponding protein expression in cells (Fig. 7J). DISCUSSION EMO alleviates ANIT toxicity in a dose-dependent manner (10–40
mg/kg). However, at a dose of 80, EMO did not reduce liver damage caused by ANIT at all. Instead, the damage could be even more serious (supplement material 1). This result is similar to the
study by Wang18, who describes that EMO mitigated CLI induced by ANIT in mice, whereas two EMO-containing herbs, Polygonum multiflorum and cassia seed, significantly aggravated intrahepatic
cholestasis in mice treated with ANIT. These results suggest that the effect of EMO is closely related to dose. Previous studies19,20 have shown that EMO can alleviate pulmonary fibrosis
and lung injury in mice by regulating Sirt1. However, whether the effect of EMO in alleviating ANIT-induced cholestatic liver injury is related to the regulation of Sirt1 is unclear. Our
molecular docking results show that EMO has a strong interaction with Sirt1, which may play a potential role. This study showed that EMO had a protective effect on ANIT-induced liver injury
in rats by stimulating the expression of Sirt1 and Fxr. The intervention of Sirt1 selective inhibitor EX-527 attenuates the protective effect of EMO on hepatocytes. Next, we evaluated the
protective effect of EMO against EX-527 by transporters and Fxr in rat primary hepatocyte. EMO can markedly increase the mRNA and protein levels of Sirt1 and Fxr, which are observably
reduced by EX-527. Then, we examined the mRNA and protein levels of downstream _Fxr_ genes, such as _Shp, Bsep, Mrp2 and Cyp7a1_ in rat primary hepatocyte. And the results showed that EMO
significantly increased the expression of Shp, Bsep and Mrp2 genes, while decreased the mRNA and protein levels of Cyp7a1. When EX-527 was used, the above effects of EMO were suppressed.
ANIT is a hepatotoxic compound widely used to induce intrahepatic cholestasis in rodents. It causes cholestasis by impairs the polarization of hepatic parenchymal cells, directly damages
bile duct epithelial cells, and inhibits the function and expression of BAs transporters21. It has been reported that intragastric administration of ANIT in rats can significantly reduce
bile flow and cause bile acid metabolism disorder22. Our previous studies have shown that ANIT can cause changes in the expression of genes related to bile acid metabolic transport in rat
liver, resulting in impaired bile acid metabolic transport7. In this study, we investigated the protective effect of EMO against ANIT-induced liver injury in rats, and investigated the
expression changes of related genes in the liver of rats in each group. The results showed that, compared with the ANIT-treated group, EMO significantly reduced the liver injury induced by
ANIT, and markedly increased the expression levels of Fxr and Sirt1 genes. However, ANIT-induced changes in BAs-related NRs have been inconsistent in different studies21,23,24,25. The
diversity of this effect may be caused by different experimental conditions and complex regulation of NRs, namely, negative feedback of BAs balance, stress response of the body, cross
regulation of BA in vivo. In order to further confirm the influence of EMO on Fxr pathway, we detected the expression of Fxr and Fxr target genes _Shp_ in HepG2 cell experiments (Fig. 5).
EMO increased _Fxr_ and _Shp_ mRNA expression in a dose-dependent manner (Fig. 5A, B). EMO significantly increased _Sirt1_ mRNA levels, but Fxr activator (GW4064) and inhibitor (GU) did not
significantly affect _Sirt1_ mRNA levels in HepG2 cells. Compared with EMO alone intervention group, the levels of Sirt1 mRNA of the group in which EMO combined with GW4064 or GU
intervention showed no significant difference (Fig. 5D). However, GW4064 significantly increased _Fxr_ mRNA level and GU significantly inhibited _Fxr_ mRNA expression in the presence or
absence of EMO (Fig. 5E). Similar changes also appeared in the results of cell protein expression measurements (Fig. 5C, F). Selective silencing of SIRT1 gene in HepG2 cells by siRNA can
weaken the upregulation effect of EMO on SIRT and FXR. While silencing SIRT gene, the expression of FXR and its downstream genes SHP, BSEP and MRP2 is reduced, and the expression of CYP7A1
is upregulated (Fig. 6). The results suggest that EMO may affect the expression of _Fxr_ by regulating _Sirt1_ expression. Sirt1 is an NAD-dependent histone deacetylase that plays a key role
in fat, glucose and BA metabolism. Sirt1 and Fxr can form an interacting regulatory network: acetylation stabilizes _Fxr_, but reduces its activity by reducing Fxr/Rxr heterodimerization26.
