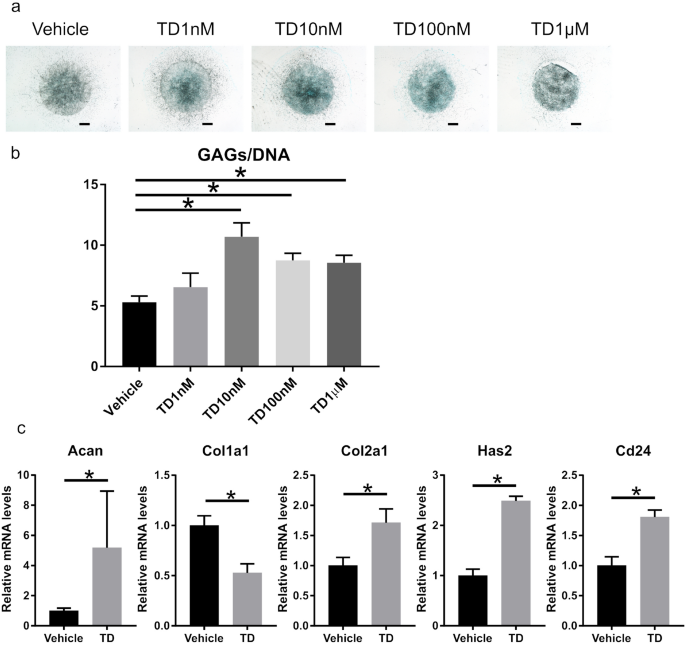
- Select a language for the TTS:
- UK English Female
- UK English Male
- US English Female
- US English Male
- Australian Female
- Australian Male
- Language selected: (auto detect) - EN
Play all audios:
ABSTRACT Degeneration of the nucleus pulposus (NP) might serve as a trigger for intervertebral disc degeneration (IDD). A recent drug screening study revealed that the thienoindazole
derivative, TD-198946, is a novel drug for the treatment of osteoarthritis. Because of the environmental and functional similarities between articular cartilage and intervertebral disc,
TD-198946 is expected to prevent IDD. Herein, we sought to evaluate the effects of TD-198946 on IDD. TD-198946 enhanced glycosaminoglycan (GAG) production and the related genes in mouse NP
cells and human NP cells (hNPCs). Further, Kyoto Encyclopedia of Genes and Genomes pathway analysis using the mRNA sequence of hNPCs suggested that the mechanism of action of TD-198946
primarily occurred via the phosphoinositide 3-kinase (PI3K)/Akt signaling pathway. The Akt inhibitor suppressed the enhancement of GAG production induced by TD-198946. The effects of
TD-198946 on IDD at two different time points (immediate treatment model, immediately after the puncture; latent treatment model, 2 weeks after the puncture) were investigated using a mouse
tail-disc puncture model. At both time points, TD-198946 prevented a loss in disc height. Histological analysis also demonstrated the preservation of the NP structures. TD-198946 exhibited
therapeutic effects on IDD by enhancing GAG production via PI3K/Akt signaling. SIMILAR CONTENT BEING VIEWED BY OTHERS EFFICACY OF NARINGENIN AGAINST AGING AND DEGENERATION OF NUCLEUS
PULPOSUS CELLS THROUGH IGFBP3 INHIBITION Article Open access 25 February 2025 PROGRESS IN UNDERSTANDING THE ROLE OF CGAS-STING PATHWAY ASSOCIATED WITH PROGRAMMED CELL DEATH IN INTERVERTEBRAL
DISC DEGENERATION Article Open access 16 October 2023 OCIFISERTIB ALLEVIATES THE GASDERMIN D-INDEPENDENT PYROPTOSIS OF NUCLEUS PULPOSUS CELLS BY TARGETING GSDME Article Open access 17 April
2025 INTRODUCTION Low back pain (LBP) is a common cause of disability and thus acts as a distinct societal and economic burden. Although several factors contribute to the severity or
persistence of LBP, intervertebral disc degeneration (IDD) is considered to be one of its leading causes1,2,3. The intervertebral disc consists of a central gel-like nucleus pulposus (NP),
an outer annulus fibrosus (AF), and superior and inferior cartilaginous endplates. Among them, NP plays a crucial role in the maintenance of homeostasis of the intervertebral disc via the
production of the extracellular matrix (ECM). Aging or mechanical/chemical stress, which disrupts the anabolic and catabolic balance, results in the deterioration of the ECM. Deterioration
in NP causes a loss in disc height and structural wear of the intervertebral disc. Further, it promotes IDD4,5. Therefore, treating NP degeneration has garnered increased attention.
