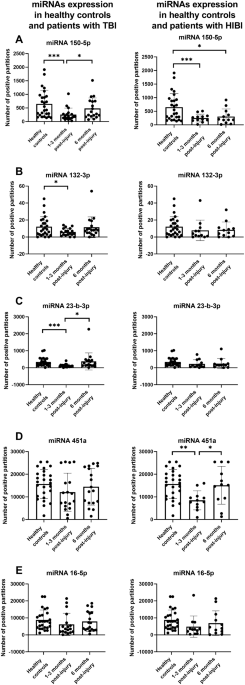
- Select a language for the TTS:
- UK English Female
- UK English Male
- US English Female
- US English Male
- Australian Female
- Australian Male
- Language selected: (auto detect) - EN
Play all audios:
ABSTRACT MicroRNAs (miRNAs) are involved in gene regulation and may affect secondary brain injury and recovery in patients with disorders of consciousness (DoC). This study investigated the
role of five miRNAs (150-5p, 132-3p, 23b-3p, 451a, and 16-5p) in prolonged DoC. miRNA levels were assessed in serum samples from 30 patients with unresponsive wakefulness syndrome or
minimally conscious state due to traumatic or hypoxic-ischemic brain injury (TBI, HIBI) at baseline (1–3 months) and 6 months post-injury. Patients’ diagnoses were determined using the Coma
Recovery Scale revised, and functional outcomes were evaluated 6 months after injury with the Glasgow Outcome Scale Extended (GOSE) and the Functional Independence Measure (FIM). Compared to
healthy controls, patients with TBI had lower levels of miRNAs 150-5p, 132-3p, and 23b-3p at baseline, while patients with HIBI had lower levels of miRNA 150-5p at baseline and 6 months
post-injury and a reduction of miRNA 451a at baseline. Higher levels of miRNAs 132-3p and 23b-3p were associated with better outcomes in TBI patients as indicated by GOSE and FIM scores.
This study highlights distinct miRNA dysregulated patterns in patients with prolonged DoC, dependent on etiology and post-injury time, and suggests that miRNAs 132-3p and 23b-3p may serve as
prognostic biomarkers. SIMILAR CONTENT BEING VIEWED BY OTHERS TEMPORAL BRAIN MICRORNA EXPRESSION CHANGES IN A MOUSE MODEL OF NEONATAL HYPOXIC–ISCHEMIC INJURY Article 31 August 2021
MICRORNAS AS BIOMARKERS OF BRAIN INJURY IN NEONATAL ENCEPHALOPATHY: AN OBSERVATIONAL COHORT STUDY Article Open access 19 March 2024 MICRORNA THERAPEUTIC TARGETS IN NEONATAL HYPOXIC–ISCHEMIC
BRAIN INJURY: A NARRATIVE REVIEW Article 19 July 2022 INTRODUCTION The vegetative state, also known as unresponsive wakefulness syndrome (UWS)1, is the worst possible clinical evolution in
comatose survivors of severe brain injuries. Patients with UWS exhibit no behavior indicative of self-awareness or awareness of the external world and they can remain unconscious
indefinitely or progress to a minimally conscious state (MCS), which is characterized by the fluctuating reappearance of minimal signs of awareness2. When UWS or MCS persist for more than 28
days, they are defined as prolonged disorders of consciousness (DoC)3. Severe brain injuries, such as traumatic brain injuries (TBI) and hypoxic-ischemic brain injuries (HIBI), can cause
long-term structural and functional changes in the brain that may contribute to both neurodegeneration and recovery of brain functions4,5,6; the mechanisms of secondary brain injury and
restorative brain plasticity occurring simultaneously in these patients are still largely unknown. As a result, to make an accurate prognosis for recovery of consciousness and functional
outcomes is a major challenge for physicians treating patients with prolonged DoC. Current prognostic recommendations suggest a multimodal approach that includes serial standardized
behavioral assessments, neurophysiological techniques, and neuroimaging studies7. However, the results are not yet satisfactory, and there is a pressing need for new biomarkers to be
incorporated into current prognostic models to improve their sensitivity. In this context, circulating microRNAs (miRNAs) are good candidates, since each miRNA can reflect large-scale and
long-term genetic reorganizations following acute brain injuries8. miRNAs are a large class of small (18–25 nucleotides) non-coding RNAs that regulate gene expression in both physiological
and pathological contexts8. miRNAs act by degrading messenger RNAs, suppressing translation, or both, by binding to partially complementary sites in the 3′ untranslated region of target
genes9. To date, approximately 2000 miRNAs have been identified, which regulate the expression of about 60% of all genes and play a central role in many biological processes such as cell
cycle, cell metabolism, apoptosis, and immune response10,11. Circulating miRNAs can be detected using digital PCR (dPCR), a highly sensitive technique, and have been proposed as emerging
biomarkers for both acute brain injury and neurodegenerative diseases12,13. The analysis of miRNAs can provide a powerful tool for understanding the pathophysiology of prolonged DoC and
predicting patient outcomes. In particular, the analysis of miRNAs in patients with prolonged DoC can provide valuable insights into the molecular mechanisms occurring during brain