So as to analyze the effect of EMO on Sirt1/Fxr signaling pathway, we further investigated the primary rat hepatocytes. We quantitatively detected the expression levels of _Sirt1_ and _Fxr_
target genes in primary rat hepatocytes. The results showed that EMO increased gene expression levels of Sirt1, Fxr, Shp, Mrp2, and Bsep, while EX-527 intervention significantly inhibited
these changes (Fig. 7). In summary, the present study suggests that EMO can alleviate liver injury induced by ANIT at a certain dose, and the mechanism may be that emodin can activate Fxr
through Sirt1, thus reducing the expression of bile acid synthesis rate-limiting enzyme, promoting the expression of efflux transporter gene, and alleviating bile acid load in hepatocytes.
MATERIALS AND METHODS CHEMICALS AND REAGENTS EMO (purity ≥ 97%), UDCA (purity ≥ 99%), SRT1720 (purity ≥ 98%, Sirt1 selective activator), EX-527 (purity ≥ 98%, Sirt1 selective inhibitor),
GW4064 (purity ≥ 98%, Fxr selective activator), Z-Guggulsterone (GU, purity ≥ 98%, Fxr selective inhibitor) and ANIT (purity ≥ 98%) were purchased from Macklin Biochemical (Shanghai, China).
Alanine aminotransferase (ALT), aspartate aminotransferase (AST), total bilirubin (TBIL) and total bile acid (TBA) assay kits were obtained from Nanjing Jiancheng Biotechnology (Nanjing,
China). Antibodies for SIRT1, SHP (NR0B2), FXR, BSEP, MRP2, CYP7A1 and GAPDH were obtained from Affinity biosciences (Cincinnati, OH, USA). Antibodies for Horseradish peroxidase-labeled goat
anti-rabbit IgG were obtained from Proteintech Group Inc. (Chicago, IL, USA). All the other chemicals of analytical grade were purchased from commercial sources. ETHICAL APPROVAL This
investigation conforms to the Guide for the Care and Use of Laboratory Animals published by the US National Institutes of Health (NIH Publication, revised 1985). All animal experiments were
approved by Ethics Committee of The First Affiliated Hospital of Nanchang University (registration number: CDYFY-IACUC-202304QR046, date: 26-04-2023). The usage of samples and all methods
were in strict accordance with the ARRIVE guidelines. ANIMALS AND TREATMENTS Male Sprague–Dawley (SD) rats (200 ± 10 g) were obtained from Hunan SJA Laboratory Animal Co., Ltd (Changsha,
Hunan, China, No. SYXK < Xiang > 2019–0017). They were housed under conditions of 55% humidity at 20–25 °C, provided a standard diet with water available ad libitum and were kept on a
12 h light/dark cycle. The rats were randomly divided into the following 6 groups (n = 6): Control group, ANIT group, ANIT combined with UDCA (60 mg/kg) group and ANIT combined with EMO (10,
20, 40 mg/kg) group. Vehicle (0.2% CMC-NA), UDCA (60 mg/kg) or EMO (10, 20, 40 mg/kg) alone were treated to rats by oral gavage once a day for 7 days13. Rats in the control group were
received olive oil and rats in other groups were given ANIT (75 mg/kg, dissolved in olive oil) intragastrically on day 57. After fasting for 12 h, all rats were sacrificed (pentobarbital
sodium, 65 mg/kg, intraperitoneal injection) on day 7 to collect serum and liver tissue for further study. BIOCHEMICAL INDEXES AND HISTOLOGICAL ANALYSIS The levels of ALT, AST, TBIL and TBA
were determined using commercial kits according to the manufacturer’s instructions. After rats were sacrificed, livers were collected, fixed in 4% formaldehyde, embedded in paraffin,
sectioned at 5 µm, and stained with hematoxylin and eosin (H&E). Images were observed by Olympus BX43 light microscope (Tokyo, Japan). MOLECULAR DOCKING The Standard Delay Format
structure profile of EMO was retrieved from the PubChem database (https://pubchem.ncbi.nlm.nih.gov/) and converted into a Program Data Base profile by Open Babel version 2.3.2 software
(http://openbabel.org/). The receptor protein for SIRT1 was then obtained from the Protein Data Bank database (http://www.rcsb.org/pdb/). PYMOL version 2.1.0 software (https://pymol.org/2/)
was then used to remove water molecules and isolate proteins, and AutoDock Tools version 1.5.6 software (https://www.cgl.ucsf.edu/) was used to add non-polar hydrogen and balance the