Previously, regenerative approaches for degenerated NP, including growth factors6,7, stem cells8, and synthetic biomaterials9, have been reported. Although these therapies are promising,
their high costs and safety concerns might prevent their widespread use. Small compounds can be employed as an alternative treatment option for common diseases such as IDD and may contribute
to lowering cost and safety concerns. Recently, a drug screening study using the COL2-GFP-ATDC5 monitoring system revealed that of the 2,500 natural and synthetic small compounds
identified, the thienoindazole derivative, TD-198946, is a novel option for early osteoarthritis (OA). In a mouse OA model, the administration of TD-198946 into the joint space prevented the
degeneration of the articular cartilage. Further, TD-198946 was found to induce the production of ECM proteins, such as glycosaminoglycan (GAG) and type 2 collagen from chondrocytes10. Both
chondrocytes and NP cells are located in a hypoxic avascular environment and secrete ECM components to attain the hydration required to resist compressive loads11. Considering the
environmental and functional similarities between chondrocytes and NP cells, we hypothesized that TD-198946 could be administered to treat IDD. However, NP and cartilage are significantly
different in terms of anatomical structure, GAG composition, and some phenotypic markers5,12. Therefore, the effects of TD-198946 on NP cells need to be investigated independently. In the
present study, we aimed to investigate the effects of TD-198946 on NP cells, elucidate its mechanism of action in vitro, and evaluate its treatment potential on IDD using a
tail-puncture-induced mouse IDD model. RESULTS TD-198946 INCREASED GAG SYNTHESIS IN MOUSE NP CELLS (MNPCS) First, we evaluated the effects of TD-198946 on mNPCs in vitro. TD-198946 enhanced
the alcian blue staining of mNPCs at a concentration of 10 nM (Fig. 1a). Based on the GAG quantification assay, TD-198946 enhanced GAG synthesis in mNPCs. Further, the most substantial
effect was observed at a concentration of 10 nM (Fig. 1b). Gene expression analysis revealed that TD-198946 (10 nM) enhanced the expression of ECM synthesis genes (Acan, Has2, and Col2a1).
TD-198946 also enhanced the expression of Cd24, which has been reported as an NP-specific marker5,13,14,15 (Fig. 1c). Thus, the effects of TD-198946 on mNPCs for GAG synthesis were
demonstrated. TD-198946 ENHANCED GLYCOSAMINOGLYCAN SYNTHESIS IN HUMAN NP CELLS (HNPCS) We examined the effects of TD-198946 on hNPCs for future clinical applications. Consistent with the
results in mNPCs, TD-198946 enhanced GAG synthesis in human NP cells (the highest effect achieved with 100 nM) (Fig. 2a,b). Although TD-198946 (10 nM) increased the expression of ACAN and
HAS2, it did not affect the expression of COL2A1 and CD24 (Fig. 2c). Thus, we revealed the effects of TD-198946 on hNPCs for GAG synthesis. PHOSPHOINOSITIDE 3-KINASE (PI3K)/AKT SIGNALING
PATHWAY WAS INVOLVED IN THE EFFECT OF TD-198946 To elucidate the mechanism of action of TD-198946, we performed an mRNA sequencing assay to search the possible intracellular signaling
pathways that were most affected by the administration of TD-198946. Most differentially expressed genes (DEGs) identified by Kyoto Encyclopedia of Genes and Genomes (KEGG) pathway analysis
were involved in the PI3K/Akt signaling pathway, which was the most altered pathway by TD-198946 (Supplementary Fig. 1). To further evaluate the mechanism of action, we performed western
blotting and Akt inhibitor analysis using three donor samples, one of which was obtained after RNA-seq analysis. In western blotting, the expression levels of PI3K and phospho-Akt were
increased by the administration of TD-198946, whereas the expression level of phospho-Erk1/2 was not affected (Fig. 3a, Supplementary Fig. 2). We proceeded to determine whether the
inhibition of PI3K/Akt signaling attenuates the effects of TD-19894 using the Akt inhibitor, MK-2206. A previous study reported that significant cell toxicity owing to MK-2206 occurs at
concentrations ≥ 5 μM16. Thus, we added 10 nM–1 μM of MK-2206 to the TD-198946 (10 nM) supplemented medium. Akt was successfully inhibited by the decreased expression of phospho-Akt in the
hNPCs cultured with TD-198946 (10 nM) and MK-2206 (100 nM) (Fig. 3b, Supplementary Fig. 3). The enhanced synthesis of GAG by TD was dose-dependently decreased by MK-2206 (Fig. 3c). Further,
the increased expression of ACAN and HAS2 by TD-198946 (10 nM) was decreased by the addition of MK-2206 (100 nM) (Fig. 3d). There was no significant difference in the expression of COL2A1
between groups. Collectively, these findings indicate that the PI3K/Akt signaling pathway is involved in the effect of TD-198946. TD-198946 TREATED THE IMMEDIATE PUNCTURE-INDUCED IDD IN VIVO
We examined whether TD-198946 could treat the immediate occurrence of IDD using a mouse tail-puncture induced IDD model (Fig. 4a,b). TD-198946 attenuated the loss in tail-puncture-induced
disc height as measured by the % disc height index (DHI) at every time point (Fig. 4c, Supplementary Fig. 4a). The histology of the vehicle group highlighted the honeycomb appearance of the
NP owing to the loss of NP cells at 2 weeks (Fig. 4e, Supplementary Fig. 5). Most NP cells disappeared until 4 weeks after the puncture. Six weeks after the puncture, NP tissue was lost and
later replaced with fibrous tissue. However, in the TD-198946 group, the NP structures were well maintained, with abundant ECM until 6 weeks after drug injection; this occurred despite the
decrease in NP cells with time. The histological score in the TD-198946 group was higher than that in the vehicle group at every time point (Fig. 4d). These findings suggest that TD-198946
prevented the occurrence of IDD in this prevention model. TD-198946 ATTENUATED THE PROGRESSION OF MILD IDD IN VIVO We sought to determine whether TD-198946 could treat mild IDD by employing
