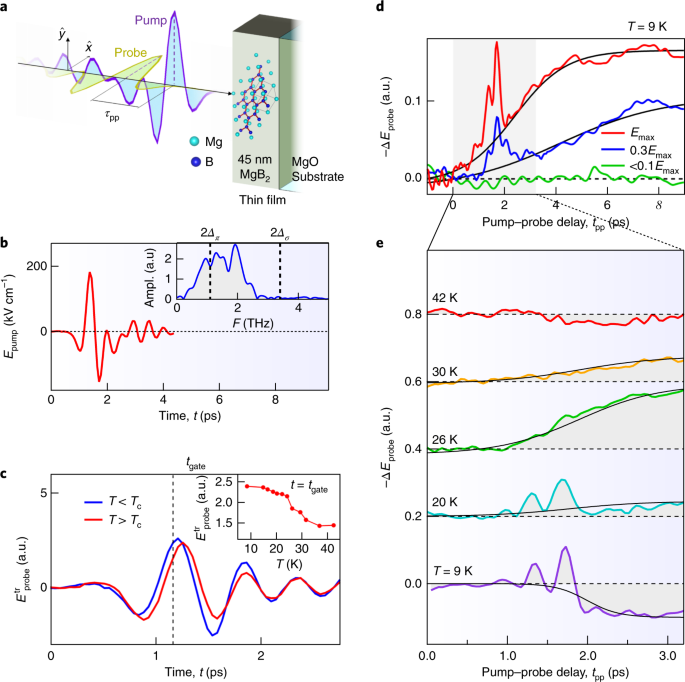
- Select a language for the TTS:
- UK English Female
- UK English Male
- US English Female
- US English Male
- Australian Female
- Australian Male
- Language selected: (auto detect) - EN
Play all audios:
ABSTRACT The discovery of symmetry-broken phases that host multiple order parameters, such as multiband superconductors1,2, has triggered an enormous interest in condensed matter physics.
However, many challenges continue to hinder the fundamental understanding of how to control the collective modes corresponding to these multiple order parameters3,4. Here we demonstrate
that, in full analogy with phonons, Raman-active electronic collective modes can be manipulated by intense light pulses. By tuning a sum-frequency excitation process, we selectively trigger
collective excitations that can be ascribed to the relative phase fluctuations between two superconducting order parameters—the so-called Leggett mode—in the multiband superconductor MgB2.
The excellent comparison between experiments and theory establishes a general protocol for the advanced control of Raman-active electronic modes in symmetry-broken quantum phases of matter.
Access through your institution Buy or subscribe This is a preview of subscription content, access via your institution ACCESS OPTIONS Access through your institution Access Nature and 54
other Nature Portfolio journals Get Nature+, our best-value online-access subscription $29.99 / 30 days cancel any time Learn more Subscribe to this journal Receive 12 print issues and
online access $259.00 per year only $21.58 per issue Learn more Buy this article * Purchase on SpringerLink * Instant access to full article PDF Buy now Prices may be subject to local taxes
which are calculated during checkout ADDITIONAL ACCESS OPTIONS: * Log in * Learn about institutional subscriptions * Read our FAQs * Contact customer support SIMILAR CONTENT BEING VIEWED BY
OTHERS TERAHERTZ DISPLACIVE EXCITATION OF A COHERENT RAMAN-ACTIVE PHONON IN V2O3 Article Open access 28 April 2022 QUANTUM COHERENCE TOMOGRAPHY OF LIGHT-CONTROLLED SUPERCONDUCTIVITY Article
Open access 05 December 2022 OBSERVATION OF LIGHT-DRIVEN BAND STRUCTURE VIA MULTIBAND HIGH-HARMONIC SPECTROSCOPY Article Open access 02 June 2022 DATA AVAILABILITY The data that support the
plots within this paper and other findings of this study are available from the corresponding author upon reasonable request. REFERENCES * Xi, X. X. Two-band superconductor magnesium
diboride. _Rep. Prog. Phys._ 71, 116501 (2008). Article ADS Google Scholar * Johnston, D. C. The puzzle of high temperature superconductivity in layered iron pnictides and chalcogenides.
_Adv. Phys._ 59, 803–1061 (2010). Article ADS Google Scholar * Nicoletti, D. & Cavalleri, A. Nonlinear light–matter interaction at terahertz frequencies. _Adv. Opt. Photon._ 8,
401–464 (2016). Article Google Scholar * Giannetti, C. et al. Ultrafast optical spectroscopy of strongly correlated materials and high-temperature superconductors: a non-equilibrium
approach. _Adv. Phys._ 65, 58–238 (2016). Article ADS Google Scholar * Zhang, X. C., Alexander Shkurinov & Zhang, Y. Extreme terahertz science. _Nat. Photon._ 11, 16–18 (2017).
