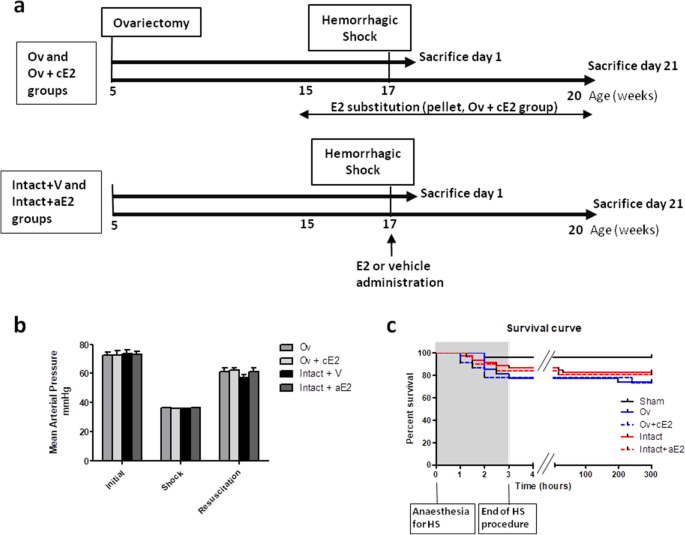
- Select a language for the TTS:
- UK English Female
- UK English Male
- US English Female
- US English Male
- Australian Female
- Australian Male
- Language selected: (auto detect) - EN
Play all audios:
ABSTRACT The protective effect of estrogens against chronic glomerular diseases is admitted but remains debated during acute kidney injury (AKI). Using a model of resuscitated hemorrhagic
shock in C57/Bl6 female mice, this study evaluated at 1 and 21 days the renal effect of (1) endogenous estrogen, using ovariectomized mice with or without chronic estrogen restoration, or
(2) exogenous estrogen, using a single administration of a pharmacological dose during shock resuscitation. In both ovariectomized and intact mice, hemorrhagic shock induced epithelial cell
damages (assessed by KIM-1 renal expression) with secondary renal fibrosis but without significant decrease in GFR at day 21. Ovariectomy with or without estrogen restoration have no
significant effect on renal damages and dysfunction. This lack of effect was associated with a marked (> 80%) reduction of total kidney GPR30 expression. By contrast, a single high dose
of estradiol in intact mice reduced renal KIM-1 expression by 2/3, attenuated the severity of cell death related to pyroptosis, and prevented the increase of fibrosis by 1/3. This provides a
rationale to investigate the benefits of a single administration of estrogen or estrogen modulators during acute kidney injuries in males. Furthermore, the cost/benefit ratio of such
administration should be investigated in Human. SIMILAR CONTENT BEING VIEWED BY OTHERS FEMALE HORMONES PREVENT SEPSIS-INDUCED CARDIAC DYSFUNCTION: AN EXPERIMENTAL RANDOMIZED STUDY Article
Open access 23 March 2022 ESTRADIOL-INDUCED INHIBITION OF ENDOPLASMIC RETICULUM STRESS NORMALIZES SPLENIC CD4 + T LYMPHOCYTES FOLLOWING HEMORRHAGIC SHOCK Article Open access 05 April 2021
DECREASED RENAL EXPRESSION OF PAQR5 IS ASSOCIATED WITH THE ABSENCE OF A NEPHROPROTECTIVE EFFECT OF PROGESTERONE IN A RAT UUO MODEL Article Open access 08 August 2023 INTRODUCTION Although
estrogens are principally reproductive hormones, their receptors are widely expressed in the body and contribute to multiple non-reproductive effects that include cardiovascular actions,
metabolic regulation, and tissue trophicity and repair1. Among non-reproductive organs, kidneys express one of the highest levels of estrogen receptors (ER), particularly ERα2.
Paradoxically, descriptions of renal actions of endogen estrogen are sparse and appear mostly related to water, electrolyte homeostasis and glomerular matrix turn-over3,4,5,6. Data from the
literature also supports the potential renal protective effect of endogen estrogen during Chronic Kidney Disease (CKD). Indeed, before menopause, CKD evolves more slowly in women than in
men, particularly among patients with diabetes or IgA nephropathy7,8,9. The protective effect of endogen estrogen has been described in various experimental models of CKD: diabetic
nephropathy, glomerulonephritis, and unilateral nephrectomy. This effect is generally lost in case of ovariectomy but in most cases, is restored with exogenous estrogen supplementation10,11.
Less is known regarding the potential renal protective effects during acute kidney injury (AKI). In humans, females appear to be protected against organ failure after major hemorrhage12.
However, mechanisms of this gender dimorphism, that likely involve hormonal status, remain to be demonstrated in the absence of clinical trials13. Interestingly, pregnancy, a status with a
higher estrogen impregnation, is associated with reduced trauma mortality when compared to matched non-pregnant women—a comparison that excludes a simple gender effect14. Conversely, a
recent prospective study has shown that during septic shock, higher serum estradiol levels were associated with lower survival and a higher risk of AKI, but most patients in this study were
males. Regarding experimental data, estrogen, either endogenous or exogenous, have been associated with significant benefits in various models of AKI such as cisplatin kidney toxicity15,
renal ischemia reperfusion16,17 and cardiac arrest18,19. Hemorrhagic shock, a highly relevant situation in clinical practice, is often responsible for acute kidney injury that worsens the
prognosis20. Among protective pharmacological interventions, administration of estrogen has been proposed in females and males alike. Despite the evidence of some benefits regarding cell
protection and the modulation of inflammatory response13, the practical renal benefit (i.e. renal function and fibrosis) remains to be demonstrated. Furthermore, most works have not
considered the distinction between estrogen restoration at physiological doses after ovariectomy and the administration of a high pharmacological dose of estrogen in intact animals. We have
previously developed a model of pressure-controlled resuscitated hemorrhagic shock in mice that exhibits a good level of anthropomorphism21,22,23. Based on this model, the present study was
designed to evaluate the renal protective effect of estrogen in two situations: (1) restoration of estrogen impregnation in ovariectomized mice; (2) additional administration of a single
bolus of estrogen at the time of resuscitation in intact female mice. We evaluated early renal damages and sequels. Regarding mechanisms, we focused on epithelial cell death related to
pyroptosis that has been involved during ischemic AKI24 and that could contribute to inflammatory response and secondary fibrosis, potential targets for estrogen as shown in the brain25.