a mild mouse IDD model. Briefly, the tail of the mouse was punctured 2 weeks before TD-198946 injection (Fig. 5a). In the vehicle group, the %DHI decreased over time during the 6-week
observation period. However, in the TD-198946 group, the %DHI was maintained for 6 weeks. Additionally, this group had a higher %DHI than the vehicle group after injection at every time
point (Fig. 5b, Supplementary Fig. 4b). The TD-198946 group had a higher histological score at every time point than the vehicle group (Fig. 5c). In the vehicle group, the number of NP cells
significantly decreased at 2 weeks (4 weeks after puncture) and there were no NP cells at 6 weeks (8 weeks after puncture) (Fig. 5d, Supplementary Fig. 6). In the TD-198946 group, the
number of NP cells in the NP decreased; however, they remained inside the ECM until 6 weeks. These findings suggest that TD-198946 could attenuate the progression of mild IDD. DISCUSSION In
this study, we demonstrated that TD-198946 enhanced the production of GAG in NP cells via the PI3K/AKT signaling pathway. Furthermore, administering TD-198946 into the intervertebral disc of
a mouse model could attenuate the progression of IDD induced by needle puncture. Our previous studies revealed that TD-198946 enhances GAG synthesis in mouse chondrocytes, human bone marrow
stromal cells, and human synovium-derived stem cells (hSSCs)10,17,18. Moreover, our previous study identified a unique effect of TD-198946 on hyaluronan secretion by hSSCs17. Consistent
with previous studies, TD-198946 was found to enhance the gene expression of aggrecan and hyaluronan synthase 2, resulting in increased GAG synthesis in both mNPCs and hNPCs of all donors.
Therefore, we concluded that the main effect of TD-198946 was the enhancement of the production of GAG. This increased production of GAGs might play a significant role in the preservation of
disc height in the mouse IDD model. Chondrocytes can synthesize GAGs and type 2 collagen; however, the ECM of NP can be distinguished from that of cartilage by the predominance of GAG in
the ECM of NP. Furthermore, despite the 3:1 ratio of GAGs:hydroxyproline in juvenile human cartilage, which decreases to 2:1 with age, a much higher ratio is found at all ages in hNPCs. The
ratio of juvenile hNPCs is 25:1, whereas that of young adult hNPCs is 27:1; the latter, however, decreases to 5:1 with age. The increase in GAG synthesis in NP cells owing to TD-198946 is
favorable for the predominant GAG feature in the ECM of NP. The effect of TD-198946 on the expression of COL2A1 in human NP cells was not significant. One possible explanation is the
difference in degeneration between mNPCs and hNPCs. The mNPCs were isolated from young, healthy NP tissue under a microscope, whereas the hNPCs were isolated from excised NP tissue during
lumbar disc herniation surgery. The hNPCs from surgically treated patients for disc disease in this study were considered to be more degenerated than mNPCs, and the degree of degeneration
should be different among human donors. Another explanation is that the increase in the expression of Col2a1 by TD-198946 was milder than that of Acan or Has2 even in mNPCs. Moreover, our
previous study using hSSCs also revealed that TD-198946 enhances GAG production but does not affect the expression of COL2A1 in hSSCs17. Thus, we considered that the expression of COL2A1 is
not a direct target of TD-198946. TD-198946 synergistically increases the expression of COL2A1 when combined with transforming growth factor-β3 or bone morphogenetic protein-217,19. Further
research is needed to explore the optimal combinations of TD-198946 and growth factors that enhance the expression of COL2A1 in hNPCs. This study indicates that the PI3K/Akt signaling
pathway is involved in the effect of TD-198946, although further research is needed to elucidate the precise mechanism of action by TD-198946. The activation of the PI3K/Akt pathway has been
reported to protect against IDD through different mechanisms, such as the increase in ECM content, prevention of apoptosis, facilitation of cell proliferation, induction or prevention of
autophagy, alleviation of oxidative damage, and adaptation to a hypoxic microenvironment20,21,22,23. The activation of the PI3K/Akt signaling may affect ECM content by enhancing the
transcription of aggrecan in rat NP cells24. In this study, the administration of TD-198946 enhanced the PI3K/Akt signaling pathway in NP cells and increased the expression of aggrecan and
hyaluronan synthase 2. After the puncture of the disc, the number of NP cells decreased following treatment with TD-198946. However, the increased production of GAG in NP cells was assumed
to contribute to the maintenance of the intervertebral disc height and NP structure (Fig. 6). This study had several limitations. First, only a few human donors were enrolled, most of whom
were young. Therefore, further research with more human donors is needed to evaluate the efficacy of TD-198946 in NP cells of older generations. Second, the hNPCs in this study were obtained
surgically and were more degenerated than mNPCs. The inconsistency of the response to TD-198946 may be attributable to these differences. However, the increased expression of ACAN and HAS
and the enhanced GAG synthesis in hNPCs of all donors by TD-198946 suggest that TD-198946 is a candidate therapeutic molecule for IDD. Third, the length of time spent by TD-198946 inside the
intervertebral disc space after intra-disc injection was not measured. Because frequent intra-disc injection might accelerate IDD, only one intra-disc injection was administered. Further,
as the fibrous annulus fibrosus and hypoperfusion surround NP owing to the avascular, TD-198946 was expected to remain in the space for a certain amount of time. A further study is thus
required to trace the length of time spent by TD-198946 inside the disc space. Finally, the biomechanical environment of the mouse coccygeal vertebrae differs from that of the human spine.