reorganization following a severe brain injury. In this longitudinal multicenter study, we selected five miRNAs involved in acute brain injury or chronic neurodegeneration (miRNAs 16-5p,
150-5p, 132-3p, 23b-3p and 451a) and evaluated them in patients with prolonged DoC caused by TBI or HIBI. Following TBI, a reduction in miRNA 16-5p levels has been observed both in plasma
during the acute phase and in the brain of patients with fatal TBI14,15. Changes in 150-5p levels have been observed both in traumatic injuries and neurodegenerative diseases16,17. miRNA
132-3p and 23b-3p have been identified as regulators of inflammatory and apoptotic pathways implicated in HIBI and neurodegenerative diseases18,19,20. Lastly, miRNA 451a has been recognized
for its role in governing apoptotic pathways following ischemia/reperfusion injury21. Our study aimed to provide a better understanding of the long-term genetic reorganization that occurs
after a severe brain injury and to identify new potential biomarkers for patients with prolonged DoC. METHODS PARTICIPANTS This multicenter longitudinal study enrolled patients admitted to
five Italian centers specialized in the rehabilitation of patients with prolonged DoC after an acute brain injury. Patients were included if they met the following criteria: (i) diagnosis of
UWS or MCS according to the Coma Recovery Scale-Revised (CRS-R) at the time of enrollment, (ii) DoC caused by TBI or HIBI, (iii) enrollment at 1–3 months after brain injury, (iv) clinical
and miRNA data availability at baseline and 6 months post-injury, and (v) age ≥ 18 years. Exclusion criteria were (i) previous brain injury and (ii) unstable clinical condition (e.g.,
hemodynamic instability, severe respiratory failure, or acute hydrocephalus). Patients’ data were compared with those of age-matched healthy controls without previous history of neurological
disease who were recruited from blood donors and healthcare personnel at the coordinating center. The study was conducted according to the guidelines of the Declaration of Helsinki and was
approved by the regional ethics review board (Palermo 1 Ethics Committee, Palermo, Italy: verbal no. 10/2021) of the coordinating center (Giuseppe Giglio Foundation, Cefalù, Italy). The
protocol was then ratified by the ethics committees of the other centers involved in the study. Patients’ legal guardians and healthy participants provided written informed consent which was
collected and stored at each participating center. CLINICAL EVALUATION At enrollment, all patients underwent standard neurological examination and assessment with the Italian version of the
Coma Recovery Scale Revised (CRS-R)22 at least 5 times over 3 consecutive days. The CRS-R is the most reliable tool for behavioral assessment in patients with DoC following a coma and is
used to diagnose UWS, MCS, and emergence from MCS23,24. It consists of 23 hierarchically organized items organized into six subscales evaluating auditory, visual, motor, oromotor/verbal,
communication, and arousal functions. The total score ranges from 0 (coma) to 23 (emergence from MCS). To minimize the risk of misdiagnosis, UWS and MCS diagnoses were accepted only if
confirmed at all 5 evaluation timepoints25. Outcomes were assessed 6 months post-injury using the CRS-R, the extended Glasgow Outcome Scale (GOSE) and the Functional Independence Measure
(FIM). The GOSE is a 1–8 global scale with the categories of death (score of 1), vegetative state, severe disability (lower and upper), moderate disability (lower and upper), and good
recovery (lower and upper; scores of 7 and 8)26. The FIM is an 18-item, seven-level ordinal scale that includes measures of independence for self-care, sphincter control, transfers,
locomotion, communication, and social cognition27. TOTAL RNA PURIFICATION At the end of initial and follow-up clinical evaluations, blood samples for miRNAs analysis were collected from
patients into serum-separating tubes. Samples were centrifuged at 1500 rpm for 15 min and stored at − 80 °C until use. Total RNA was purified using the miRNeasy Serum/Plasma Advanced Kit
(cat. No. 217204, QIAGEN) from 200 μL of serum, following the manufacturer’s instructions. This kit maximizes the recovery of small RNAs, including miRNAs, and is optimized for serum
samples. MIRNAS QUANTIFICATION Capillary electrophoresis is considered the most reliable method for accurately determining the integrity and the quantification of miRNAs, that are the major
factors affecting the outcome of downstream expression analyses28. Both quantity and quality of miRNAs have been assessed through chip-electrophoresis using the Agilent Small RNA Kit (cat.
