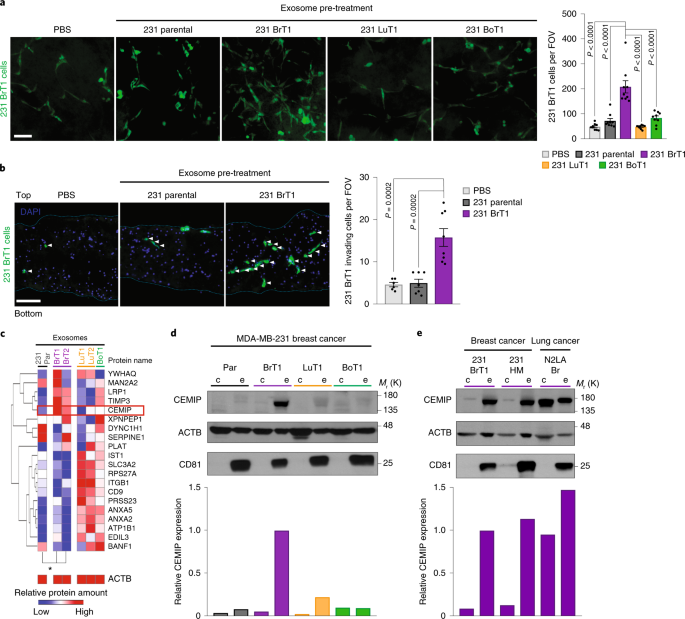
- Select a language for the TTS:
- UK English Female
- UK English Male
- US English Female
- US English Male
- Australian Female
- Australian Male
- Language selected: (auto detect) - EN
Play all audios:
ABSTRACT The development of effective therapies against brain metastasis is currently hindered by limitations in our understanding of the molecular mechanisms driving it. Here we define the
contributions of tumour-secreted exosomes to brain metastatic colonization and demonstrate that pre-conditioning the brain microenvironment with exosomes from brain metastatic cells enhances
cancer cell outgrowth. Proteomic analysis identified cell migration-inducing and hyaluronan-binding protein (CEMIP) as elevated in exosomes from brain metastatic but not lung or bone
metastatic cells. CEMIP depletion in tumour cells impaired brain metastasis, disrupting invasion and tumour cell association with the brain vasculature, phenotypes rescued by
pre-conditioning the brain microenvironment with CEMIP+ exosomes. Moreover, uptake of CEMIP+ exosomes by brain endothelial and microglial cells induced endothelial cell branching and
inflammation in the perivascular niche by upregulating the pro-inflammatory cytokines encoded by _Ptgs2_, _Tnf_ and _Ccl/Cxcl_, known to promote brain vascular remodelling and metastasis.
CEMIP was elevated in tumour tissues and exosomes from patients with brain metastasis and predicted brain metastasis progression and patient survival. Collectively, our findings suggest that
targeting exosomal CEMIP could constitute a future avenue for the prevention and treatment of brain metastasis. Access through your institution Buy or subscribe This is a preview of
subscription content, access via your institution ACCESS OPTIONS Access through your institution Access Nature and 54 other Nature Portfolio journals Get Nature+, our best-value
online-access subscription $29.99 / 30 days cancel any time Learn more Subscribe to this journal Receive 12 print issues and online access $209.00 per year only $17.42 per issue Learn more
Buy this article * Purchase on SpringerLink * Instant access to full article PDF Buy now Prices may be subject to local taxes which are calculated during checkout ADDITIONAL ACCESS OPTIONS:
* Log in * Learn about institutional subscriptions * Read our FAQs * Contact customer support SIMILAR CONTENT BEING VIEWED BY OTHERS EXOSOMAL MIR-155-5P DERIVED FROM GLIOMA STEM-LIKE CELLS
PROMOTES MESENCHYMAL TRANSITION VIA TARGETING ACOT12 Article Open access 19 August 2022 HYPOXIA LUAD H1975 CELL-DERIVED EXOSOMAL MIR-671-3P PROMOTES ANGIOGENESIS VIA REGULATING KLF2-VEGFR2
AXIS Article Open access 16 April 2025 EXOSOMES DERIVED FROM HYPOXIC GLIOMA DELIVER MIR-1246 AND MIR-10B-5P TO NORMOXIC GLIOMA CELLS TO PROMOTE MIGRATION AND INVASION Article 14 January 2021
DATA AVAILABILITY RNA-seq raw data that support the findings of this study have been deposited in the Gene Expression Omnibus under accession code GSE136628. Mass spectrometry raw data have