Thus, a pre-clinical animal model whose spine has a similar biomechanical environment to the human spine is desired for future clinical application. In conclusion, the small compound,
TD-198946, increased the ability of NP cells to produce GAGs via the PI3K/Akt signaling pathway and protected against early IDD. METHODS REAGENTS Herein, the same lot of TD-198946 employed
in our previous study17 was utilized for all experiments. TD-198946 was dissolved in dimethyl sulfoxide (DMSO, Sigma-Aldrich, St. Louis, MO) and diluted in phosphate-buffered saline (PBS)
(1:1,000) for the in vivo analysis, and culture medium (1:1,000) for the in vitro analysis. DMSO diluted in PBS (1:1,000) and the culture medium (1:1,000) were respectively used as the
vehicle in the in vivo and in vitro analyses. ISOLATION OF MOUSE NP CELLS AND HUMAN NP CELLS Male C57BL6J mice (age, 10–12 weeks) were purchased from Oriental Yeast Co., Ltd. (Tokyo, Japan).
mNPCs were cultured as described previously25. With this method, NP cells from five mice were required to prepare one set of NP cells to perform in vitro experiments. Briefly, mouse NP
tissues were digested with 0.1% pronase (Roche, Indianapolis, IN) at 37 °C for 30 min and 0.2% collagenase type 2 (Worthington, Lakewood, NJ) at 37 °C for 2 h. After digestion, the mNPCs
were cultured in 3D collagen gel (Cellmatrix type I-A, Nitta Gelatin, Osaka, Japan) in Dulbecco’s Modified Eagle’s Medium (DMEM, Nacalai Tesque, Kyoto, Japan) with 10% fetal bovine serum
(FBS, Thermo Fisher Scientific, Waltham, MA) and 1% antibiotic/antimycotic solution (A/A, Sigma-Aldrich) under a 5% CO2 and 5% oxygen atmosphere at 37 °C. After primary 3D collagen gel
culture, the gel was digested, and the mNPCs were cultured in a 2D monolayer in expansion medium (DMEM with 10% FBS and 1% A/A, with 10 ng/mL of bFGF [recombinant human basic fibroblast
growth factor, Fujifilm Wako, Osaka, Japan]). Low-passage cells (passage 2) were used for all experiments. We prepared three sets of NP cells from 15 mice, and all experiments were performed
using each set of NP cells. After the retrieval of informed consent from patients, human NP tissue was isolated from three donors during nucleotomy surgery for lumbar intervertebral disc
herniation. The gender, age, and disc levels of the donors are listed in Supplementary Table 1. The tissues were digested with 0.1% pronase at 37 °C for 30 min and 0.2% collagenase type 2 at
37 °C overnight. After digestion, the isolated hNPCs were cultured as a monolayer in expansion medium under a 5% CO2 and 5% oxygen atmosphere at 37 °C. Low-passage cells (passage 1) were
used for all experiments. TWO-DIMENSIONAL MICROMASS CULTURE Two-dimensional micromass culture was performed as described previously17,25. Briefly, a high concentration of mNPCs or hNPCs was
cultured in micromass (1 × 105 cells/10 μL) in chondrogenic basal medium (DMEM with 1% ITS [insulin, transferrin, selenium, Corning Inc., Corning, NY], 50 μg/mL ascorbic acid
[Sigma-Aldrich], 40 μg/mL L-proline [Fujifilm Wako], 1% FBS, and 1% A/A) supplemented with vehicle or TD-198946 (1 nM to 1 μM) for 7 days. The medium was changed twice per week. ALCIAN BLUE
STAINING Both mNPCs and hNPCs were fixed with 4% paraformaldehyde, stained with alcian blue (pH 1.0) for 3 h, and washed with distilled water. SULFATED GAG QUANTIFICATION The micromass of
the mNPCs or the hNPCs was first digested with 0.05% papain (Sigma‐Aldrich) for 3 h at 65 °C with shaking. Thereafter, the sulfated GAG content was measured by a dimethylmethylene blue
dye‐binding assay (Blyscan Glycosaminoglycan Assay Kit, Biocolor, Westbury, NY) with a kit containing chondroitin sulfate as the standard. Cellularity was measured according to the
double‐stranded DNA (dsDNA) content using a Qubit 3.0 Fluorometer (Thermo Fisher Scientific) and the Qubit dsDNA HS Assay kit (Thermo Fisher Scientific). REAL-TIME PCR ASSAY The mNPCs or
hNPCs were homogenized in TRIzol Reagent (Invitrogen, Carlsbad, CA). Total RNA was extracted using the Direct-zol RNA kit (Zymo Research, Orange, CA). Then, the total RNA was converted to
cDNA using ReverTra Ace qPCR RT Master Mix (Toyobo, Osaka, Japan). Gene expression was measured using quantitative real-time PCR with SYBR green master mix (Thermo Fisher Scientific) for
mNPCs and TaqMan Fast Advanced Master Mix (Thermo Fisher Scientific) for hNPCs on the Step One Plus Real-Time PCR System (Applied Biosystems, Foster City, CA). The expression of the ECM
synthesis genes (Acan, Has2, Col1a1, and Col2a1), the NP-specific marker (Cd24)5,13,14,15, and the endogenous gene (Gapdh) was evaluated. The primer sequences used for real-time PCR are
listed in Supplementary Tables 2 and 3. The mRNA levels were calculated using the relative quantitation standard curve method and normalized to the level of Gapdh in each sample. RNA
SEQUENCING AND PATHWAY ANALYSIS After total RNA was extracted from hNPCs (Donors 1 and 2) cultured with the vehicle or TD-198946 (10 nM) for 7 days, an mRNA sequencing analysis was performed
at BGI Tech Solutions Co., Ltd. (Hong Kong) using the BGISEQ-500 platform. A transcript library was constructed for all samples, and all transcripts were sequenced with the 50-bp single-end
sequencing technology. The differential analysis compared the differential transcripts between the control and the TD-198946 group. DEGs between the groups were detected with DEseq2 by BGI