no. 5067-1548, Agilent Technologies) on the Agilent 2100 Bioanalyzer. CDNA SYNTHESIS Starting from a fixed RNA input of 2.5 μL, cDNA synthesis was carried out using the miRCURY LNA™ RT Kit
(cat. no. 339340, QIAGEN) according to the manufacturer’s instructions. As recommended, cDNAs were diluted 1:30 prior to dPCR. DPCR Absolute quantification (positive particle = copies/μL) of
miRNAs was performed through dPCR with QIAcuity EG PCR Kit (cat. no. 250111, QIAGEN) and 26k-partitions nanoplates (cat. no. 250001, QIAGEN) on QIAcuity One platform. The used primers are
listed in Supplementary Table 1. Thermal cycling and imaging were performed following the manufacturer’s instructions. STATISTICAL ANALYSIS Demographic and clinical data are presented as
medians (interquartile range). The Mann–Whitney _U_ test for continuous variables, and the chi-square test for categorical variables were used to compare demographic and clinical data
between patients and healthy controls, and between groups of patients (TBI vs. HIBI) as well. The Student’s _t_-test was used to compare miRNA levels between patients and healthy controls
and between miRNA levels at 1–3 months (baseline) and 6 months post-injury. Correlations among all miRNA expressions were evaluated in healthy controls and patients (at baseline and
six-month post-injury) using Pearson’s correlation coefficients in correlation matrixes. Correlation coefficients range from − 1 to + 1, where − 1 indicates a total negative correlation
between two miRNAs, 0 no correlation, and + 1 a total positive linear correlation. The R-Squared was also calculated to measure the reliability of the linear relationship among miRNA
expressions included in the model. Its value ranges from 0 (fully correlated miRNAs) to 1 (non-correlated miRNAs). Multiple linear regression was used to determine the effect of each miRNA
and was adjusted for the effects of the other miRNAs. A linear model was constructed, in which the observed (dependent) variables were GOSE, FIM, or CRS-R scores 6 months post-injury in
patients with traumatic and hypoxic-ischemic etiologies, and the explanatory factors (regressors) all five miRNAs. _p_ values < 0.05 were considered significant. Statistical analyses were
performed with GraphPad Prism 8.0.0 (GraphPad Software, San Diego, California, USA; http://www.graphpad.com). RESULTS PARTICIPANT CHARACTERISTICS A total of 30 patients with prolonged DoC
and 35 age-matched healthy controls were included in this study, as shown in Table 1 and Supplementary Table 2. Of the patient cohort, 20 were diagnosed with UWS and 10 with MCS. The
underlying causes of DoC were TBI in 18 patients (15 of whom were involved in road accidents and 3 who suffered falls) and HIBI in 12 patients (10 of whom had cardiac arrests, 1 with opioid
overdose, and 1 with asphyxia). There were no significant differences between TBI and HIBI patients in terms of age, sex, and time since brain injury (Table 1). However, the TBI group had
more patients in MCS compared to the HIBI group. Additionally, patients with TBI had higher CRS-R and FIM scores at 6 months post-injury compared to those with HIBI (Table 1). CHANGES IN
MIRNA EXPRESSION AFTER TBI AND HIBI At the time of study inclusion, miRNA 150-5p expression was reduced both in patients with TBI (_p_ = 0.003) and HIBI (_p_ = 0.01) compared to healthy
controls (Fig. 1A). Six months post-injury, miRNA 150-5p expression returned to normal in patients with TBI, but not in patients with HIBI (_p_ = 0.03). miRNAs 132-3p and 23-b-3p expressions
were reduced only in patients with TBI at the time of study inclusion (_p_ values = 0.04 and 0.001, respectively) (Fig. 1B,C). Conversely, miRNA 451a expression was reduced only in patients
with HIBI at the time of study inclusion (_p_ = 0.001) (Fig. 1D). miRNA 16-5p expression did not change compared to healthy controls (Fig. 1E). No significant differences were found in
miRNA levels between TBI and HIBI patients at either time point. MIRNAS DYSHOMEOSTASIS AFTER TBI AND HIBI Pearson’s correlation matrixes showed that the expression of the selected miRNAs was
highly interrelated in healthy subjects, but this correlation was generally lost after the brain injury (Fig. 2 and Supplementary Table 3). Six months post-injury, most combinations
realigned with the normal trend, showing positive linear correlations (Fig. 2 and Supplementary Table 3). This trend was observed in both TBI and HIBI patients, but with a more evident
dyshomeostasis in the latter at baseline. Despite some differences related to etiology and evaluation timing, this data indicates a significant disruption in the reciprocal expression of
miRNAs 150-5p, 132-3p, 23b-3p and 451a, with only miRNA16-5p retaining a strong correlation with the other miRNAs both at baseline and 6 months post-injury. CORRELATION BETWEEN MIRNAS LEVELS
AND CLINICAL OUTCOMES Multiple regression analysis showed a positive correlation between miRNA 23b-3p levels 1–3 months post-injury and both FIM (_p_ = 0.01) and GOSE (_p_ = 0.006) scores 6
months post-injury in patients with TBI (Fig. 3A,B). Additionally, a positive correlation was found between miRNA 132-3p levels and GOSE scores in patients with TBI (_p_ = 0.03) (Fig. 3C).
No other significant correlations were observed. DISCUSSION In this longitudinal study on patients with prolonged DoC, we found that a severe brain injury triggers a reorganization of miRNAs
expression that (i) depends on the time elapsed since injury, (ii) is etiology-independent for some miRNA changes, and (iii) is etiology-specific for other miRNA alterations. In comparison
to healthy controls, patients with TBI exhibited lower levels of miRNAs 150-5p, 132-3p, and 23-b-3p at baseline, while patients with HIBI had lower levels of miRNA 150-5p at baseline and 6
months post-injury and a reduction of miRNA 451a at baseline. Moreover, higher levels of miRNA 132-3p and miRNA 23b-3p were associated with better outcomes in patients with TBI, as
demonstrated by improved GOSE and FIM scores 6 months post-injury. Our findings concur with previous research indicating a reduction in miRNA 150-5p expression both in a mouse model of TBI29
and in patients with Alzheimer's and Parkinson's diseases30,31, suggesting that miRNA 150-5p is altered in a wide range of pathological conditions from acute brain injury to
neurodegenerative diseases, making it a potential marker of brain injury independently of its etiology. Our study further showed that miRNA 150-5p expression was down-regulated in patients
with DoC, both after TBI and HIBI. One potential explanation for the link between miRNA-150-5p dysregulation and various pathological conditions is its involvement in neuroinflammation.