RESULTS Protocol design is described in Fig. 1a. EFFECT OF HEMORRHAGIC SHOCK PROCEDURE Initial mean arterial blood pressure was not different between experimental groups (73.0 ± 1.1 mmHg,
Fig. 1b). Ovariectomy (Ov group) and chronic E2 substitution (Ov + cE2 group) had no impact on basal blood pressure. During the hemorrhagic shock procedure (2 h), mean arterial blood
pressure was 36.1 ± 0.1 mmHg, for a target of 35 mmHg, and was not different between groups. Mean blood volume removal necessary to reach this pressure was 0.7 mL and was not different
between groups. Mean blood pressure during resuscitation (blood restitution and Ringer’s lactate infusion, approximately 20 min) was 59.6 ± 1.2 mmHg and was similar between groups. We
therefore considered that the severity of the hemorrhagic procedure was similar between groups. Death rates consecutive to hemorrhagic shock procedure were 17% in the intact shocked mice
(Intact + V group), 26% in the Ov group, 25% in the Ov + cE2 group and 20% in the intact mice treated with a single administration of estradiol (Intact + aE2 group). These slight differences
in mortality were not statistically different. As shown is Fig. 1c, most of the mortality resulted from mice that died during or immediately after the HS procedure. RENAL IMPACT OF
HEMORRHAGIC SHOCK KIM-1 (Kidney-Injury-Molecule-1) was used as an early marker of acute tubular injury (Fig. 2a). One day after Acute Kidney Injury (AKI), KIM-1 was increased in all shocked
mice. There was no significant difference between intact and ovariectomized mice for KIM-1 expression. By contrast, a single intravenous bolus of estradiol (25 µg) administered at the end of
resuscitation in non-ovariectomized female mice significantly reduced the increase in KIM-1 after HS when compared to vehicle treated mice. Creatininemia did not increase in intact shocked
mice whereas a subtle increase was detected in ovariectomized mice, whether substituted with estradiol or not (Fig. 2b). Creatininemia remained unaffected by acute estradiol administration.
Twenty-one days after AKI, shocked mice recovered a normal GFR (Fig. 2c) that was not significantly different between groups, although it tended to be slightly lower in Ov group. Similarly,
creatininemia was not different between groups. However, shocked animals had developed significant renal fibrosis, as assessed by immunohistochemical analysis of collagen III deposition
(Fig. 2d,e,f). Fibrosis was slightly more pronounced in ovariectomized mice, whether they were supplemented or not and was lower than in Intact + V and Intact + aE2 mice. Finally, the lack
of endogenous estrogen did not worsen acute kidney injury and estrogen substitution in ovariectomized mice did not prevent the renal impact of hemorrhagic shock. By contrast a single
administration of estradiol at the end of HS in intact mice protected against KIM-1 induction at day 1 and fibrosis development 21 days later. UTERUS WEIGHT AND ESTROGEN RECEPTOR EXPRESSION
In order to verify the absence of estrogen impregnation in ovariectomized mice, we measured the weight of the uterus at days 1 and 21. Uterus weight showed a strong decrease in the Ov group
compared to all other groups. In contrast, estrogen restoration using pellets resulted in a uterus weight similar to that of intact mice (Fig. 3a,e). The expressions of estrogen receptors
ERα, ERβ and GPR30 in whole kidney extracts were studied by RT-PCR. GPR30 mRNA expression was significantly reduced in all shocked groups one day after HS and remained significantly lower
than the sham group in the Ov + cE2 group at day 21, whereas other groups were similar to the sham (Fig. 3b,f). ERα mRNA expression seemed to have decreased after HS (at day 1) in all
shocked animals compared to the sham, but this difference was not significant (Fig. 3c). At day 21, ERα expression was similar in all groups (Fig. 3g). ERβ expression was quite
heterogeneous, but values were similar in all groups for both day 1 and day 21 (Fig. 3d,h). MECHANISMS INVOLVED IN THE RENAL EFFECT OF EXOGENOUS ESTROGEN We have explored mechanisms
potentially involved in the mediation and modulation of damages, during the first 24 h following AKI and at the stage of tubular repair (3 weeks). Regarding cell death, programmed cell death
apoptosis and pro-inflammatory cell death pyroptosis were studied since both have been described during renal ischemic injuries. APOPTOSIS Programmed cell death was quantified by TUNEL,
which stains degraded nuclei (Fig. 4a). Paradoxically, the sham group exhibited cell death, suggesting that the anesthesia and surgery performed on these animals had detrimental effects to
some extents. Cell death following shock was increased in ovariectomized mice compared to intact. This increase appeared attenuated in Ov + cE2 mice. Cell death in both the control and
estradiol groups was similar to that of the sham. The contribution of apoptosis was checked using caspase 8 protein expression, the initiator and specific marker of the classical apoptosis
pathway. In the sham and shocked groups, the expression of caspase 8 mRNA was not different (Fig. 4b). However, a differential expression of its active cleaved form (p18) studied using WB
(Fig. 4c,d), was observed. The sham group already presented a high expression of this active form that was not different from that of the Intact + Vehicle and ovariectomized shocked mice. By
contrast, the expression of activated caspase 8 was significantly lower in estradiol-treated mice. PYROPTOSIS We further examined markers for pyroptosis. The TLR4-induced inflammasome
cascade (TLR4 – NLRP3 – caspase 1 – IL1β) appeared to be recruited during shock in intact and ovariectomized animals even if the changes in TLR4 and NLRP3 mRNA expression did not reach
significance because of individual disparity (Fig. 5). In the group that has received the bolus of estradiol (Intact + aE2), TLR4 and IL1β expression was significantly reduced with a similar
trend for NLRP3. The caspase 1-cleaved form was increased by HS in intact mice in the vehicle group. The bolus of estradiol partially prevented this increase and results were not different
from that of the sham group. Pyroptosis activation involves endoreticular stress. Expression of GPR78 and the transcription factor CHOP produced by the endoplasmic reticulum in case of