Tech Solutions. DEseq2 is based on the negative binomial distribution performed as described previously26. The false discovery rate (FDR) for each P value was calculated. Generally, the
terms with an FDR less than 0.01 were defined as significantly enriched. The P value cut-off was set at 0.05. A fold change ≥ 2.00 or ≤ 0.50 and an adjusted P value ≤ 0.05 were considered to
indicate significance. KEGG pathway analysis was performed to identify significant DEGs27,28,29,30. Using the KEGG annotation result, DEGs were classified according to the official
classification to determine the number of DEGs in the most enriched pathway. WESTERN BLOTTING The hNPCs were cultured with serum-free DMEM for 24 h. After serum starvation, the hNPCs were
cultured with DMEM supplemented with the vehicle or TD-198946 (10 nM) for 60 min. Thereafter, the cells were washed with PBS and lysed in RIPA buffer (Nacalai Tesque) containing a
protease/phosphatase inhibitor cocktail (Cell Signaling Technology, Danvers, MA). The total protein was sonicated, centrifuged at 12,000 rpm for 5 min at 4 °C, and quantified using the
Pierce Rapid Gold BCA Protein Assay Kit (Thermo Fisher Scientific). Equal amounts of protein were separated on Bolt Bis–Tris Plus, 4–12% precast polyacrylamide gels (Thermo Fisher
Scientific). Fractionated proteins were transferred onto a PVDF membrane using the Mini Blot Module (Thermo Fisher Scientific). After 1 h of blocking with Phospho-blocker (Cell Biolabs Inc.,
San Diego, CA), the membrane was probed overnight at 4 °C with a primary antibody followed by 1 h at room temperature with anti-rabbit IgG HRP-linked antibody (7,074, Cell Signaling
Technology). Immunodetection was performed with the Amersham ECL Prime western blotting detection reagent (GE Healthcare, UK). The membrane was visualized using MF-ChemiBIS 3.2 (DNR
Bio-Imaging Systems Ltd., Israel). The primary antibodies used for western blot analysis are listed in Supplementary Table 4. The bands were quantified using Image J software (National
Institutes of Health, Bethesda, MD). β-Actin was used as the loading control for internal correction. AKT INHIBITOR ANALYSIS The Akt-inhibitor, MK-2206 (Chemscene, Monmouth Junction, NJ),
was used. The hNPCs were cultured in micromass in chondrogenic basal medium supplemented with TD-198946 (10 nM) and MK-2206 (10 nM to 1 μM) for seven days for alcian blue staining and
real-time PCR. The hNPCs were also cultured with DMEM supplemented with TD-198946 (10 nM) and MK-2206 (100 nM) (TD + MK2206) for 60 min for western blot analysis. MICE Male C57BL6J mice
(age, 10–12 weeks) were purchased from Oriental Yeast Co., LTD. (Tokyo, Japan) and maintained under standard animal housing conditions (12 h light–12 h dark cycle and free access to food and
water). All mice were maintained under specific-pathogen-free conditions and handled according to the guidelines of the Institutional Animal Care and Use Committee of Osaka University
Graduate School of Medicine. IDD ANIMAL MODEL The mouse IDD model by AF puncture using a 33G needle has been established7,31. The AF puncture using a 33G needle can lead to a mild IDD. Thus,
the 33G needle was recommended for the study of intervertebral disc regeneration. Briefly, under general anesthesia, the segments of tail intervertebral discs were shown by high-resolution
micro-computed tomography (CT) (Rigaku, Tokyo, Japan) at 90 kV and 160 mA. Micro CT was performed using a fixed metal marker on the tail side and skin marker at the puncture level, which was
created based on the relative positional relation between the metal marker and the disc levels. Then, the discs (Co3-Co4 segment) were punctured percutaneously with a 33-gauge needle
(Neuros Syringes, Hamilton, Reno, NV) through the annulus fibrosus to the depth of the needle stopper (1 mm) from the dorsal to the ventral side. Two experimental models (immediate and
latent treatment models) were established according to the timing of the puncture and injection. In the immediate treatment model, 5 μL of either the vehicle or TD-198946 (100 nM) was
injected into the disc using the Neuros Syringe at the same time as the tail disc puncture (n = 8 for each group, total puncture number = 1). In the latent treatment model, the drugs were
injected into the disc using the Neuros Syringe at 2 weeks following the puncture (n = 6 for each group, total puncture number = 2). The injection was performed slowly over 1 min to prevent
the acute increase in internal pressure of the disc. After the injection, the needle was held for another 1 min to promote perfusion of the solution into the tissue and prevent the leakage
from the disc, as previously described7,32. RADIOGRAPHIC ASSESSMENT OF THE DISC HEIGHT A high-resolution micro-computed tomography was used to scan the tails at 90 kV and 160 mA. The DHI was
measured using Image J software) using a previously reported method33. The disc height and two adjacent vertebral body heights were obtained as averages of the anterior, middle, and
posterior portions. DHI was calculated by dividing disc height by the average of the two adjacent vertebral body heights and multiplying the result by 100. The change in DHI was expressed as
%DHI (post-injection DHI/pre-injection DHI). HISTOLOGICAL ANALYSIS The intervertebral discs were fixed in 4% paraformaldehyde in PBS and decalcified with 20% EDTA until soft and pliable.