There is evidence of prolonged microglial activation after severe TBI and HIBI32,33,34 and miRNA-150-5p may inhibit the release of proinflammatory cytokines such as interleukine-1β,
interleukine-6, and tumor necrosis factor-α by binding to the protein kinase AKT331. Additionally, studies in patients with major trauma have shown that miRNA 150-5p targets the mRNA coding
for protein kinase C alpha, a key player in inflammation35. Thus, the down-regulation of miRNA-150-5p may contribute to persistent neuroinflammation following brain injury, and increasing
its expression may offer a new strategy for reducing neuroinflammation. In contrast to patients with TBI, downregulation of miRNA-150-5p persisted up to 6 months post-injury in patients with
HIBI. This suggests that in these patients, whose outcomes were worse than those with TBI, the biochemical relevant pathways dependent on miRNA 150-5p remain dysregulated for an extended
period post-injury. The expression of miRNA 451a was reduced in patients with HIBI 1–3 months post-injury, making it a time- and etiology-specific marker of brain injury that may be related
to inflammation. The down- or up-regulation of miRNA 451a has been shown to either increase or decrease neuroinflammation by modulating the levels of tumor necrosis factor α, interleukin‑1b,
interleukin‑6, and interleukin‑1836. In patients with TBI, miRNAs 132-3p and 23-b-3p were down-regulated 1–3 months after injury, making them specific markers for the timing and etiology of
brain injury. The miRNA132 family is involved in the formation of neuronal connections and in synaptic plasticity. Post-mortem studies have revealed a decrease in miRNA 132-3p in the
hippocampus, prefrontal cortex, and temporal cortex of patients with Alzheimer’s disease, including those in the early stages of disease37,38. miRNA 132 has been linked to various pathways,
including astrocyte-related inflammation39, synaptic structure and plasticity40,41, and cholinergic signaling42, all of which play key roles in Alzheimer's disease. TBI increases the
risk of developing dementia, including Alzheimer's disease, likely via mechanisms of long-term neurodegeneration43,44. Thus, the decrease in miRNA 132-3p levels may affect brain
plasticity and contribute to neurodegeneration in patients with prolonged post-traumatic DOC, impacting their outcomes. After 6 months, miRNA 132-3p levels returned to normal values when
most patients with post-traumatic DOC showed improvements in their level of consciousness. Therefore, the upregulation of miRNA 132-3p could have potential implications for brain plasticity
and the recovery of consciousness in post-traumatic DoC, as demonstrated by its association with improved cognitive abilities in animal models45. The expression of miRNA 23-b-3p exhibited a
similar pattern to miRNA 132-3p, with a decrease in patients with TBI at baseline. miRNA 23-b-3p plays a role in regulating neuronal apoptosis and is involved in various cellular pathways in
both physiological and pathological conditions20. A previous study on TBI patients and animal models has shown that miRNA 23-b levels decrease after TBI, and that overexpression of miRNA
23-b reduces neuronal autophagy in the hippocampus by targeting autophagy-related genes46. Our study expands upon this previous work by investigating patients with more severe injuries and a
different temporal window (1–3 months post-injury compared to 24 h post-injury) and demonstrating that miRNA 23-b-3p levels recover to normal after 6 months. This finding is significant
because it provides new insights into the dynamic changes in miRNA 23-b-3p levels after TBI and highlights the potential for miRNA 23-b-3p as a biomarker for post-traumatic DoC. The levels
of miRNA 16-5p, which have been linked to apoptosis induction and cell proliferation47,48, did not differ between healthy controls and patients with TBI or HIBI at 1–3 months and 6 months
post-injury. However, a previous study reported reduced levels of miRNA 16-5p in plasma of patients with severe TBI 25–72 h after injury compared to healthy controls or patients with
orthopedic injury14. Therefore, our findings suggest that the downregulation of miRNA 16-5p occurs only in the early phase after TBI. In terms of clinical relevance, the most noteworthy
finding was the correlation between the levels of miRNAs 132-3p and 23-b-3p 1–3 months post-injury and GOSE and FIM scores. Patients with higher expression of these miRNAs displayed better
functional outcomes 6 months after injury. Previous studies have linked decreased levels of miRNAs 132-3p and 23-b-3p with neurodegeneration in both animal models and patients with
Alzheimer's disease, while increased levels have been related to enhanced cognitive function37,45,46,49. Mechanisms of neurodegeneration and impaired plasticity have been described in
patients with prolonged DoC, such as amyloid-β deposition50, gray and white matter atrophy51,52,53, and decreased expression of brain-derived neurotrophic factor54. Elevated levels of miRNAs
132-3p and 23-b-3p may counteract neurodegeneration and promote brain plasticity in patients with DoC, leading to better outcomes. So, miRNAs 132-3p and 23-b-3p are promising candidates for
inclusion in prognostic models for patients with prolonged DoC and warrant further investigation as potential targets for therapeutic intervention. Studying the overall changes in miRNA
expression after a brain injury can provide a more comprehensive view of the impact of severe brain injuries on the miRNA regulatory network. We observed a loss of reciprocal correlations
among miRNAs, which was more pronounced 1–3 months post-injury and in patients with HIBI. The loss of reciprocal correlations among miRNAs may reflect a disruption of the complex molecular
network regulating neuronal survival and plasticity, and this dysregulation is more profound in patients with HIBI. Furthermore, the more pronounced dysregulation in the acute phase after
injury suggests that this is a critical time window for therapeutic intervention. The complex and simultaneous regulation of multiple biochemical pathways by each miRNA makes it challenging