stress were increased in shocked mice, particularly in the Ov group (Fig. 6). Conversely, these expressions remained similar to that of sham mice in the acute estradiol group. Caspase 11 is
specific to the pyroptosis pathway and its synthesis is activated by CHOP. Caspase 11 mRNA expression was studied using qPCR and its active form (p20) was assessed by WB (Fig. 6c–e). Caspase
11 mRNA expression was increased in all shocked groups but the intact + aE2 group in which the expression was similar to the sham group. Activated caspase 11 expression was similarly
modified by shock and by acute administration of E2. Particularly, activated caspase 11 in the intact + aE2 group was significantly decreased when compared to intact + vehicle and appeared
similar to the sham group. DISCUSSION The key findings from this study are that during hemorrhagic shock in female mice, i) endogenous estrogens do not reduce death rate, acute kidney injury
intensity, and secondary renal fibrosis, whereas ii) a single high-dose administration of estradiol during resuscitation in non-ovariectomized shocked mice exerts a protective effect
associated with a reduced recruitment of renal pyroptosis pathway. Endogenous estrogens are considered nephroprotective during chronic kidney disease7,8,9. This assumption is driven by both
epidemiological data obtained before and after menopause in women and experimental data obtained in female rodents when comparing intact versus ovariectomized mice with or without estrogen
restoration. These promising results are challenged by conflicting results in humans26. In particular, long-term estrogen treatment after menopause may negatively affect glomerular
filtration rate in women with previous renal damages27. During acute kidney injury, the benefits of estrogen also appear encouraging even if sparse and disparate. Several studies suggest
that estrogen could limit renal damages in a number of models of AKI, including cardiac arrest, ischemia–reperfusion and cisplatin toxicity15,18,28. In these investigations, estradiol was
administrated at a high dosage, most often in male (mice or rat) or ovariectomized female rodents. These studies had focused on early consequences regarding renal histology or function but
not on delayed renal repair or sequels. AKI induced by hemorrhagic shock in mice is a potentially anthropomorphic situation. To our knowledge, renal effects of estrogen during hemorrhagic
shock in female rodents have not been studied yet, probably because these models are demanding. We have previously described a model of resuscitated hemorrhagic shock in female mice23 that
was associated with approximately 20% death, acute kidney damage early in the protocol and renal functional recovery after 21 days with moderate renal fibrotic sequels. Herein, we used this
model to establish the renal effects of: i) ovariectomy and of estrogen restoration; ii) an acute dose of estradiol given at the time of resuscitation in mice with intact gonadic functions.
In the present study, the effect of a bolus of estradiol in Ov mice has not been investigated in detail in order to limit group multiplicity and because preliminary studies had not shown any
differences with the Ov + cE2 group. In the present work, the loss of estrogen impregnation resulting from ovariectomy in adult mice only slightly worsened the functional impact of
hemorrhagic shock and had no significant effects on kidney histology. Additionally, the group of ovariectomized mice that received a pellet-based estradiol supplementation at 15 weeks and
that was submitted to the shock 2 weeks later was not protected from renal impact despite the obvious effect of estradiol assessed by uterus weight determination29. The dose of estrogen we
used was slightly supraphysiologic and completely prevented uterus atrophy. Such a dose has been shown to exert a protective effect in a model of skin flap necrosis in mice30. By contrast,
our results suggest that endogenous estrogens in adult female mice do not play a noticeable protective role against acute hemodynamic injury. Of interest, estrogen receptors expressions
(GPR30, ERα and ERβ) were not significantly altered in ovariectomized mice when compared to sham mice or intact shocked mice. The only significant difference (at day 21) was a marked and
persistent decrease in GPR30 mRNA expression in Ov + cE2 mice. The down-regulation of GPR30 could have contributed to limiting the protective effect of estrogen in the Ov + cE2 group since
transmembranous GPR30 receptor appeared to contribute to estradiol-related vascular protection31. The lack of protective effects of estrogen restoration in our study contrasts with the
beneficial renal effect observed in a previous work that used a model of ischemia reperfusion in mice17,28. However, the dose of estrogen used in the above study was almost twenty times
higher than in the present work. Thus, a differential action of such doses on ERα and ERβ receptors could have contributed to the beneficial effects of estradiol on this model. Such dose
effect has already been described in the kidney6. Finally, the benefit observed in ovariectomized mice with high doses were quite similar to what we have observed with a single bolus of a
high dose of estradiol in non-ovariectomized mice. Another explanation for the lack of efficiency that we have observed with a lower and more physiological dose could be that either the
ovarian nephroprotective effect results from a more complex hormonal equilibrium, or that the delay in estradiol restoration following ovariectomy was too long, thus leading to an
irreversible loss of function of this hormonal system despite persistent ERα and ERβ expression32,33,34. Complementary work is required to determine if the time interval between the loss of
ovarian function and estradiol supplementation could explain the ineffectiveness of estrogen restoration. The administration of a single bolus of estrogen at a high dose has been studied in
male rats for which it was effective in improving the survival from hemorrhagic shock35. To our knowledge, the potential benefit of such a single dose has not been studied in
non-ovariectomized female mice. Nonetheless, estradiol concentration can reach high values during a female’s life, particularly during pregnancy29. In this case, high concentrations of