Thereafter, the intervertebral discs were dehydrated using serial ethanol and cleared in xylene. After the samples were embedded in paraffin wax, sections were created at the central part of
the intervertebral disc using the shape of the NP, AF, and epiphyseal plate as the indices. However, it was technically challenging to create histological images of all samples at precisely
the same depth. Sections were cut 3-μm-thick and stained with hematoxylin and eosin (H&E) and Safranin-O fast green (SO) according to the standard protocol. IDD generated via the tail
puncture method was quantified with a histological grading score as described previously34. Briefly, the grading score of IDD is based on the morphological features of the NP, AF, and NP/AF
boundary, with higher scores representing higher levels of degenerative changes (from 0 [standard] to 14 [severely degenerated]). STATISTICAL ANALYSIS GraphPad Prism 7 (GraphPad Inc., La
Jolla, CA) was used for the analysis. Differences in the measured variables between the groups were analyzed using the Student’s _t_ test or Mann–Whitney U test, as appropriate. Differences
in the measured variables between multiple groups were analyzed using one-way ANOVA followed by the Dunnett test or the Bonferroni test. Differences with a p value < 0.05 were considered
significant. ETHICS STATEMENT The Ethical Review Board of Osaka University Hospital approved the experiments performed with human tissue (No. 19423). Written informed consent was obtained
from each patient and/or from either the parent or the legally authorized representative of the patient under eighteen years old according to the principles of the Declaration of Helsinki
and the laws and regulations of Japan. The Animal Experimental Committee of Osaka University Graduate School of Medicine approved all animal studies (No. 280038). All methods were performed
per relevant laboratory guidelines and regulations. DATA AVAILABILITY The datasets generated during and/or analyzed during the current study are available from the corresponding authors on
reasonable request. REFERENCES * Risbud, M. V. & Shapiro, I. M. Role of cytokines in intervertebral disc degeneration: Pain and disc content. _Nat. Rev. Rheumatol._ 10, 44–56 (2014).
Article CAS Google Scholar * Martin, B. I. _et al._ Expenditures and health status among adults with back and neck problems. _JAMA_ 299, 656–664 (2008). Article CAS Google Scholar *
Hoy, D. _et al._ A systematic review of the global prevalence of low back pain. _Arthritis Rheum._ 64, 2028–2037 (2012). Article Google Scholar * Sakai, D. & Andersson, G. B. Stem cell
therapy for intervertebral disc regeneration: obstacles and solutions. _Nat. Rev. Rheumatol._ 11, 243–256 (2015). Article Google Scholar * Risbud, M. V. _et al._ Defining the phenotype of
young healthy nucleus pulposus cells: Recommendations of the Spine Research Interest Group at the 2014 annual ORS meeting. _J. Orthop. Res._ 33, 283–293 (2015). Article Google Scholar *
Paglia, D. N., Singh, H., Karukonda, T., Drissi, H. & Moss, I. L. PDGF-BB delays degeneration of the intervertebral discs in a rabbit preclinical model. _Spine (Phila Pa 1976)_ 41,
E449-458 (2016). Article Google Scholar * Liang, H., Ma, S. Y., Feng, G., Shen, F. H. & Joshua, L. X. Therapeutic effects of adenovirus-mediated growth and differentiation factor-5 in
a mice disc degeneration model induced by annulus needle puncture. _Spine J._ 10, 32–41 (2010). Article Google Scholar * Ishiguro, H. _et al._ Intervertebral disc regeneration with an
adipose mesenchymal stem cell-derived tissue-engineered construct in a rat nucleotomy model. _Acta Biomater._ 87, 118–129 (2019). Article CAS Google Scholar * Sun, Z. _et al._ Effect of
perfluorotributylamine-enriched alginate on nucleus pulposus cell: Implications for intervertebral disc regeneration. _Biomaterials_ 82, 34–47 (2016). Article CAS Google Scholar * Yano,
F. _et al._ A novel disease-modifying osteoarthritis drug candidate targeting Runx1. _Ann. Rheum. Dis._ 72, 748–753 (2013). Article CAS Google Scholar * Le Maitre, C. L., Pockert, A.,
Buttle, D. J., Freemont, A. J. & Hoyland, J. A. Matrix synthesis and degradation in human intervertebral disc degeneration. _Biochem. Soc. Trans._ 35, 652–655 (2007). Article Google
Scholar * Mwale, F., Roughley, P. & Antoniou, J. Distinction between the extracellular matrix of the nucleus pulposus and hyaline cartilage: A requisite for tissue engineering of
intervertebral disc. _Eur. Cell Mater._ 8, 58–63 (2004). Article CAS Google Scholar * Fujita, N. _et al._ CD24 is expressed specifically in the nucleus pulposus of intervertebral discs.