charges. To find the best ligand conformer with the least binding energy, three dimensional structures of EMO were built and optimized. HEPG2 CELLS CULTURE AND TREATMENTS HepG2 cells were
purchased from Procell Life Science Co., Ltd. (Wuhan, Hubei, China). The cells were cultured in Dulbecco’s modified Eagle medium (DMEM) high glucose (Hyclone, Logan, UT, USA) at 37℃ and 5%
CO2 humidified atmosphere supplemented with 10% fetal bovine serum (Biological Industries, Beit Haemek, Israel). The Cells were seeded onto six-well plates at a density of 5 × 105 cells per
well, and 24 h later, SRT1720 (5 μM), EX-527 (5 μM), GW4064 (2 μM) or GU (2 μM) was added to the wells for 24 h. Then, the cells were treated with EMO (0.2, 0.6, 0.8 μM) or 0.1% dimethyl
sulfoxide (DMSO) for 24 h. For the RNA silencing experiment, the cells were transfected with siRNA targeting Sirt1, or control siRNA using commercially available kits (Guangzhou RiboBio,
Guangzhou, China). the survival rate of cells was determined by CCK8 (Dojindo Laboratories, Japan)(supplementary materials 2). RAT PRIMARY HEPATOCYTES ISOLATION, CULTURE AND TREATMENTS Rat
primary hepatocytes were prepared from male SD rats and seeded in collagen-coated plates as previously described7. The cells were cultured in serum-free Williams’ E medium. EX-527 (5 μM) or
EX-527 (5 μM) + EMO (0.6 μM) were added to the wells for 24 h. Then, the cells were treated with ANIT (50 μM) or 0.1% DMSO for 24 h. the survival rate of cells was determined by CCK8
(Dojindo Laboratories, Japan) (supplementary materials 2). RT-QPCR AND WESTERN BLOT ANALYSIS Total RNA from liver and intestine was extracted using TRIzol Reagent (Invitrogen, CA, United
States) according to the manufacturer’s instructions. Total RNA was reverse transcribed into cDNA using PrimeScriptTM RT Master Mix (Takara, Dalian, China), and then obtained cDNA was used
as a template for real-time PCR amplification, performed by using SYBR Green (Takara, Dalian, China) and forward/reverse primer pairs for the tested genes. Independent reactions were
performed in triplicate using an ABI StepOne Plus system (Applied Biosystems, CA, United States)12. Both forward and reverse primers used are presented in Table 1. The total proteins were
collected by lysis with RIPA lysis buffer. The nuclear proteins were prepared with nuclear and cytoplasmic protein preparation kit (APPLYGEN, Beijing, China). The total proteins were
resolved on 10% SDS-PAGE gel and transferred to nitrocellulose membranes. Prior to hybridisation with antibodies, some blots were cut, precluding the provision of original images of the
full-length blots. The immunoreactive bands were detected using horseradish peroxidase-conjugated secondary antibodies and ECL reagents7. Specific protein bands were detected using enhanced
chemiluminescence (ECL) kit (US EVERBRIGHT, Suzhou, China). STATISTICAL ANALYSIS The data were analyzed by the Statistical Package for Social Sciences (SPSS) version 25.0 (Chicago, USA). The
graphs were generated using the GraphPad Prism software (version 8.0) (San Diego, USA). All data were presented as the mean ± standard deviation (SD). The differences among the experimental
groups were calculated by ANOVA, whereas student’s t-test was used to detect significant differences between two groups. _p_ < 0.05 was considered statistically significant, and _p_ <
0.01 was considered highly significant. DATA AVAILABILITY The datasets generated during the present study are available from the corresponding author on reasonable request. ABBREVIATIONS *
ALT: Alanine aminotransferase * ANIT: Alpha-naphthylisothiocyanate * AST: Aspartate aminotransferase * BAs: Bile acids * Bsep: Bile salt export pump * CLI: Cholestatic liver injury * Cyp7a1:
Cholesterol 7 α-hydroxylase * DMSO: Dimethyl sulfoxide * ECL: Enhanced chemiluminescence * EMO: Emodin; FBS, petal bovine serum * Fxr: Farnesoid x receptor * GU: Z-Guggulsterone * H&E:
Hematoxylin and eosin * Mrp2: Multi-drug resistance-associated protein 2 * NRs: Nuclear receptors * TBA: Total bile acid * TBIL: Total bilirubin * UDCA: Ursodesoxycholic acid REFERENCES *
Sarin, S. K. _et al._ Liver diseases in the Asia-Pacific region: A lancet gastroenterology and hepatology commission. _Lancet Gastroenterol. Hepatol._ 5(2), 167–228.