estrogen result in additional effects that could, for instance, contribute to the high tolerance of pregnant female during postpartum hemorrhage14. This prompted us to explore the
consequences of an acute administration of estrogen in non-ovariectomized mice at the time of resuscitation in our model of AKI. The amount of estradiol used for the bolus in the present
study was largely supra physiological. However, it was within the range of single doses used in previous studies18,19,36. The bolus of estradiol was able to limit the initial renal impact of
hemorrhagic shock as assessed by KIM-1 expression. Three weeks later, the increase in fibrosis assessed by Sirius red staining was also reduced when compared to non-estradiol-treated
shocked animals. However, the mild renal impact of hemorrhagic shock in our model using C57Bl6 strain made it difficult to determine if the protective effect of estradiol that we observed
was clinically relevant. Nevertheless, this effect allowed us to examine the potential mechanisms of estrogen renal protection in this model of AKI. The single bolus of estradiol partly
prevented the rise in KIM-1 mRNA expression following shock. Total cell death assessed by TUNEL was slightly lower. Moreover, the mechanism of cell death appeared to have been shifted from a
pyroptosis pathway profile to the more classical apoptosis pathway. At the same time, inflammasome response was also modified. It has been recently shown that the pyroptosis pathway could
be activated in epithelial renal cells24. This pathway is particularly immunogenic, resulting in a pronounced inflammatory response37,38. First identified in macrophages where it results
from an activation of TLR4, pyroptosis appears possible in all cells through the activation of DAMPS (Damage / Danger-Associated Molecular Patterns)39,40 or directly through endoplasmic
reticulum stress. Endoplasmic reticulum stress is a key early event of cellular aggression, which may lead to cell death by pyroptosis via the activation of the transcription factor C/EBP
Homologous Protein (CHOP). This activation of CHOP induces, in turn, that of caspase 11 and the recruitment of inflammasome. In our study, hemorrhagic shock in female mice appeared to
recruit pyroptosis pathways whereas in mice that received the bolus of estradiol, activation of that pathway was largely prevented. It is possible that the bolus of estrogen given during
resuscitation modulated endoplasmic reticulum stress, possibly via GPR30, thus impacting cell death pathways and the resulting inflammation. Neither suppression of endogenous estrogen
production by ovariectomy nor restoration of estrogen impregnation using the prolonged administration of a physiological dose of estradiol had any significant impact in our model of
hemorrhagic shock. In contrast, the present work provides evidences for a renal protective effect of a pharmacological dose of estradiol when acutely given at the time of resuscitation in
the same model with intact ovarian functions. Whereas post-ovariectomy estrogen restoration largely questions about timing of restoration, dose and duration, the benefit of an immediate and
large amount of estrogen during hemorrhagic shock suggests the recruitment of other supra-physiologic effects of this hormone41. Whether additional estradiol administration would further
improve even more kidney protection has not been studied here. This limitation will require further work. However, the interest in the single administration of estrogen at the time of
aggression relies in a lower risk of adverse events, potentially allowing their extended use during hemorrhagic shock. Even though morphological and functional damages were moderate in our
model, this provides a rationale to extend the investigation regarding the potential protective effects of a single administration of estrogen on survival and renal impact in human. Finally,
whereas estrogen deprivation or restoration has minor effects, a single bolus of estradiol ameliorates renal injury in hemorrhagic shock in female mice. Further experiments should test the
hypothesis in males. The results also support the study on the role of estradiol in human patients with hemorrhagic shock. MATERIALS AND METHODS EXPERIMENTAL MODEL Adult C57BL/6J female mice
were purchased from Janvier Labs (Le Genest Saint Isle, France) and housed in a pathogen-free, temperature-controlled environment with a 12-h/12-h light/dark photocycle, as described42.
Animals had free access to food and tap water. Animal experimentations were performed according to the national and institutional animal care and ethical guidelines and were approved by the
animal care and use committee UMS US006/INSERM (Toulouse, France). Protocol design is described in Fig. 1a. We studied the consequence of hemorrhagic shock (HS) in both: (1) endogenous
estrogen depletion (by ovariectomy) with or without chronic restoration by subcutaneous estradiol pellets; (2) A single acute administration of estrogen in intact mice. For this purpose, 5
groups of mice were used: (1) Ovariectomized mice with HS (Ov); (2) Ovariectomized mice with subcutaneous estradiol pellets (0.1 mg E2, Innovative Research of America, releasing 80
µg/kg/day), with HS (Ov + cE2); (3) Intact mice with HS (Intact + V); (4) Intact mice treated with a single administration of 1 mg/kg of estradiol at the end of HS procedure (Intact + aE2);
(5) Sham-operated mice. One part of the mice was euthanized 1 day after HS procedure and the remaining part 21 days after HS. OVARIECTOMY At the age of 5 weeks, mice from the Ov and Ov + cE2
groups were anesthetized with intraperitoneal injection of ketamine 125 mg/kg and xylazine 10 mg/kg. A small incision was made on the flank of the animal; the ipsilateral ovary was released
and removed after ligation of the uterus. The muscle layers and skin were sutured separately. The same procedure was repeated on the other side. Non-ovariectomized females (Intact)
underwent the same anesthetic and surgical protocol but without ovariectomy. ESTRADIOL TREATMENTS CHRONIC SUBSTITUTION Two weeks before hemorrhagic shock, subcutaneous estradiol pellets (0.1
mg E2, Innovative Research of America, releasing 80 µg/kg/day) were placed at the nape of the neck. SINGLE ADMINISTRATION Cyclodextrin-encapsulated 17β-estradiol (β-estradiol-water soluble,
Sigma E4389, 25 µg diluted in 0.2 mL of saline) or vehicle (0.2 mL of saline) was given intravenously at the time of the resuscitation from HS. HEMORRHAGIC SHOCK PROCEDURE HS was performed