Article ADS Google Scholar * Kampfrath, T., Tanaka, K. & Nelson, K. A. Resonant and nonresonant control over matter and light by intense terahertz transients. _Nat. Photon._ 7,
680–690 (2013). Article ADS Google Scholar * Landau, L. D. & Lifshitz, E. M. _Statistical Physics_ 3rd edn (Elsevier, Moscow, 2013). * Bardeen, J., Cooper, L. N. & Schrieffer, J.
R. Microscopic theory of superconductivity. _Phys. Rev._ 106, 162–164 (1957). Article ADS MathSciNet Google Scholar * Volkov, F. & Kogan, S. M. Collisionless relaxation of the energy
gap in a superconductor. _Zh. Eksp. Teor. Fiz._ 65, 2038–2046 (1973). Google Scholar * Pekker, D. & Varma, C. M. Amplitude/Higgs modes in condensed matter. _Annu. Rev. Condens. Matter
Phys._ 6, 269–297 (2015). Article ADS Google Scholar * Sooryakumar, R. & Klein, M. V. Raman scattering by superconducting-gap excitations and their coupling to charge-density waves.
_Phys. Rev. Lett._ 45, 660 (1980). Article ADS Google Scholar * Méasson, M. A. et al. Amplitude Higgs mode in the 2H−NbSe2 superconductor. _Phys. Rev. B_ 89, 060503(R) (2014). Article
ADS Google Scholar * Grasset, R. et al. Higgs-mode radiance and charge-density-wave order in 2H-NbSe2. _Phys. Rev. B_ 97, 094502 (2018). Article ADS Google Scholar * Littlewood, P. B.
& Varma, C. M. Amplitude collective modes in superconductors and their coupling to charge-density waves. _Phys. Rev. B_ 26, 4883 (1982). Article ADS Google Scholar * Matsunaga, R. et
al. Higgs amplitude mode in the BCS superconductors Nb1−_x_Ti_x_N induced by terahertz pulse excitation. _Phys. Rev. Lett._ 111, 057002 (2013). Article ADS Google Scholar * Matsunaga, R.
et al. Light-induced collective pseudospin precession resonating with Higgs mode in a superconductor. _Science_ 345, 1145–1149 (2014). Article ADS MathSciNet Google Scholar * Matsunaga,
R. et al. Polarization-resolved terahertz third-harmonic generation in a single-crystal superconductor NbN: dominance of the Higgs mode beyond the BCS approximation. _Phys. Rev. B_ 96,
020505 (2017). Article ADS Google Scholar * Cea, T., Castellani, C. & Benfatto, L. Nonlinear optical effects and third-harmonic generation in superconductors: Cooper pairs versus
Higgs mode contribution. _Phys. Rev. B_ 93, 180507 (2016). Article ADS Google Scholar * Cea, T., Barone, P., Castellani, C. & Benfatto, L. Polarization dependence of the
third-harmonic generation in multiband superconductors. _Phys. Rev. B_ 97, 094516 (2018). Article ADS Google Scholar * Leggett, A. J. Number-phase fluctuations in two-band
superconductors. _Progr. Theoret. Phys._ 36, 901–930 (1966). Article ADS Google Scholar * Blumberg, G. et al. Observation of Leggett’s collective mode in a multiband MgB2 superconductor.
_Phys. Rev. Lett._ 99, 227002 (2007). Article ADS Google Scholar * Klein, M. V. Theory of Raman scattering from Leggett’s collective mode in a multiband superconductor: application to
MgB2. _Phys. Rev. B_ 82, 014507 (2010). Article ADS Google Scholar * Cea, T. & Benfatto, L. Signature of the Leggett mode in the A1g Raman response: from MgB2 to iron-based
superconductors. _Phys. Rev. B_ 94, 064512 (2016). Article ADS Google Scholar * Canfield, P. C. & Crabtree, G. W. Magnesium diboride: better late than never. _Phys. Today_ 56, 34–40
(March, 2003). * Maehrlein, S., Paarmann, A., Wolf, M. & Kampfrath, T. Terahertz sum-frequency excitation of a Raman-active phonon. _Phys. Rev. Lett._ 119, 127402 (2017). Article ADS
Google Scholar * Merlin, R. Generating coherent THz phonons with light pulses. _Solid State Commun._ 102, 207–220 (1997). Article ADS Google Scholar * Rajasekaran, S. et al. Parametric
amplification of a superconducting plasma wave. _Nat. Phys._ 12, 1012–1016 (2016). Article Google Scholar * Murotani, Y., Tsuji, N. & Aoki, H. Theory of light-induced resonances with
collective Higgs and Leggett modes in multiband superconductors. _Phys. Rev. B_ 95, 104503 (2017). Article ADS Google Scholar * Krull, H., Bittner, N., Uhrig, G. S., Manske, D. &
Schnyder, A. P. Coupling of Higgs and Leggett modes in non-equilibrium superconductors. _Nat. Commun._ 7, 11921 (2016). Article ADS Google Scholar * Blumberg, G. et al. Multi-gap
superconductivity in MgB2: Magneto-Raman spectroscopy. _Physica C_ 456, 75–82 (2007). Article ADS Google Scholar * Orenstein, J. & Millis, A. J. Advances in the physics of
high-temperature superconductivity. _Science_ 288, 468–474 (2000). Article ADS Google Scholar * Srivats, S. Rajasekaran et al. Probing optically silent superfluid stripes in cuprates.