_Biochem. Biophys. Res. Commun._ 338, 1890–1896 (2005). Article CAS Google Scholar * Risbud, M. V. _et al._ Evidence for skeletal progenitor cells in the degenerate human intervertebral
disc. _Spine (Phila Pa 1976)_ 32, 2537–2544 (2007). Article Google Scholar * Sakai, D. _et al._ Exhaustion of nucleus pulposus progenitor cells with ageing and degeneration of the
intervertebral disc. _Nat. Commun._ 3, 1264 (2012). Article ADS Google Scholar * Kakiuchi, Y. _et al._ Pharmacological inhibition of mTORC1 but not mTORC2 protects against human disc
cellular apoptosis, senescence, and extracellular matrix catabolism through Akt and autophagy induction. _Osteoarthr. Cartil._ 27, 965–976 (2019). Article CAS Google Scholar * Chijimatsu,
R. _et al._ Effect of the small compound TD-198946 on glycosaminoglycan synthesis and transforming growth factor beta3-associated chondrogenesis of human synovium-derived stem cells in
vitro. _J. Tissue Eng. Regen. Med._ 13, 446–458 (2019). Article CAS Google Scholar * Yano, F. _et al._ Cell-sheet technology combined with a thienoindazole derivative small compound
TD-198946 for cartilage regeneration. _Biomaterials_ 34, 5581–5587 (2013). Article CAS Google Scholar * Hamamoto, S. _et al._ Enhancement of chondrogenic differentiation supplemented by a
novel small compound for chondrocyte-based tissue engineering. _J. Exp. Orthop._ 7, 10 (2020). Article Google Scholar * Ouyang, Z. H., Wang, W. J., Yan, Y. G., Wang, B. & Lv, G. H.
The PI3K/Akt pathway: a critical player in intervertebral disc degeneration. _Oncotarget_ 8, 57870–57881 (2017). Article Google Scholar * Zhang, M. _et al._ IGF-1 regulation of type II
collagen and MMP-13 expression in rat endplate chondrocytes via distinct signaling pathways. _Osteoarthr. Cartil._ 17, 100–106 (2009). Article CAS Google Scholar * Kim, J. S. _et al._
Insulin-like growth factor 1 synergizes with bone morphogenetic protein 7-mediated anabolism in bovine intervertebral disc cells. _Arthritis Rheum._ 62, 3706–3715 (2010). Article CAS
Google Scholar * Wang, B., Wang, D., Yan, T. & Yuan, H. MiR-138-5p promotes TNF-alpha-induced apoptosis in human intervertebral disc degeneration by targeting SIRT1 through
PTEN/PI3K/Akt signaling. _Exp. Cell Res._ 345, 199–205 (2016). Article CAS Google Scholar * Cheng, C. C. _et al._ PI3K/AKT regulates aggrecan gene expression by modulating Sox9 expression
and activity in nucleus pulposus cells of the intervertebral disc. _J. Cell Physiol._ 221, 668–676 (2009). Article CAS Google Scholar * Kushioka, J. _et al._ A novel and efficient method
for culturing mouse nucleus pulposus cells. _Spine J._ 19, 1573–1583 (2019). Article Google Scholar * Love, M. I., Huber, W. & Anders, S. Moderated estimation of fold change and
dispersion for RNA-seq data with DESeq2. _Genome Biol._ 15, 550 (2014). Article Google Scholar * Kanehisa, M. & Goto, S. KEGG: Kyoto encyclopedia of genes and genomes. _Nucleic Acids
Res_ 28, 27–30 (2000). Article CAS Google Scholar * Kanehisa, M., Furumichi, M., Tanabe, M., Sato, Y. & Morishima, K. KEGG: new perspectives on genomes, pathways, diseases and drugs.