as previously described23. Animals were anesthetized with ketamine and xylazine (125 mg/kg and 10 mg/kg, respectively) and intubated using an intra-tracheal cannula. Mechanical ventilation
(9 mL/kg, 150 min−1) was carried out with a specific ventilator Minivent 845 (Hugo Sachs Elektronik, March-Hugstetten, Germany). Animal body temperature was continuously monitored and
maintained to 37 °C. The left jugular vein was catheterized and anesthesia was maintained with ketamine (20 mg/kg/hour) until the end of shock. The femoral artery was catheterized and the
catheter was connected to a pressure sensor and a blood pressure analyzer (IOX, EMKA technology) in order to monitor mean arterial blood pressure during all the procedure. Blood was
withdrawn through the femoral arterial line until the mean arterial blood pressure reached 35 mmHg. Blood was stored in 0.15 mL of saline with heparin. Mean arterial blood pressure was
maintained to 35 ± 5 mmHg for 2 h through successive blood withdrawals or replacements. At the end of that period, the blood previously stored and a Ringer's lactate solution (equal to
the initial blood volume) were infused to provide appropriate fluid resuscitation. Sham-operated animals underwent the same anesthetic and surgical procedures, but neither hemorrhage nor
fluid resuscitation was performed. RENAL FUNCTION At day 1, serum creatinine was measured on a Pentra 400 analyzer (HoribaMedical, Grabels, France) to provide an index of renal function. At
day 21, GFR was measured using urinary clearance of inulin43. After anesthesia (Sodium Thiobutabarbital, Inactin, Sigma T133, 150 mg/kg) and tracheotomy, the jugular vein was catheterized
for infusion of inulin (Cerb Laboratoire, France, 0.06 mg/min) and Gelofusine 4%. Another catheter was placed in the femoral artery for the monitoring of arterial blood pressure and
collection of blood samples. Finally, an intravesical catheter was inserted for urine collection. At the end of surgery, 4 mg of inulin were injected intravenously. After 30 min of
equilibration, urine was collected during one hour with a blood sample at mid-period. Plasma and urine inulin concentration were then measured. GFR was assessed by calculation of inulin
clearance. GENE EXPRESSION QUANTIFICATION Total RNA was isolated from mouse whole kidneys using Qiagen RN EasyPlus Mini kit (Qiagen, Valencia, CA). The sample RNA concentration was measured
on a NanoDrop instrument (ND-1000 Spectrophometer) and RNA purity was determined by the A260/A280 and A260/A230 ratios as described42. For RT-PCR, 500 ng of total RNA were used for cDNA
synthesis by Superscript II Reverse Transcriptase (Invitrogen, Life Technologies SAS). Biomark qPCR analysis was performed according to the manufacturer’s protocol (ADP37, Fluidigm, South
San Francisco, USA). Water was loaded with primers as negative control. Primers had been previously tested to assure efficacy and rule out genomic DNA cross-reactivity. Relative mRNA
expression levels were calculated using the ΔΔCtCt method, normalized to HPRT and β actin, and expressed as fold increases relative to the sham animals. PROTEIN EXPRESSION Tissue proteins
exctracts (10 μg) were separated by electrophoresis in polyacrylamide gels 10% SDS-PAGE gels (Invitrogen, Life Technologies SAS) and electrophoretically transferred to nitrocellulose
membranes (Hybond-ECL; Amersham). After 1-h incubation at room temperature in Tris-buffered saline, 5% milk, the blots were exposed to primary antibodies (Enzolife : caspase 1 mAb4844,
caspase 8 mAb1G1245, caspase 11mAb4E1146 ; 1:500 in a solution Tris-buffered saline, 5% milk for 1 night at 4 °C). The primary antibodies were revealed using the corresponding rabbit or
mouse peroxidase- conjugated secondary antibodies for 1 h at room temperature. Immunoreactivities were revealed with the ECL Western Blotting Reagent (Amersham). Quantifications were
performed with the operating system ChemiDoc (Biorad) and analyzed with the software ImageLab (Biorad). Densitometric values from murine caspases 1, 8 and 11 antibodies on kidney extracts
were normalized by the anti-β actin (Monoclonal Anti-β-Actin antibody produced in mouse, A5316, Sigma) signal intensity, and then normalized to sham. HISTOLOGIC ANALYSIS Kidneys were stored
in Carnoy solution (ethanol 60%, chloroform 30%, acetic acid 10%) for 48 h and then in 70% alcohol. They were dehydrated and embedded in paraffin and 4 µm paraffin-embedded tissue sections
were cut and used for immunohistochemistry and histological staining. TUNEL Cell death was studied through the TUNEL (Terminal Deixynucleotidyl Transferase dUTP Nick End Labelling) method
using Apoptotag Peroxidase Det Kit (S7100, Merkmillipore). Sections were scanned using a Nanozoomer 2.0 RS (Hamamatsu Photonics SARL, Massy, France) and quantification was performed manually
by counting nuclei in the cortex and cortico-medullary junction. Then the account was reported to the area of the studied field. IMMUNOHISTOCHEMISTRY FOR COLLAGEN III As previously
described42, after endogenous peroxidase blockade (S2001, DakoCytomation) tissue sections were incubated for 1 h with primary antibody anti-collagen type III (Rabbit anti Mouse/Rat Collagen
type III alpha 1 chain, Acris Antibodies GmbH) and then for 1 h with the secondary anti-rabbit IgG (Dako Envision HRP system). Immunological complexes were visualized by the addition of the
DAB substrate during 10 min (TA-125-HDX, Thermo Fisher Scientific). Sections were counterstained with hematoxylin. Sections were scanned using a Nanozoomer 2.0 RS (Hamamatsu Photonics SARL,
Massy, France) and treated with ImageJ analysis software for morphometric analyses. STATISTICAL ANALYSES Statistical comparisons used a Kruskall-Wallis with a Dunn post-hoc test, performed
using GraphPad Prism Version 6. Difference between groups were considered significant when _P_ < 0.05. Data were expressed as mean ± standard error of the mean (m ± s.e.m.). REFERENCES *
Nilsson, S. _et al._ Mechanisms of estrogen action. _Physiol. Rev._ 81, 1535–1565. https://doi.org/10.1152/physrev.2001.81.4.1535 (2001). Article CAS PubMed Google Scholar * Grimont, A.,
Bloch-Faure, M., El Abida, B. & Crambert, G. Mapping of sex hormone receptors and their modulators along the nephron of male and female mice. _FEBS Lett._ 583, 1644–1648.