been deposited in ProteomeXchange with the primary accession code PXD015210. The mass spectrometry processed data of MDA-MB-231 parental (parental), brain-tropic (231-BR (BrT1) and 831
(BrT2)), lung-tropic (4175 (LuT1) and 4173 (LuT2)) and bone-tropic (1833 (BoT1)) exosomes (Fig. 1c) are available in Supplementary Table 2. The processed RNA sequencing data from Fig. 4d and
Supplementary Tables 4, 5, 6, 7, for murine BrECs and microglia cells isolated from ex vivo brain slices treated with PBS, 231 BrT1 WT, 231 BrT1 CEMIP KO1 and KO2 exosomes, are available as
Supplementary Table 3. The processed patient data from Fig. 5 and Supplementary Fig. 5 are available as Supplementary Table 8. Unprocessed scans and replicates for all immunoblots presented
in the manuscript are available as Supplementary Fig. 6. REFERENCES * Valastyan, S. & Weinberg, R. A. Tumor metastasis: molecular insights and evolving paradigms. _Cell_ 147, 275–292
(2011). Article CAS Google Scholar * Maher, E. A., Mietz, J., Arteaga, C. L., DePinho, R. A. & Mohla, S. Brain metastasis: opportunities in basic and translational research. _Cancer
Res._ 69, 6015–6020 (2009). Article CAS Google Scholar * Eichler, A. F. et al. The biology of brain metastases—translation to new therapies. _Nat. Rev. Clin. Oncol._ 8, 344–356 (2011).
Article CAS Google Scholar * Valiente, M. et al. Serpins promote cancer cell survival and vascular co-option in brain metastasis. _Cell_ 156, 1002–1016 (2014). Article CAS Google
Scholar * Sevenich, L. et al. Analysis of tumour- and stroma-supplied proteolytic networks reveals a brain-metastasis-promoting role for cathepsin S. _Nat. Cell Biol._ 16, 876–888 (2014).
Article CAS Google Scholar * Bos, P. D. et al. Genes that mediate breast cancer metastasis to the brain. _Nature_ 459, 1005–1009 (2009). Article CAS Google Scholar * Lorger, M. &
Felding-Habermann, B. Capturing changes in the brain microenvironment during initial steps of breast cancer brain metastasis. _Am. J. Pathol._ 176, 2958–2971 (2010). Article Google Scholar
* Thery, C., Ostrowski, M. & Segura, E. Membrane vesicles as conveyors of immune responses. _Nat. Rev. Immunol._ 9, 581–593 (2009). Article CAS Google Scholar * Peinado, H. et al.
Pre-metastatic niches: organ-specific homes for metastases. _Nat. Rev. Cancer_ 17, 302–317 (2017). Article CAS Google Scholar * Peinado, H. et al. Melanoma exosomes educate bone marrow
progenitor cells toward a pro-metastatic phenotype through MET. _Nat. Med._ 18, 883–891 (2012). Article CAS Google Scholar * Costa-Silva, B. et al. Pancreatic cancer exosomes initiate
pre-metastatic niche formation in the liver. _Nat. Cell Biol._ 17, 816–826 (2015). Article CAS Google Scholar * Hoshino, A. et al. Tumour exosome integrins determine organotropic
metastasis. _Nature_ 527, 329–335 (2015). Article CAS Google Scholar * Lowery, F. J. & Yu, D. Brain metastasis: unique challenges and open opportunities. _Biochim. Biophys. Acta Rev.