https://doi.org/10.1016/S2468-1253(19)30342-5 (2020). Article PubMed Google Scholar * Floreani, A. & Mangini, C. Primary biliary cholangitis: Old and novel therapy. _Eur. J. Intern.
Med._ 47, 1–5. https://doi.org/10.1016/j.ejim.2017.06.020 (2018). Article CAS PubMed Google Scholar * Kummen, M. & Hov, J. R. The gut microbial influence on cholestatic liver
disease. _Liver Int._ 39(7), 1186–1196. https://doi.org/10.1111/liv.14153 (2019). Article PubMed Google Scholar * Wu, J. S. _et al._ Huangqi decoction alleviates
alpha-naphthylisothiocyanate induced intrahepatic cholestasis by reversing disordered bile acid and glutathione homeostasis in mice. _Front. Pharmacol._ 8, 938.
https://doi.org/10.3389/fphar.2017.00938 (2017). Article CAS PubMed PubMed Central Google Scholar * Xu, L. _et al._ Analyzing the hepatoprotective effect of the Swertia cincta
Burkillextract against ANIT-induced cholestasis in rats by modulating the expression of transporters and metabolic enzymes. _J. Ethnopharmacol._ 209, 91–99.
https://doi.org/10.1016/j.jep.2017.07.031 (2017). Article PubMed Google Scholar * Jia, W., Xie, G. & Jia, W. Bile acid-microbiota crosstalk in gastrointestinal inflammation and
carcinogenesis. _Nat. Rev. Gastroenterol. Hepatol._ 15(2), 111–128. https://doi.org/10.1038/nrgastro.2017.119 (2018). Article CAS PubMed Google Scholar * Liu, J. _et al._ Oleanolic acid
alleviates ANIT-induced cholestatic liver injury by activating Fxr and Nrf2 pathways to ameliorate disordered bile acids homeostasis. _Phytomedicine_ 102, 154173.
https://doi.org/10.1016/j.phymed.2022.154173 (2022). Article CAS PubMed Google Scholar * Kong, L. _et al._ Yangonin modulates lipid homeostasis, ameliorates cholestasis and cellular
senescence in alcoholic liver disease via activating nuclear receptor FXR. _Phytomedicine_ 90, 153629. https://doi.org/10.1016/j.phymed.2021.153629 (2021). Article CAS PubMed Google
Scholar * Molinaro, A. & Marschall, H. U. Bile acid metabolism and FXR-mediated effects in human cholestatic liver disorders. _Biochem. Soc. Trans._ 50(1), 361–373.
https://doi.org/10.1042/BST20210658 (2022). Article CAS PubMed Google Scholar * Keitel, V., Droge, C. & Haussinger, D. Targeting FXR in cholestasis. _Handb. Exp. Pharmacol._ 256,
299–324. https://doi.org/10.1007/164_2019_231 (2019). Article CAS PubMed Google Scholar * Garcia-Rodriguez, J. L. _et al._ SIRT1 controls liver regeneration by regulating bile acid
metabolism through farnesoid X receptor and mammalian target of rapamycin signaling. _Hepatology_ 59(5), 1972–1983. https://doi.org/10.1002/hep.26971 (2014). Article CAS PubMed Google
Scholar * Wu, S. Y. _et al._ 18beta-glycyrrhetinic acid protects against alpha-naphthylisothiocyanate-induced cholestasis through activation of the Sirt1/FXR signaling pathway. _Acta
Pharmacol. Sin._ 39(12), 1865–1873. https://doi.org/10.1038/s41401-018-0110-y (2018). Article CAS PubMed PubMed Central Google Scholar * Hu, N., Liu, J., Xue, X. & Li, Y. The effect
of emodin on liver disease—comprehensive advances in molecular mechanisms. _Eur. J. Pharmacol._ 882, 173269. https://doi.org/10.1016/j.ejphar.2020.173269 (2020). Article CAS PubMed
Google Scholar * Dong, X. _et al._ Emodin: A review of its pharmacology, toxicity and pharmacokinetics. _Phytother. Res._ 30(8), 1207–1218. https://doi.org/10.1002/ptr.5631 (2016). Article
CAS PubMed PubMed Central Google Scholar * Shen, P., Han, L., Chen, G., Cheng, Z. & Liu, Q. Emodin attenuates acetaminophen-induced hepatotoxicity via the cGAS-STING pathway.