https://doi.org/10.1016/j.febslet.2009.04.032 (2009). Article CAS PubMed Google Scholar * Burris, D. _et al._ Estrogen directly and specifically downregulates NaPi-IIa through the
activation of both estrogen receptor isoforms (ERalpha and ERbeta) in rat kidney proximal tubule. _Am. J. Physiol. Renal Physiol._ 308, F522-534. https://doi.org/10.1152/ajprenal.00386.2014
(2015). Article CAS PubMed PubMed Central Google Scholar * Cheema, M. U. _et al._ Estradiol regulates AQP2 expression in the collecting duct: a novel inhibitory role for estrogen
receptor alpha. _Am. J. Physiol. Renal Physiol._ 309, F305-317. https://doi.org/10.1152/ajprenal.00685.2014 (2015). Article CAS PubMed Google Scholar * Dignam, W. S., Voskian, J. &
Assali, N. S. Effects of estrogens on renal hemodynamics and excretion of electrolytes in human subjects. _J. Clin. Endocrinol. Metab._ 16, 1032–1042. https://doi.org/10.1210/jcem-16-8-1032
(1956). Article CAS PubMed Google Scholar * Potier, M. _et al._ Expression and regulation of estrogen receptors in mesangial cells: influence on matrix metalloproteinase-9. _J. Am. Soc,
Nephrol._ 12, 241–251 (2001). CAS Google Scholar * Cattran, D. C. _et al._ The impact of sex in primary glomerulonephritis. _Nephrol. Dial. Transplant_ 23, 2247–2253.
https://doi.org/10.1093/ndt/gfm919 (2008). Article PubMed Google Scholar * Neugarten, J., Acharya, A. & Silbiger, S. R. Effect of gender on the progression of nondiabetic renal
disease: a meta-analysis. _J. Am. Soc. Nephrol._ 11, 319–329 (2000). CAS PubMed Google Scholar * Neugarten, J. & Golestaneh, L. Gender and the prevalence and progression of renal
disease. _Adv. Chronic Kidney Dis._ 20, 390–395. https://doi.org/10.1053/j.ackd.2013.05.004 (2013). Article PubMed Google Scholar * Riazi, S., Maric, C. & Ecelbarger, C. A. 17-beta
Estradiol attenuates streptozotocin-induced diabetes and regulates the expression of renal sodium transporters. _Kidney Int._ 69, 471–480. https://doi.org/10.1038/sj.ki.5000140 (2006).
Article CAS PubMed Google Scholar * Ulas, M. & Cay, M. 17beta-Estradiol and vitamin E modulates oxidative stress-induced kidney toxicity in diabetic ovariectomized rat. _Biol. Trace
Elem. Res._ 144, 821–831. https://doi.org/10.1007/s12011-011-9025-x (2011). Article CAS PubMed Google Scholar * Trentzsch, H., Nienaber, U., Behnke, M., Lefering, R. & Piltz, S.
Female sex protects from organ failure and sepsis after major trauma haemorrhage. _Injury_ 45(Suppl 3), S20-28. https://doi.org/10.1016/j.injury.2014.08.013 (2014). Article PubMed Google
Scholar * Bosch, F., Angele, M. K. & Chaudry, I. H. Gender differences in trauma, shock and sepsis. _Mil. Med. Res._ 5, 35. https://doi.org/10.1186/s40779-018-0182-5 (2018). Article
PubMed PubMed Central Google Scholar * John, P. R. _et al._ An assessment of the impact of pregnancy on trauma mortality. _Surgery_ 149, 94–98. https://doi.org/10.1016/j.surg.2010.04.019
(2011). Article PubMed Google Scholar * Tsushida, K. _et al._ Estrogen-related receptor alpha is essential for maintaining mitochondrial integrity in cisplatin-induced acute kidney
injury. _Biochem. Biophys. Res. Commun._ https://doi.org/10.1016/j.bbrc.2018.03.080 (2018). Article PubMed Google Scholar * Hutchens, M. P., Fujiyoshi, T., Komers, R., Herson, P. S. &
Anderson, S. Estrogen protects renal endothelial barrier function from ischemia-reperfusion in vitro and in vivo. _Am. J. Physiol. Renal Physiol._ 303, F377-385.
https://doi.org/10.1152/ajprenal.00354.2011 (2012). Article CAS PubMed PubMed Central Google Scholar * Singh, A. P., Singh, N., Pathak, D. & Bedi, P. M. S. Estradiol attenuates
ischemia reperfusion-induced acute kidney injury through PPAR-gamma stimulated eNOS activation in rats. _Mol. Cell. Biochem._ https://doi.org/10.1007/s11010-018-3427-4 (2018). Article
PubMed Google Scholar * Hutchens, M. P. _et al._ Estrogen-mediated renoprotection following cardiac arrest and cardiopulmonary resuscitation is robust to GPR30 gene deletion. _PLoS ONE_ 9,
e99910. https://doi.org/10.1371/journal.pone.0099910 (2014). Article ADS CAS PubMed PubMed Central Google Scholar * Ikeda, M. _et al._ Estrogen administered after cardiac arrest and
cardiopulmonary resuscitation ameliorates acute kidney injury in a sex- and age-specific manner. _Crit. Care_ 19, 332. https://doi.org/10.1186/s13054-015-1049-8 (2015). Article PubMed
PubMed Central Google Scholar * Bouchard, J. _et al._ Fluid accumulation, survival and recovery of kidney function in critically ill patients with acute kidney injury. _Kidney Int._ 76,
422–427. https://doi.org/10.1038/ki.2009.159 (2009). Article PubMed Google Scholar * Charbonneau, H. _et al._ Acute bradykinin receptor blockade during hemorrhagic shock in mice prevents
the worsening hypotensive effect of angiotensin-converting enzyme inhibitor. _Crit. Care Med._ 44, e882-885. https://doi.org/10.1097/CCM.0000000000001646 (2016). Article CAS PubMed Google
Scholar * Dupuy, V. _et al._ Type 2 diabetes mellitus in mice aggravates the renal impact of hemorrhagic shock. _Shock_ 38, 351–355. https://doi.org/10.1097/SHK.0b013e318268810f (2012).