Cancer_ 1867, 49–57 (2017). Article CAS Google Scholar * Polleux, F. & Ghosh, A. The slice overlay assay: a versatile tool to study the influence of extracellular signals on neuronal
development. _Sci. STKE_ 2002, pl9 (2002). PubMed Google Scholar * Yoneda, T., Williams, P. J., Hiraga, T., Niewolna, M. & Nishimura, R. A bone-seeking clone exhibits different
biological properties from the MDA-MB-231 parental human breast cancer cells and a brain-seeking clone in vivo and in vitro. _J Bone Miner. Res._ 16, 1486–1495 (2001). Article CAS Google
Scholar * Carbonell, W. S., Ansorge, O., Sibson, N. & Muschel, R. The vascular basement membrane as ‘soil’ in brain metastasis. _PLoS One_ 4, e5857 (2009). Article Google Scholar *
Lorger, M., Krueger, J. S., O’Neal, M., Staflin, K. & Felding-Habermann, B. Activation of tumor cell integrin αvβ3 controls angiogenesis and metastatic growth in the brain. _Proc. Natl
Acad. Sci. USA_ 106, 10666–10671 (2009). Article CAS Google Scholar * Abe, S., Usami, S. & Nakamura, Y. Mutations in the gene encoding KIAA1199 protein, an inner-ear protein expressed
in Deiters’ cells and the fibrocytes, as the cause of nonsyndromic hearing loss. _J. Hum. Genet._ 48, 564–570 (2003). Article CAS Google Scholar * Yoshida, H. et al. KIAA1199, a deafness
gene of unknown function, is a new hyaluronan binding protein involved in hyaluronan depolymerization. _Proc. Natl Acad. Sci. USA_ 110, 5612–5617 (2013). Article CAS Google Scholar *
Evensen, N. A. et al. Unraveling the role of KIAA1199, a novel endoplasmic reticulum protein, in cancer cell migration. _J. Natl Cancer Inst._ 105, 1402–1416 (2013). Article CAS Google
Scholar * Birkenkamp-Demtroder, K. et al. Repression of KIAA1199 attenuates Wnt-signalling and decreases the proliferation of colon cancer cells. _Br. J. Cancer_ 105, 552–561 (2011).
Article CAS Google Scholar * Zhang, Y., Jia, S. & Jiang, W. G. KIAA1199 and its biological role in human cancer and cancer cells (review). _Oncol. Rep._ 31, 1503–1508 (2014). Article
CAS Google Scholar * Yang, X. et al. KIAA1199 as a potential diagnostic biomarker of rheumatoid arthritis related to angiogenesis. _Arthritis Res. Ther._ 17, 140 (2015). Article Google
Scholar * Yoshino, Y. et al. Distribution and function of hyaluronan binding protein involved in hyaluronan depolymerization (HYBID, KIAA1199) in the mouse central nervous system.
_Neuroscience_ 347, 1–10 (2017). Article CAS Google Scholar * Zhang, H. et al. Identification of distinct nanoparticles and subsets of extracellular vesicles by asymmetric flow field-flow
fractionation. _Nat. Cell Biol._ 20, 332–343 (2018). Article CAS Google Scholar * Winkler, F. Hostile takeover: how tumours hijack pre-existing vascular environments to thrive. _J.