_Inflammation_ 45(1), 74–87. https://doi.org/10.1007/s10753-021-01529-5 (2022). Article CAS PubMed Google Scholar * Fang, L. _et al._ Anticancer activity of Emodin is associated with
downregulation of CD155. _Int. Immunopharmacol._ 75, 105763. https://doi.org/10.1016/j.intimp.2019.105763 (2019). Article CAS PubMed PubMed Central Google Scholar * Xia, Y. _et al._
Mechanism and active components of Qingre Lidan tablets alleviate intrahepatic cholestasis by activating the Farnesoid X receptor. _Evid. Based Complement. Alternat. Med._ 2022, 1589388.
https://doi.org/10.1155/2022/1589388 (2022). Article PubMed PubMed Central Google Scholar * Wang, X. _et al._ Paradoxical effects of Emodin on ANIT-induced intrahepatic cholestasis and
herb-induced hepatotoxicity in mice. _Toxicol. Sci._ 168(1), 264–278. https://doi.org/10.1093/toxsci/kfy295 (2019). Article CAS PubMed Google Scholar * Yang, T. _et al._ Emodin
suppresses silica-induced lung fibrosis by promoting Sirt1 signaling via direct contact. _Mol. Med. Rep._ 14(5), 4643–4649. https://doi.org/10.3892/mmr.2016.5838 (2016). Article CAS PubMed
PubMed Central Google Scholar * Liu, F., Gu, T. & Wei, D. Emodin alleviates sepsis-mediated lung injury via inhibition and reduction of NF-kB and HMGB1 pathways mediated by SIRT1.
_Kaohsiung J. Med. Sci._ 38(3), 253–260. https://doi.org/10.1002/kjm2.12476 (2022). Article CAS PubMed Google Scholar * Yang, T. _et al._ Early indications of ANIT-induced cholestatic
liver injury: Alteration of hepatocyte polarization and bile acid homeostasis. _Food Chem. Toxicol._ 110, 1–12. https://doi.org/10.1016/j.fct.2017.09.051 (2017). Article ADS CAS PubMed
Google Scholar * Meng, F. _et al._ Dolomiaea souliei ethyl acetate extract protected against alpha-naphthylisothiocyanate-induced acute intrahepatic cholestasis through regulation of
farnesoid x receptor-mediated bile acid metabolism. _Phytomedicine_ 87, 153588. https://doi.org/10.1016/j.phymed.2021.153588 (2021). Article CAS PubMed Google Scholar * Yan, J. _et al._
Herbal medicine Yinchenhaotang protects against alpha-naphthylisothiocyanate-induced cholestasis in rats. _Sci. Rep._ 7(1), 4211. https://doi.org/10.1038/s41598-017-04536-5 (2017). Article
ADS CAS PubMed PubMed Central Google Scholar * Ma, X. _et al._ Paeonia lactiflora Pall protects against ANIT-induced cholestasis by activating Nrf2 via PI3K/Akt signaling pathway. _Drug
Des. Dev. Ther._ 9, 5061–5074. https://doi.org/10.2147/DDDT.S90030 (2015). Article CAS Google Scholar * Shi, M., Tang, J., Zhang, T. & Han, H. Swertiamarin, an active iridoid
glycoside from Swertia pseudochinensis H.Hara, protects against alpha-naphthylisothiocyanate-induced cholestasis by activating the farnesoid X receptor and bile acid excretion pathway. _J.