to predict the final effects on neuronal survival and plasticity. Our understanding of this field is still limited, and further research is needed, such as developing computational models to
predict the combined effect of multiple miRNAs on patient outcomes. Two recent studies have investigated changes in miRNA expression in patients with prolonged DoC55,56. Although they
focused on different miRNAs or employed different methodologies compared to our study, their findings are consistent in suggesting that miRNAs represent an intriguing target for evaluation
in prolonged DoC. In patients with TBI, HIBI, or subarachnoid hemorrhage, Petrova and colleagues identified significant differences in the expression of miRNAs 93-5p, 21-5p, and 191-5p in
both cerebrospinal fluid and plasma, depending on the specific etiologies55. Zilliox and colleagues examined the whole blood miRNA profile in six patients with post-traumatic DoC lasting an
average of 1.5 years, discovering 48 miRNAs that were differentially expressed compared to controls56. While the findings of this study provide valuable insights into the potential role of
miRNAs as biomarkers for prognosis and targets for therapeutic intervention in patients with prolonged DoC, there are several limitations to consider. One of the primary limitations is the
small sample size, especially concerning HIBI and female patients, which may limit the generalizability of the results. Moreover, we evaluated miRNAs in the blood only, while analyzing
miRNAs in the cerebrospinal fluid may possibly provide more accurate results regarding the changes occurring in the central nervous system55,57. Additionally, our assessment of outcomes was
limited to a follow-up period of 6 months post-injury. While this time frame may be adequate to capture most of the recovery in patients with non-TBI etiologies, it may not fully evaluate
the complete recovery of patients with TBI, who may continue to improve up to 1-year post-injury3. Finally, while the study focused on miRNA dysregulation, it must be added that miRNAs are
just one of the many potential molecular mechanisms dysregulated in DoC, and further research is needed to fully understand the particularly complex pathophysiology of this condition. In
conclusion, this study offers important insights into the potential of miRNAs for understanding the molecular mechanisms of DoC as well as for enhancing prognosis and treatment in patients
with prolonged DoC. However, the limited sample size and the need for further research to fully understand the complex interactions of miRNAs and their effects on neuronal survival and
plasticity should be considered. Further studies with larger sample sizes and longer follow-up periods could help validate and expand upon these findings. DATA AVAILABILITY The data
supporting the findings of the study are available upon request from the corresponding author (S.B.). REFERENCES * Laureys, S. _et al._ Unresponsive wakefulness syndrome: A new name for the
vegetative state or apallic syndrome. _BMC Med._ 8, 68. https://doi.org/10.1186/1741-7015-8-68 (2010). Article PubMed PubMed Central Google Scholar * Giacino, J. T. _et al._ (2002) The
minimally conscious state: Definition and diagnostic criteria. _Neurology_ 58, 349–353 (2002). Article PubMed Google Scholar * Royal College of Physicians. _Prolonged Disorders of
Consciousness: National Clinical Guidelines_ (RCP, 2013). Google Scholar * Jassam, Y. N., Izzy, S., Whalen, M., McGavern, D. B. & El Khoury, J. Neuroimmunology of traumatic brain
injury: Time for a paradigm shift. _Neuron_ 95, 1246–1265 (2017). Article CAS PubMed PubMed Central Google Scholar * Lin, P. H., Kuo, L. T. & Luh, H. T. The roles of neurotrophins
in traumatic brain injury. _Life (Basel)_ 12, 26. https://doi.org/10.3390/life12010026 (2021). Article ADS CAS PubMed Google Scholar * Bagnato, S. The role of plasticity in the recovery
of consciousness. _Handb. Clin. Neurol._ 184, 375–395 (2022). Article PubMed Google Scholar * Giacino, J. T. _et al._ Practice guideline update recommendations summary: Disorders of
consciousness: Report of the guideline development, dissemination, and implementation subcommittee of the American Academy of Neurology; the American Congress of Rehabilitation Medicine; and
the National Institute on Disability, Independent Living, and Rehabilitation Research. _Neurology_ 91, 450–460 (2018). Article PubMed PubMed Central Google Scholar * Pritchard, C. C.,
Cheng, H. H. & Tewari, M. MicroRNA profiling: Approaches and considerations. _Nat. Rev. Genet._ 13, 358–369 (2012). Article CAS PubMed PubMed Central Google Scholar * Baek, D. _et
al._ The impact of microRNAs on protein output. _Nature_ 455, 64–71 (2008). Article ADS CAS PubMed PubMed Central Google Scholar * Bartel, D. P. MicroRNAs: Genomics, biogenesis,
mechanism, and function. _Cell_ 116, 281–297 (2004). Article CAS PubMed Google Scholar * Ha, M. & Kim, V. N. Regulation of microRNA biogenesis. _Nat. Rev. Mol. Cell. Biol._ 15,
509–524 (2014). Article CAS PubMed Google Scholar * Huibregtse, M. E., Bazarian, J. J., Shultz, S. R. & Kawata, K. The biological significance and clinical utility of emerging blood
biomarkers for traumatic brain injury. _Neurosci. Biobehav. Rev._ 130, 433–447 (2021). Article CAS PubMed PubMed Central Google Scholar * van den Berg, M. M. J. _et al._ Circulating
microRNAs as potential biomarkers for psychiatric and neurodegenerative disorders. _Prog. Neurobiol._ 185, 101732. https://doi.org/10.1016/j.pneurobio.2019.101732 (2020). Article PubMed
Google Scholar * Redell, J. B., Moore, A. N., Ward, N. H. 3rd., Hergenroeder, G. W. & Dash, P. K. Human traumatic brain injury alters plasma microRNA levels. _J. Neurotrauma_ 27,
2147–2156 (2010). Article PubMed PubMed Central Google Scholar * Pinchi, E. _et al._ Clinical-pathological study on β-APP, IL-1β, GFAP, NFL, Spectrin II, 8OHdG, TUNEL, miR-21, miR-16,
miR-92 expressions to verify DAI-diagnosis, grade and prognosis. _Sci. Rep._ 8, 2387. https://doi.org/10.1038/s41598-018-20699-1 (2018). Article ADS CAS PubMed PubMed Central Google
Scholar * Yang, J., Liang, Y., Han, H. & Qin, H. Identification of a miRNA signature in neutrophils after traumatic injury. _Acta Biochim. Biophys. Sin. (Shanghai)_ 45, 938–945 (2013).