Pathol_. 242, 267–272 (2017). Article Google Scholar * Fidler, I. J. The role of the organ microenvironment in brain metastasis. _Semin. Cancer Biol._ 21, 107–112 (2011). Article Google
Scholar * Arnold, T. & Betsholtz, C. The importance of microglia in the development of the vasculature in the central nervous system. _Vasc. Cell_ 5, 4 (2013). Article Google Scholar
* Tran, Q. K., Ohashi, K. & Watanabe, H. Calcium signalling in endothelial cells. _Cardiovasc. Res._ 48, 13–22 (2000). Article CAS Google Scholar * Liebner, S. et al. Wnt/beta-catenin
signaling controls development of the blood–brain barrier. _J. Cell Biol._ 183, 409–417 (2008). Article CAS Google Scholar * Shimizu, H. et al. Hyaluronan-binding protein involved in
hyaluronan depolymerization is up-regulated and involved in hyaluronan degradation in human osteoarthritic cartilage. _Am. J. Pathol._ 188, 2109–2119 (2018). Article CAS Google Scholar *
Burri, P. H., Hlushchuk, R. & Djonov, V. Intussusceptive angiogenesis: its emergence, its characteristics, and its significance. _Dev. Dyn._ 231, 474–488 (2004). Article Google Scholar
* Dimova, I. et al. Inhibition of Notch signaling induces extensive intussusceptive neo-angiogenesis by recruitment of mononuclear cells. _Angiogenesis_ 16, 921–937 (2013). Article CAS
Google Scholar * Giacomini, A. et al. Brain angioarchitecture and intussusceptive microvascular growth in a murine model of Krabbe disease. _Angiogenesis_ 18, 499–510 (2015). Article CAS
Google Scholar * Doron, H., Pukrop, T. & Erez, N. A blazing landscape: neuroinflammation shapes brain metastasis. _Cancer Res._ 79, 423–436 (2019). Article CAS Google Scholar *
Tominaga, N. et al. Brain metastatic cancer cells release microRNA-181c-containing extracellular vesicles capable of destructing blood–brain barrier. _Nat. Commun._ 6, 6716 (2015). Article
CAS Google Scholar * van der Vos, K. E. et al. Directly visualized glioblastoma-derived extracellular vesicles transfer RNA to microglia/macrophages in the brain. _Neuro Oncol._ 18, 58–69
(2016). Article Google Scholar Download references ACKNOWLEDGEMENTS We thank M. Ginsberg, G. Marra, P. Raju and T. Milner for reagents and expert advice; L. Nogues Vera, M. Teixeira and S.
Grass for help in the laboratory; M. Schaeffer for proofreading; T. Zhang and K. Gyan for help with bioinformatics analysis; L. Cohen-Gould, the MSKCC Molecular Cytology Core Facility and
K. Uryu for imaging counselling; T. Miller and F. Fang at the MSKCC Flow cytometry Core Facility, and T. Baumgartner at the Weill Cornell Medicine Flow Cytometry Core, as well as R. Bowman,
for expert cell sorting; and the MSKCC Gene Editing and Screening Core Facility for molecular cloning and gene editing advice. We gratefully acknowledge support from the following funding
sources: the National Cancer Institute (CA169538 to D.L. and CA232093 to D.L.), the US Department of Defense (W81XWH-13-1-0427 to D.L.), the Breast Cancer Research Foundation (to D.L. and
C.M.G.), the Champalimaud Foundation, the Daedalus Fund for Innovation (Weill Cornell Medicine, to D.L.), the Children’s Cancer and Blood Foundation, the Pediatric Oncology Experimental
Therapeutics Investigator’s Consortium Foundation, the Nancy C. and Daniel P. Paduano Foundation, the Eileen and James A. Paduano Foundation, the Sohn Foundation, the Hartwell Foundation,
the Manning Foundation, the Thompson Foundation, the Malcolm Hewitt Wiener Foundation and the Tortolani Foundation. G.R. has been supported by a Peter Oppenheimer Fellowship, awarded by the
American Portuguese Biomedical Research Fund, and by the Fundação para a Ciência e a Tecnologia from Portugal. A.H. was supported by a Susan Komen Foundation for the Cure Fellowship. H.P. is