Ethnopharmacol._ 291, 115164 (2022). Article CAS PubMed Google Scholar * Kulkarni, S. R., Soroka, C. J., Hagey, L. R. & Boyer, J. L. Sirtuin 1 activation alleviates cholestatic liver
injury in a cholic acid-fed mouse model of cholestasis. _Hepatology_ 64(6), 2151–2164. https://doi.org/10.1002/hep.28826 (2016). Article CAS PubMed Google Scholar Download references
ACKNOWLEDGEMENTS This study was supported by National Natural Science Foundation of China (No. 82360735), Natural Science Foundation of Jiangxi Province (No. 20232BAB206137), Science and
technology project of Jiangxi Provincial Administration of Traditional Chinese Medicine(No. 2023B1340) and The First Affiliated Hospital of Nanchang University youth talent training Fund
(No. YFYPY202275). AUTHOR INFORMATION AUTHORS AND AFFILIATIONS * Department of Pharmacy, The First Affiliated Hospital of Nanchang University, Nanchang, 330006, People’s Republic of China
Zhi Hu, Xiaohua Cheng, Jun Cai, Jinfang Hu & Jianming Liu * School of Pharmacy, Nanchang University, Nanchang, 330031, People’s Republic of China Chao Huang Authors * Zhi Hu View author
publications You can also search for this author inPubMed Google Scholar * Xiaohua Cheng View author publications You can also search for this author inPubMed Google Scholar * Jun Cai View
author publications You can also search for this author inPubMed Google Scholar * Chao Huang View author publications You can also search for this author inPubMed Google Scholar * Jinfang Hu
View author publications You can also search for this author inPubMed Google Scholar * Jianming Liu View author publications You can also search for this author inPubMed Google Scholar
CONTRIBUTIONS Zhi Hu: Validation and writing-original draft. Xiaohua Cheng and Jun Cai: Data curation. Chao Huang and Jinfang Hu: Formal analysis and investigation. Jianming Liu:
Writing-original draft, funding acquisition, conceptualization and supervision. All authors reviewed the manuscript. CORRESPONDING AUTHORS Correspondence to Jinfang Hu or Jianming Liu.
ETHICS DECLARATIONS COMPETING INTERESTS The authors declare no competing interests. ADDITIONAL INFORMATION PUBLISHER'S NOTE Springer Nature remains neutral with regard to jurisdictional
claims in published maps and institutional affiliations. SUPPLEMENTARY INFORMATION SUPPLEMENTARY FIGURE 1. SUPPLEMENTARY FIGURE 2. SUPPLEMENTARY FIGURE 3. SUPPLEMENTARY FIGURE 4.
SUPPLEMENTARY FIGURE 5. SUPPLEMENTARY FIGURE 6. SUPPLEMENTARY FIGURE 7. RIGHTS AND PERMISSIONS OPEN ACCESS This article is licensed under a Creative Commons
Attribution-NonCommercial-NoDerivatives 4.0 International License, which permits any non-commercial use, sharing, distribution and reproduction in any medium or format, as long as you give
appropriate credit to the original author(s) and the source, provide a link to the Creative Commons licence, and indicate if you modified the licensed material. You do not have permission
under this licence to share adapted material derived from this article or parts of it. The images or other third party material in this article are included in the article’s Creative Commons
licence, unless indicated otherwise in a credit line to the material. If material is not included in the article’s Creative Commons licence and your intended use is not permitted by
statutory regulation or exceeds the permitted use, you will need to obtain permission directly from the copyright holder. To view a copy of this licence, visit
http://creativecommons.org/licenses/by-nc-nd/4.0/. Reprints and permissions ABOUT THIS ARTICLE CITE THIS ARTICLE Hu, Z., Cheng, X., Cai, J. _et al._ Emodin alleviates cholestatic liver
injury by modulating Sirt1/Fxr signaling pathways. _Sci Rep_ 14, 16756 (2024). https://doi.org/10.1038/s41598-024-67882-1 Download citation * Received: 31 March 2024 * Accepted: 17 July 2024
* Published: 20 July 2024 * DOI: https://doi.org/10.1038/s41598-024-67882-1 SHARE THIS ARTICLE Anyone you share the following link with will be able to read this content: Get shareable link
Sorry, a shareable link is not currently available for this article. Copy to clipboard Provided by the Springer Nature SharedIt content-sharing initiative KEYWORDS * Emodin * Cholestatic
liver injury * Sirtuin1 (Sirt1) * Farnesoid x receptor (Fxr) * Bile acid metabolism