Article PubMed Google Scholar * Mayeur, N. _et al._ Morphologic and functional renal impact of acute kidney injury after prolonged hemorrhagic shock in mice. _Crit. Care Med._ 39,
2131–2138. https://doi.org/10.1097/CCM.0b013e31821f04f0 (2011). Article PubMed Google Scholar * Yang, J. R. _et al._ Ischemia-reperfusion induces renal tubule pyroptosis via the
CHOP-caspase-11 pathway. _Am. J. Physiol. Renal Physiol._ 306, F75-84. https://doi.org/10.1152/ajprenal.00117.2013 (2014). Article CAS PubMed Google Scholar * Vegeto, E. _et al._
Estrogen receptor-alpha mediates the brain anti inflammatory activity of estradiol. _Proc. Natl. Acad. Sci. U.S.A._ 100, 9614–9619. https://doi.org/10.1073/pnas.1531957100 (2003). Article
ADS CAS PubMed PubMed Central Google Scholar * Ahmed, S. B. & Ramesh, S. Sex hormones in women with kidney disease. _Nephrol. Dial. Transplant._ 31, 1787–1795.
https://doi.org/10.1093/ndt/gfw084 (2016). Article CAS PubMed Google Scholar * Ahmed, S. B. _et al._ Oral estrogen therapy in postmenopausal women is associated with loss of kidney
function. _Kidney Int._ 74, 370–376. https://doi.org/10.1038/ki.2008.205 (2008). Article CAS PubMed Google Scholar * Wu, C. C., Chang, C. Y., Chang, S. T. & Chen, S. H.
17beta-Estradiol accelerated renal tubule regeneration in male rats after ischemia/reperfusion-induced acute kidney injury. _Shock_ 46, 158–163. https://doi.org/10.1097/SHK.0000000000000586
(2016). Article CAS PubMed Google Scholar * Abbassi-Ghanavati, M., Greer, L. G. & Cunningham, F. G. Pregnancy and laboratory studies: a reference table for clinicians. _Obstet.
Gynecol._ 114, 1326–1331. https://doi.org/10.1097/AOG.0b013e3181c2bde8 (2009). Article CAS PubMed Google Scholar * Toutain, C. E. _et al._ Prevention of skin flap necrosis by estradiol
involves reperfusion of a protected vascular network. _Circ. Res._ 104, 245–254. https://doi.org/10.1161/CIRCRESAHA.108.182410 (2009). Article CAS PubMed Google Scholar * Meyer, M. R.,
Prossnitz, E. R. & Barton, M. The G protein-coupled estrogen receptor GPER/GPR30 as a regulator of cardiovascular function. _Vasc. Pharmacol._ 55, 17–25.
https://doi.org/10.1016/j.vph.2011.06.003 (2011). Article CAS Google Scholar * Hodis, H. N. _et al._ Vascular effects of early versus late postmenopausal treatment with estradiol. _N.
Engl. J. Med._ 374, 1221–1231. https://doi.org/10.1056/NEJMoa1505241 (2016). Article CAS PubMed PubMed Central Google Scholar * Lenfant, F., Tremollieres, F., Gourdy, P. & Arnal, J.
F. Timing of the vascular actions of estrogens in experimental and human studies: why protective early, and not when delayed?. _Maturitas_ 68, 165–173.
https://doi.org/10.1016/j.maturitas.2010.11.016 (2011). Article CAS PubMed Google Scholar * Tarhouni, K. _et al._ Determinants of flow-mediated outward remodeling in female rodents:
respective roles of age, estrogens, and timing. _Arterioscler. Thromb. Vasc. Biol._ 34, 1281–1289. https://doi.org/10.1161/ATVBAHA.114.303404 (2014). Article CAS PubMed Google Scholar *
Hubbard, W. _et al._ 17 alpha-ethynylestradiol-3-sulfate treatment of severe blood loss in rats. _J. Surg. Res._ 193, 355–360. https://doi.org/10.1016/j.jss.2014.06.047 (2015). Article CAS
PubMed Google Scholar * Tanaka, R. _et al._ Protective effect of 17beta-estradiol on ischemic acute kidney injury through the renal sympathetic nervous system. _Eur. J. Pharmacol._ 683,
270–275. https://doi.org/10.1016/j.ejphar.2012.02.044 (2012). Article CAS PubMed Google Scholar * Bergsbaken, T., Fink, S. L. & Cookson, B. T. Pyroptosis: host cell death and
inflammation. _Nat. Rev. Microbiol._ 7, 99–109. https://doi.org/10.1038/nrmicro2070 (2009). Article CAS PubMed PubMed Central Google Scholar * Miao, E. A., Rajan, J. V. & Aderem, A.