supported by grants from MINECO (SAF2014-54541-R), Fundación Fero, Asociación Española Contra el Cáncer and Worldwide Cancer Research. C.M.G. is supported by a US Department of Defense
Breast Cancer Research Program Era of Hope Scholar Award (W81XWH-15-1-0201), the US National Cancer Institute (CA193461-01), the National Breast Cancer Coalition’s Artemis Project and the
Pink Gene Foundation. AUTHOR INFORMATION Author notes * These authors contributed equally: Gonçalo Rodrigues, Ayuko Hoshino, Candia M. Kenific, Irina R. Matei. AUTHORS AND AFFILIATIONS *
Children’s Cancer and Blood Foundation Laboratories, Departments of Pediatrics, and Cell and Developmental Biology, Drukier Institute for Children’s Health, Meyer Cancer Center, Weill
Cornell Medicine, New York, NY, USA Gonçalo Rodrigues, Ayuko Hoshino, Candia M. Kenific, Irina R. Matei, Loïc Steiner, Daniela Freitas, Han Sang Kim, Ilana Scandariato, Irene Casanova-Salas,
Haiying Zhang, Alberto Benito-Martin, Linda Bojmar, Yonathan Ararso, Katharine Offer, Quincey LaPlant, Weston Buehring, Huajuan Wang, Xinran Jiang, Héctor Peinado, Maria de Sousa &
David Lyden * Graduate Program in Areas of Basic and Applied Biology, Abel Salazar Biomedical Sciences Institute, University of Porto, Porto, Portugal Gonçalo Rodrigues & Maria de Sousa
* International Research Center for Neurointelligence (WPI-IRCN), The University of Tokyo, Tokyo, Japan Ayuko Hoshino * JST, PRESTO, Tokyo, Japan Ayuko Hoshino * Swiss Institute for
Experimental Cancer Research, School of Life Sciences, Ecole Polytechnique Fédérale de Lausanne, Lausanne, Switzerland Loïc Steiner & Etienne Meylan * i3S-Institute for Research and
Innovation in Health, University of Porto, Porto, Portugal Daniela Freitas * IPATIMUP - Institute of Molecular Pathology and Immunology of the University of Porto, Porto, Portugal Daniela
Freitas * Instituto de Ciências Biomédicas Abel Salazar (ICBAS), University of Porto, Porto, Portugal Daniela Freitas * Yonsei Cancer Center, Division of Medical Oncology, Departments of
Internal Medicine, and Pharmacology, Yonsei University College of Medicine, Seoul, Korea Han Sang Kim * Samuel J. Wood Library, Weill Cornell Medicine, New York, NY, USA Peter R. Oxley *
Public Health Sciences Division/Translational Research Program, Fred Hutchinson Cancer Research Center, Seattle, WA, USA Jinxiang Dai & Cyrus M. Ghajar * Ansary Stem Cell Institute,
Division of Regenerative Medicine, Department of Medicine, Weill Cornell Medicine, New York, NY, USA Chaitanya R. Badwe, Tyler M. Lu & Shahin Rafii * Woman’s Malignancies Branch, Center
for Cancer Research, National Cancer Institute, Bethesda, MD, USA Brunilde Gril & Patricia S. Steeg * Proteomics Resource Center, The Rockefeller University, New York, NY, USA Milica
Tešić Mark, Brian D. Dill & Henrik Molina * Ronald O. Perelman and Claudia Cohen Center for Reproductive Medicine and Infertility, Weill Cornell Medicine (WCM), New York, NY, USA Tyler
M. Lu * Department of Surgery, Memorial Sloan Kettering Cancer Center, New York, NY, USA Yuan Liu & David R. Jones * Thoracic Oncology Service, Memorial Sloan Kettering Cancer Center,
New York, NY, USA Joshua K. Sabari & Charles M. Rudin * Department of Pathology and Laboratory Medicine, Weill Cornell Medicine, New York, NY, USA Sandra J. Shin, Navneet Narula, Paula
S. Ginter & David Pisapia * Breast Medicine Service, Department of Medicine, Memorial Sloan Kettering Cancer Centre, New York, NY, USA Vinagolu K. Rajasekhar * Orthopaedic Service,
Department of Surgery, Memorial Sloan Kettering Cancer Center, New York, NY, USA John H. Healey * Systems Oncology Group, Champalimaud Research, Champalimaud Centre for the Unknown, Lisbon,
Portugal Bruno Costa-Silva * Department of Pathology, University of California, San Diego, La Jolla, CA, USA Shizhen Emily Wang * Department of Cardiothoracic Surgery, Weill Cornell