Article CAS PubMed Google Scholar * Wen, Q. _et al._ Lead-exposure associated miRNAs in humans and Alzheimer’s disease as potential biomarkers of the disease and disease processes. _Sci.
Rep._ 12, 15966. https://doi.org/10.1038/s41598-022-20305-5 (2022). Article CAS PubMed PubMed Central Google Scholar * Qu, J. _et al._ MicroRNA-132-3p alleviates neuron apoptosis and
impairments of learning and memory abilities in Alzheimer’s disease by downregulation of HNRNPU stabilized BACE1. _Cell Cycle_ 20, 2309–2320 (2021). Article CAS PubMed PubMed Central
Google Scholar * Walgrave, H. _et al._ microRNA-132 regulates gene expression programs involved in microglial homeostasis. _iScience_ 26, 106829. https://doi.org/10.1016/j.isci.2023.106829
(2023). Article ADS CAS PubMed PubMed Central Google Scholar * Chen, Q. _et al._ MicroRNA-23a/b and microRNA-27a/b suppress Apaf-1 protein and alleviate hypoxia-induced neuronal
apoptosis. _Cell Death Dis._ 5, e1132. https://doi.org/10.1038/cddis.2014.92 (2014). Article CAS PubMed PubMed Central Google Scholar * Liu, Q. _et al._ microRNA-451 protects neurons
against ischemia/reperfusion injury-induced cell death by targeting CELF2. _Neuropsychiatr. Dis. Treat._ 14, 2773–2782 (2018). Article CAS PubMed PubMed Central Google Scholar *
Estraneo, A. _et al._ An Italian multicentre validation study of the coma recovery scale-revised. _Eur. J. Phys. Rehabil. Med._ 51, 627–634 (2015). CAS PubMed Google Scholar * Giacino, J.
T., Kalmar, K. & Whyte, J. The JFK Coma Recovery Scale-Revised: Measurement characteristics and diagnostic utility. _Arch. Phys. Med. Rehabil._ 85, 2020–2029 (2004). Article PubMed
Google Scholar * Seel, R. T. _et al._ Assessment scales for disorders of consciousness: Evidence-based recommendations for clinical practice and research. _Arch. Phys. Med. Rehabil._ 91,
1795–1813 (2010). Article PubMed Google Scholar * Wannez, S. _et al._ The repetition of behavioral assessments in diagnosis of disorders of consciousness. _Ann. Neurol._ 81, 883–889
(2017). Article PubMed Google Scholar * Wilson, J. T., Pettigrew, L. E. & Teasdale, G. M. Structured interviews for the Glasgow Outcome Scale and the extended Glasgow Outcome Scale:
Guidelines for their use. _J. Neurotrauma_ 15, 573–585 (1998). Article CAS PubMed Google Scholar * Heinemann, A. W., Linacre, J. M., Wright, B. D., Hamilton, B. B. & Granger, C.
Relationships between impairment and physical disability as measured by the functional independence measure. _Arch. Phys. Med. Rehabil._ 74, 566–573 (1993). Article CAS PubMed Google
Scholar * Biassoni, R. & Raso, A. _mRNA and microRNA Purity and Integrity: The Key to Success in Expression Profiling_ (Springer, 2014). Google Scholar * Ko, J. _et al._
Multi-dimensional mapping of brain-derived extracellular vesicle microRNA biomarker for traumatic brain injury diagnostics. _J. Neurotrauma_ 37, 2424–2434 (2020). Article PubMed PubMed
Central Google Scholar * Lugli, G. _et al._ Plasma exosomal miRNAs in persons with and without Alzheimer disease: Altered expression and prospects for biomarkers. _PLoS One_ 10, e0139233.
https://doi.org/10.1371/journal.pone.0139233 (2015). Article CAS PubMed PubMed Central Google Scholar * Li, H. _et al._ MicroRNA-150 serves as a diagnostic biomarker and is involved in
the inflammatory pathogenesis of Parkinson’s disease. _Mol. Genet. Genom. Med._ 8, e1189. https://doi.org/10.1002/mgg3.1189 (2020). Article Google Scholar * Ramlackhansingh, A. F. _et al._
Inflammation after trauma: Microglial activation and traumatic brain injury. _Ann. Neurol._ 70, 374–383 (2011). Article PubMed Google Scholar * Johnson, V. E. _et al._ Inflammation and
white matter degeneration persist for years after a single traumatic brain injury. _Brain_ 136, 28–42 (2013). Article PubMed PubMed Central Google Scholar * Radenovic, L. _et al._
Heterogeneity in brain distribution of activated microglia and astrocytes in a rat ischemic model of Alzheimer’s disease after 2 years of survival. _Aging (Albany, NY)_ 12, 12251–12267
(2020). Article CAS PubMed Google Scholar * Yang, J., Han, H., Zhao, Y. & Qin, H. Specific miRNA and its target in neutrophils after traumatic injury. _Acta Biochim. Biophys. Sin.