Caspase-1-induced pyroptotic cell death. _Immunol. Rev._ 243, 206–214. https://doi.org/10.1111/j.1600-065X.2011.01044.x (2011). Article CAS PubMed PubMed Central Google Scholar *
Anders, H. J. Toll-like receptors and danger signaling in kidney injury. _J. Am. Soc. Nephrol._ 21, 1270–1274. https://doi.org/10.1681/ASN.2010030233 (2010). Article CAS PubMed Google
Scholar * Rock, K. L., Latz, E., Ontiveros, F. & Kono, H. The sterile inflammatory response. _Annu. Rev. Immunol._ 28, 321–342. https://doi.org/10.1146/annurev-immunol-030409-101311
(2010). Article CAS PubMed Google Scholar * Heldring, N. _et al._ Estrogen receptors: how do they signal and what are their targets. _Physiol. Rev._ 87, 905–931.
https://doi.org/10.1152/physrev.00026.2006 (2007). Article CAS PubMed Google Scholar * Belliere, J. _et al._ Specific macrophage subtypes influence the progression of
rhabdomyolysis-induced kidney injury. _J. Am. Soc. Nephrol._ 26, 1363–1377. https://doi.org/10.1681/ASN.2014040320 (2015). Article CAS PubMed Google Scholar * Buleon, M. _et al._
Pharmacological blockade of B2-kinin receptor reduces renal protective effect of angiotensin-converting enzyme inhibition in db/db mice model. _Am. J. Physiol. Renal Physiol._ 294,
F1249-1256. https://doi.org/10.1152/ajprenal.00501.2007 (2008). Article CAS PubMed Google Scholar * Xiao, N. _et al._ Ginsenoside Rg5 inhibits succinate-associated lipolysis in adipose
tissue and prevents muscle insulin resistance. _Front. Pharmacol._ 8, 43. https://doi.org/10.3389/fphar.2017.00043 (2017). Article CAS PubMed Google Scholar * Mandal, P. _et al._
Caspase-8 collaborates with caspase-11 to drive tissue damage and execution of endotoxic shock. _Immunity_ 49, 42–55. https://doi.org/10.1016/j.immuni.2018.06.011 (2018). Article CAS
PubMed PubMed Central Google Scholar * van Delft, M. F., Smith, D. P., Lahoud, M. H., Huang, D. C. & Adams, J. M. Apoptosis and non-inflammatory phagocytosis can be induced by
mitochondrial damage without caspases. _Cell Death Differ._ 17, 821–832. https://doi.org/10.1038/cdd.2009.166 (2010). Article CAS PubMed Google Scholar Download references FUNDING This
work was supported by the French National Institute for Health and Medical Research (INSERM). AUTHOR INFORMATION Author notes * These authors contributed equally: Marie Buléon and Mélodie
Cuny. AUTHORS AND AFFILIATIONS * Institute of Cardiovascular and Metabolic Disease, Institut National de la Santé et de la Recherche Médicale (INSERM), U1048, Toulouse, France Marie Buléon,
Mélodie Cuny, Jimmy Grellier, Pierre-Yves Charles, Julie Belliere, Audrey Casemayou, Jean-François Arnal, Joost-Peter Schanstra & Ivan Tack * Université Toulouse III Paul-Sabatier,
Toulouse, France Marie Buléon, Mélodie Cuny, Jimmy Grellier, Pierre-Yves Charles, Julie Belliere, Audrey Casemayou, Jean-François Arnal, Joost-Peter Schanstra & Ivan Tack * Service des
Explorations Fonctionnelles Physiologiques, CHU de Toulouse, 1 avenue Jean Poulhès, 31 059, Toulouse Cedex 9, France Pierre-Yves Charles, Jean-François Arnal & Ivan Tack Authors * Marie
Buléon View author publications You can also search for this author inPubMed Google Scholar * Mélodie Cuny View author publications You can also search for this author inPubMed Google
Scholar * Jimmy Grellier View author publications You can also search for this author inPubMed Google Scholar * Pierre-Yves Charles View author publications You can also search for this
author inPubMed Google Scholar * Julie Belliere View author publications You can also search for this author inPubMed Google Scholar * Audrey Casemayou View author publications You can also
search for this author inPubMed Google Scholar * Jean-François Arnal View author publications You can also search for this author inPubMed Google Scholar * Joost-Peter Schanstra View author
publications You can also search for this author inPubMed Google Scholar * Ivan Tack View author publications You can also search for this author inPubMed Google Scholar CONTRIBUTIONS I.T.,
J-F.A and J-P.S designed the study; M.B., M.C., J.G., P-Y.C., J.B. and A.C. performed the experiments; M.B., M.C. and I.T. analyzed and interpreted results, and wrote the manuscript. All
authors read and approved the final manuscript. CORRESPONDING AUTHOR Correspondence to Ivan Tack. ETHICS DECLARATIONS COMPETING INTERESTS The authors declare no competing interests.
ADDITIONAL INFORMATION PUBLISHER'S NOTE Springer Nature remains neutral with regard to jurisdictional claims in published maps and institutional affiliations. SUPPLEMENTARY INFORMATION
SUPPLEMENTARY INFORMATION RIGHTS AND PERMISSIONS OPEN ACCESS This article is licensed under a Creative Commons Attribution 4.0 International License, which permits use, sharing, adaptation,
distribution and reproduction in any medium or format, as long as you give appropriate credit to the original author(s) and the source, provide a link to the Creative Commons licence, and
indicate if changes were made. The images or other third party material in this article are included in the article's Creative Commons licence, unless indicated otherwise in a credit
line to the material. If material is not included in the article's Creative Commons licence and your intended use is not permitted by statutory regulation or exceeds the permitted use,
you will need to obtain permission directly from the copyright holder. To view a copy of this licence, visit http://creativecommons.org/licenses/by/4.0/. Reprints and permissions ABOUT THIS
ARTICLE CITE THIS ARTICLE Buléon, M., Cuny, M., Grellier, J. _et al._ A single dose of estrogen during hemorrhagic shock protects against Kidney Injury whereas estrogen restoration in
ovariectomized mice is ineffective. _Sci Rep_ 10, 17240 (2020). https://doi.org/10.1038/s41598-020-73974-5 Download citation * Received: 15 November 2019 * Accepted: 02 June 2020 *
Published: 14 October 2020 * DOI: https://doi.org/10.1038/s41598-020-73974-5 SHARE THIS ARTICLE Anyone you share the following link with will be able to read this content: Get shareable link
Sorry, a shareable link is not currently available for this article. Copy to clipboard Provided by the Springer Nature SharedIt content-sharing initiative