_Nucleic Acids Res._ 45, D353–D361 (2017). Article CAS Google Scholar * Kanehisa, M., Sato, Y., Furumichi, M., Morishima, K. & Tanabe, M. New approach for understanding genome
variations in KEGG. _Nucleic Acids Res._ 47, D590–D595 (2019). Article CAS Google Scholar * Kanehisa, M. Toward understanding the origin and evolution of cellular organisms. _Protein
Sci._ 28, 1947–1951 (2019). Article CAS Google Scholar * Ohnishi, T. _et al._ In vivo mouse intervertebral disc degeneration model based on a new histological classification. _PLoS ONE_
11, e0160486 (2016). Article Google Scholar * Walsh, A. J., Bradford, D. S. & Lotz, J. C. In vivo growth factor treatment of degenerated intervertebral discs. _Spine (Phila Pa 1976)_
29, 156–163 (2004). Article Google Scholar * Masuda, K. _et al._ A novel rabbit model of mild, reproducible disc degeneration by an anulus needle puncture: correlation between the degree
of disc injury and radiological and histological appearances of disc degeneration. _Spine (Phila Pa 1976)_ 30, 5–14 (2005). Article Google Scholar * Tam, V. _et al._ Histological and
reference system for the analysis of mouse intervertebral disc. _J. Orthop. Res._ 36, 233–234 (2017). PubMed Google Scholar Download references ACKNOWLEDGEMENTS This work was supported by
JSPS Grant-in-Aid (C) Grant Number 17K11005 and JSPS bilateral Joint Research Project Grant Number 1007397. The study sponsors had no involvement in this study. We thank Ms. Fumiko Hirayama,
Ms. Yukiko Eguchi, and Ms. Mari Shinkawa for their exceptional contribution to this work. AUTHOR INFORMATION AUTHORS AND AFFILIATIONS * Department of Orthopaedic Surgery, Osaka University
Graduate School of Medicine, 2-2 Yamadaoka, Suita, Osaka, 565-0871, Japan Junichi Kushioka, Takashi Kaito, Rintaro Okada, Hiroyuki Ishiguro, Zeynep Bal, Joe Kodama & Hideki Yoshikawa *
Bone and Cartilage Regenerative Medicine, Graduate School of Medicine, The University of Tokyo, 7-3-1 Hongo, Bunkyo-ku, Tokyo, 113-8655, Japan Ryota Chijimatsu & Fumiko Yano * Sensory
and Motor System Medicine, Graduate School of Medicine, The University of Tokyo, 7-3-1 Hongo, Bunkyo-ku, Tokyo, 113-8655, Japan Taku Saito & Sakae Tanaka * Center for Disease Biology and
Integrative Medicine, The University of Tokyo, 7-3-1 Hongo, Bunkyo-ku, Tokyo, 113-8655, Japan Ung-il Chung Authors * Junichi Kushioka View author publications You can also search for this
author inPubMed Google Scholar * Takashi Kaito View author publications You can also search for this author inPubMed Google Scholar * Ryota Chijimatsu View author publications You can also
search for this author inPubMed Google Scholar * Rintaro Okada View author publications You can also search for this author inPubMed Google Scholar * Hiroyuki Ishiguro View author
publications You can also search for this author inPubMed Google Scholar * Zeynep Bal View author publications You can also search for this author inPubMed Google Scholar * Joe Kodama View
author publications You can also search for this author inPubMed Google Scholar * Fumiko Yano View author publications You can also search for this author inPubMed Google Scholar * Taku
Saito View author publications You can also search for this author inPubMed Google Scholar * Ung-il Chung View author publications You can also search for this author inPubMed Google Scholar
* Sakae Tanaka View author publications You can also search for this author inPubMed Google Scholar * Hideki Yoshikawa View author publications You can also search for this author inPubMed
Google Scholar CONTRIBUTIONS J.Kushioka, T.K., and R.C. conceived the study. J.Kushioka, T.K., and R.C. contributed to the acquisition of data. J.Kushioka, T.K., and R.C. contributed to the
data analysis. J.Kushioka, T.K., R.C., R.O., H.I., Z.B., J.Kodama, F.Y., T.S., U.C., S.T., and H.Y discussed the results. J.Kushioka, T.K., and R.C. wrote the initial draft. J.Kushioka,
T.K., R.C., R.O., H.I., Z.B., J.Kodama, F.Y., T.S., U.C., S.T., and H.Y. revised the manuscript and approved the final version. CORRESPONDING AUTHOR Correspondence to Takashi Kaito. ETHICS
DECLARATIONS COMPETING INTERESTS The authors declare no competing interests. ADDITIONAL INFORMATION PUBLISHER'S NOTE Springer Nature remains neutral with regard to jurisdictional claims
in published maps and institutional affiliations. SUPPLEMENTARY INFORMATION SUPPLEMENTARY INFORMATION RIGHTS AND PERMISSIONS OPEN ACCESS This article is licensed under a Creative Commons
Attribution 4.0 International License, which permits use, sharing, adaptation, distribution and reproduction in any medium or format, as long as you give appropriate credit to the original
author(s) and the source, provide a link to the Creative Commons license, and indicate if changes were made. The images or other third party material in this article are included in the
article’s Creative Commons license, unless indicated otherwise in a credit line to the material. If material is not included in the article’s Creative Commons license and your intended use
is not permitted by statutory regulation or exceeds the permitted use, you will need to obtain permission directly from the copyright holder. To view a copy of this license, visit
http://creativecommons.org/licenses/by/4.0/. Reprints and permissions ABOUT THIS ARTICLE CITE THIS ARTICLE Kushioka, J., Kaito, T., Chijimatsu, R. _et al._ The small compound, TD-198946,
protects against intervertebral degeneration by enhancing glycosaminoglycan synthesis in nucleus pulposus cells. _Sci Rep_ 10, 14190 (2020). https://doi.org/10.1038/s41598-020-71193-6
Download citation * Received: 17 March 2020 * Accepted: 07 August 2020 * Published: 25 August 2020 * DOI: https://doi.org/10.1038/s41598-020-71193-6 SHARE THIS ARTICLE Anyone you share the
following link with will be able to read this content: Get shareable link Sorry, a shareable link is not currently available for this article. Copy to clipboard Provided by the Springer
Nature SharedIt content-sharing initiative