(Shanghai)_ 47, 749–754 (2015). Article CAS PubMed Google Scholar * Li, W., Dong, M., Chu, L., Feng, L. & Sun, X. MicroRNA-451 relieves inflammation in cerebral ischemia-reperfusion
via the Toll-like receptor 4/MyD88/NF-κB signaling pathway. _Mol. Med. Rep._ 20, 3043–3054 (2019). CAS PubMed PubMed Central Google Scholar * Lau, P. _et al._ Alteration of the microRNA
network during the progression of Alzheimer’s disease. _EMBO Mol. Med._ 5, 1613–1634 (2013). Article CAS PubMed PubMed Central Google Scholar * Pichler, S. _et al._ The miRNome of
Alzheimer’s disease: Consistent downregulation of the miR-132/212 cluster. _Neurobiol. Aging_ 50, 167.e1-167.e10. https://doi.org/10.1016/j.neurobiolaging.2016.09.019 (2016). Article CAS
PubMed Google Scholar * Kong, H. _et al._ The effect of miR-132, miR-146a, and miR-155 on MRP8/TLR4-induced astrocyte-related inflammation. _J. Mol. Neurosci._ 57, 28–37.
https://doi.org/10.1007/s12031-015-0574-x (2015). Article CAS PubMed Google Scholar * Magill, S. T. _et al._ microRNA-132 regulates dendritic growth and arborization of newborn neurons
in the adult hippocampus. _Proc. Natl. Acad. Sci. U.S.A._ 107, 20382–20387 (2010). Article ADS CAS PubMed PubMed Central Google Scholar * Remenyi, J. _et al._ miR-132/212 knockout mice
reveal roles for these miRNAs in regulating cortical synaptic transmission and plasticity. _PLoS One_ 8, e62509 (2013). Article ADS CAS PubMed PubMed Central Google Scholar *
Shaltiel, G. _et al._ Hippocampal microRNA-132 mediates stress-inducible cognitive deficits through its acetylcholinesterase target. _Brain Struct. Funct._ 218, 59–72 (2013). Article CAS
PubMed Google Scholar * Brett, B. L., Gardner, R. C., Godbout, J., Dams-O’Connor, K. & Keene, C. D. Traumatic brain injury and risk of neurodegenerative disorder. _Biol. Psychiatry_
91, 498–507 (2022). Article CAS PubMed Google Scholar * Bagnato, S. & Boccagni, C. Moderate/severe traumatic brain injury as a trigger of chronic neurodegeneration in humans. _Neural
Regen. Res._ 15, 1247–1248. https://doi.org/10.4103/1673-5374.272574 (2020). Article CAS PubMed PubMed Central Google Scholar * Hansen, K. F. _et al._ miRNA-132: A dynamic regulator of
cognitive capacity. _Brain Struct. Funct._ 218, 817–831 (2013). Article ADS PubMed Google Scholar * Sun, L. _et al._ miR-23b improves cognitive impairments in traumatic brain injury by
targeting ATG12-mediated neuronal autophagy. _Behav. Brain Res._ 340, 126–136 (2018). Article CAS PubMed Google Scholar * Cimmino, A. _et al._ miR-15 and miR-16 induce apoptosis by
targeting BCL2. _Proc. Natl. Acad. Sci. U.S.A._ 102, 13944–13949 (2005). Article ADS CAS PubMed PubMed Central Google Scholar * Kaddar, T. _et al._ Two new miR-16 targets: Caprin-1 and
HMGA1, proteins implicated in cell proliferation. _Biol. Cell_ 101, 511–524 (2009). Article CAS PubMed Google Scholar * Jiang, H. _et al._ miR-23b-3p rescues cognition in Alzheimer’s
disease by reducing tau phosphorylation and apoptosis via GSK-3β signaling pathways. _Mol. Ther. Nucleic Acids_ 28, 539–557 (2022). Article CAS PubMed PubMed Central Google Scholar *
Bagnato, S. _et al._ Dissociation of cerebrospinal fluid amyloid-β and tau levels in patients with prolonged posttraumatic disorders of consciousness. _Brain Inj._ 32, 1056–1060 (2018).
Article PubMed Google Scholar * Annen, J. _et al._ Regional brain volumetry and brain function in severely brain-injured patients. _Ann. Neurol._ 83, 842–853 (2018). Article CAS PubMed
Google Scholar * Bagnato, S. _et al._ Prolonged cerebrospinal fluid neurofilament light chain increase in patients with post-traumatic disorders of consciousness. _J. Neurotrauma_ 34,
2475–2479 (2017). Article PubMed Google Scholar * Bagnato, S. _et al._ Sustained axonal degeneration in prolonged disorders of consciousness. _Brain Sci._ 11, 1068.
https://doi.org/10.3390/brainsci11081068 (2021). Article PubMed PubMed Central Google Scholar * Bagnato, S. _et al._ Serum BDNF levels are reduced in patients with disorders of
consciousness and are not modified by verticalization with robot-assisted lower-limb training. _Neural Plast._ 2020, 5608145. https://doi.org/10.1155/2020/5608145 (2020). Article CAS
PubMed PubMed Central Google Scholar * Petrova, T. A. _et al._ miR-21, miR-93, miR-191, miR-let-7b, and miR-499 expression level in plasma and cerebrospinal fluid in patients with
prolonged disorders of consciousness. _Neurol. Int._ 15, 40–54 (2022). Article PubMed PubMed Central Google Scholar * Zilliox, M. J. _et al._ An initial miRNA profile of persons with
persisting neurobehavioral impairments and states of disordered consciousness after severe traumatic brain injury. _J. Head Trauma Rehabil._ 38, E267–E277 (2023). Article PubMed Google
Scholar * Bagnato, S. & Boccagni, C. Cerebrospinal fluid and blood biomarkers in patients with post-traumatic disorders of consciousness: A scoping review. _Brain Sci._ 13, 364.