_Science_ 359, 575–579 (2018). Article MathSciNet Google Scholar * Kindt, J. T. & Schmuttenmaer, C. A. Theory for determination of the low-frequency time-dependent response function
in liquids using time-resolved terahertz pulse spectroscopy. _J. Chem. Phys._ 110, 8589 (1999). Article ADS Google Scholar * Orenstein, J. & Dodge, J. S. Terahertz time-domain
spectroscopy of transient metallic and superconducting states. _Phys. Rev. B_ 92, 134507 (2015). Article ADS Google Scholar Download references ACKNOWLEDGEMENTS This work was supported by
Swiss National Science Foundation, project nos. IZLRZ2_164051 and 200021_146769. C.P.H. acknowledges association with NCCR-MUST. The work at Temple University was supported in part by the
US Department of Energy, Office of Science, under grant no. DE-SC0011616. T.C. acknowledges financial support by the Graphene Flagship, project CNECTICT-604391. L.B. acknowledges financial
support from Italian Maeci under the Italia–India collaborative project SUPERTOP-PGR04879. AUTHOR INFORMATION Author notes * These authors contributed equally: Flavio Giorgianni, Tommaso
Cea. AUTHORS AND AFFILIATIONS * Paul Scherrer Institute, Laboratory for Non-linear Optics (LNO), Villigen-PSI, Switzerland Flavio Giorgianni, Carlo Vicario & Christoph P. Hauri * IMDEA
Nanoscience, Madrid, Spain Tommaso Cea * ISC-CNR and Department of Physics, Sapienza University of Rome, Rome, Italy Tommaso Cea & Lara Benfatto * Department of Physics, Temple
University, Philadelphia, PA, USA Wenura K. Withanage & Xiaoxing Xi Authors * Flavio Giorgianni View author publications You can also search for this author inPubMed Google Scholar *
Tommaso Cea View author publications You can also search for this author inPubMed Google Scholar * Carlo Vicario View author publications You can also search for this author inPubMed Google
Scholar * Christoph P. Hauri View author publications You can also search for this author inPubMed Google Scholar * Wenura K. Withanage View author publications You can also search for this
author inPubMed Google Scholar * Xiaoxing Xi View author publications You can also search for this author inPubMed Google Scholar * Lara Benfatto View author publications You can also search
for this author inPubMed Google Scholar CONTRIBUTIONS F.G., T.C. and L.B. initiated the research. W.K.W. and X.X. provided the samples. F.G., C.V. and C.P.H. designed the experimental
set-up and carried out the experiment. T.C. and L.B. performed the theoretical calculations. F.G., T.C. and L.B. wrote the manuscript with the input from all co-authors. CORRESPONDING AUTHOR
Correspondence to Lara Benfatto. ETHICS DECLARATIONS COMPETING INTERESTS The authors declare no competing interests. ADDITIONAL INFORMATION PUBLISHER’S NOTE: Springer Nature remains neutral
with regard to jurisdictional claims in published maps and institutional affiliations. SUPPLEMENTARY INFORMATION SUPPLEMENTARY INFORMATION 13 Figures, 14 References RIGHTS AND PERMISSIONS
Reprints and permissions ABOUT THIS ARTICLE CITE THIS ARTICLE Giorgianni, F., Cea, T., Vicario, C. _et al._ Leggett mode controlled by light pulses. _Nat. Phys._ 15, 341–346 (2019).
https://doi.org/10.1038/s41567-018-0385-4 Download citation * Received: 07 March 2018 * Accepted: 21 November 2018 * Published: 21 January 2019 * Issue Date: April 2019 * DOI:
https://doi.org/10.1038/s41567-018-0385-4 SHARE THIS ARTICLE Anyone you share the following link with will be able to read this content: Get shareable link Sorry, a shareable link is not
currently available for this article. Copy to clipboard Provided by the Springer Nature SharedIt content-sharing initiative