https://doi.org/10.3390/brainsci13020364 (2023). Article CAS PubMed PubMed Central Google Scholar Download references AUTHOR INFORMATION Author notes * These authors contributed
equally: Nicolò Musso and Dalida Bivona. AUTHORS AND AFFILIATIONS * Department of Biomedical and Biotechnological Sciences, University of Catania, 95123, Catania, Italy Nicolò Musso, Dalida
Bivona, Carmelo Bonomo, Paolo Bonacci & Stefania Stefani * Molecular Biology Laboratory, Giuseppe Giglio Foundation, 90015, Cefalù, Italy Maria Enza D’Ippolito * Unit of Neurophysiology
and Unit for Severe Acquired Brain Injuries, Giuseppe Giglio Foundation, 90015, Cefalù, Italy Cristina Boccagni, Francesca Rubino & Sergio Bagnato * Cardinal Ferrari Center, 43012,
Fontanellato, Italy Antonio De Tanti * RAN (Research in Advanced Neuro-Rehabilitation), S. Anna Institute, 80067, Crotone, Italy Lucia Francesca Lucca * Neurorehabilitation and Spinal Units,
Istituti Clinici Scientifici Maugeri IRCCS, 27100, Pavia, Italy Valeria Pingue * Montecatone Rehabilitation Institute, 40026, Imola, Italy Valentina Colombo * Don Gnocchi Foundation IRCCS,
50124, Florence, Italy Anna Estraneo * Clinical Pathology Laboratory, Provincial Health Authority of Caltanissetta, 93100, Caltanissetta, Italy Maria Andriolo Authors * Nicolò Musso View
author publications You can also search for this author inPubMed Google Scholar * Dalida Bivona View author publications You can also search for this author inPubMed Google Scholar * Carmelo
Bonomo View author publications You can also search for this author inPubMed Google Scholar * Paolo Bonacci View author publications You can also search for this author inPubMed Google
Scholar * Maria Enza D’Ippolito View author publications You can also search for this author inPubMed Google Scholar * Cristina Boccagni View author publications You can also search for this
author inPubMed Google Scholar * Francesca Rubino View author publications You can also search for this author inPubMed Google Scholar * Antonio De Tanti View author publications You can
also search for this author inPubMed Google Scholar * Lucia Francesca Lucca View author publications You can also search for this author inPubMed Google Scholar * Valeria Pingue View author
publications You can also search for this author inPubMed Google Scholar * Valentina Colombo View author publications You can also search for this author inPubMed Google Scholar * Anna
Estraneo View author publications You can also search for this author inPubMed Google Scholar * Stefania Stefani View author publications You can also search for this author inPubMed Google
Scholar * Maria Andriolo View author publications You can also search for this author inPubMed Google Scholar * Sergio Bagnato View author publications You can also search for this author
inPubMed Google Scholar CONTRIBUTIONS N.M., S.S., M.A., and S.B. designed this work. N.M., D.B., Ca.B., and P.B. performed miRNA analyses. M.E.D.I., Cr.B., F.R., A.D.T., L.F.L., V.P., and
V.C. collected blood samples and clinical data. N.M. performed the statistical analysis. N.M. and S.B. wrote the manuscript. Cr.B., A.E., and S.B. revised the manuscript. CORRESPONDING
AUTHOR Correspondence to Sergio Bagnato. ETHICS DECLARATIONS COMPETING INTERESTS The authors declare no competing interests. ADDITIONAL INFORMATION PUBLISHER'S NOTE Springer Nature
remains neutral with regard to jurisdictional claims in published maps and institutional affiliations. SUPPLEMENTARY INFORMATION SUPPLEMENTARY TABLES. RIGHTS AND PERMISSIONS OPEN ACCESS This
article is licensed under a Creative Commons Attribution 4.0 International License, which permits use, sharing, adaptation, distribution and reproduction in any medium or format, as long as
you give appropriate credit to the original author(s) and the source, provide a link to the Creative Commons licence, and indicate if changes were made. The images or other third party
material in this article are included in the article's Creative Commons licence, unless indicated otherwise in a credit line to the material. If material is not included in the
article's Creative Commons licence and your intended use is not permitted by statutory regulation or exceeds the permitted use, you will need to obtain permission directly from the
copyright holder. To view a copy of this licence, visit http://creativecommons.org/licenses/by/4.0/. Reprints and permissions ABOUT THIS ARTICLE CITE THIS ARTICLE Musso, N., Bivona, D.,
Bonomo, C. _et al._ Investigating microRNAs as biomarkers in disorders of consciousness: a longitudinal multicenter study. _Sci Rep_ 13, 18415 (2023).
https://doi.org/10.1038/s41598-023-45719-7 Download citation * Received: 27 February 2023 * Accepted: 23 October 2023 * Published: 27 October 2023 * DOI:
https://doi.org/10.1038/s41598-023-45719-7 SHARE THIS ARTICLE Anyone you share the following link with will be able to read this content: Get shareable link Sorry, a shareable link is not
currently available for this article. Copy to clipboard Provided by the Springer Nature SharedIt content-sharing initiative