Medicine, New York, NY, USA Nasser Khaled Altorki * Microenvironment and Metastasis Laboratory, Department of Molecular Oncology, Spanish National Cancer Research Center (CNIO), Madrid,
Spain Héctor Peinado * Department of Medicine, Memorial Sloan Kettering Cancer Center, New York, NY, USA Jacqueline Bromberg * Department of Medicine, Weill Cornell Medicine, New York, NY,
USA Jacqueline Bromberg Authors * Gonçalo Rodrigues View author publications You can also search for this author inPubMed Google Scholar * Ayuko Hoshino View author publications You can also
search for this author inPubMed Google Scholar * Candia M. Kenific View author publications You can also search for this author inPubMed Google Scholar * Irina R. Matei View author
publications You can also search for this author inPubMed Google Scholar * Loïc Steiner View author publications You can also search for this author inPubMed Google Scholar * Daniela Freitas
View author publications You can also search for this author inPubMed Google Scholar * Han Sang Kim View author publications You can also search for this author inPubMed Google Scholar *
Peter R. Oxley View author publications You can also search for this author inPubMed Google Scholar * Ilana Scandariato View author publications You can also search for this author inPubMed
Google Scholar * Irene Casanova-Salas View author publications You can also search for this author inPubMed Google Scholar * Jinxiang Dai View author publications You can also search for
this author inPubMed Google Scholar * Chaitanya R. Badwe View author publications You can also search for this author inPubMed Google Scholar * Brunilde Gril View author publications You can
also search for this author inPubMed Google Scholar * Milica Tešić Mark View author publications You can also search for this author inPubMed Google Scholar * Brian D. Dill View author
publications You can also search for this author inPubMed Google Scholar * Henrik Molina View author publications You can also search for this author inPubMed Google Scholar * Haiying Zhang
View author publications You can also search for this author inPubMed Google Scholar * Alberto Benito-Martin View author publications You can also search for this author inPubMed Google
Scholar * Linda Bojmar View author publications You can also search for this author inPubMed Google Scholar * Yonathan Ararso View author publications You can also search for this author
inPubMed Google Scholar * Katharine Offer View author publications You can also search for this author inPubMed Google Scholar * Quincey LaPlant View author publications You can also search
for this author inPubMed Google Scholar * Weston Buehring View author publications You can also search for this author inPubMed Google Scholar * Huajuan Wang View author publications You can
also search for this author inPubMed Google Scholar * Xinran Jiang View author publications You can also search for this author inPubMed Google Scholar * Tyler M. Lu View author
publications You can also search for this author inPubMed Google Scholar * Yuan Liu View author publications You can also search for this author inPubMed Google Scholar * Joshua K. Sabari
View author publications You can also search for this author inPubMed Google Scholar * Sandra J. Shin View author publications You can also search for this author inPubMed Google Scholar *
Navneet Narula View author publications You can also search for this author inPubMed Google Scholar * Paula S. Ginter View author publications You can also search for this author inPubMed
Google Scholar * Vinagolu K. Rajasekhar View author publications You can also search for this author inPubMed Google Scholar * John H. Healey View author publications You can also search for
this author inPubMed Google Scholar * Etienne Meylan View author publications You can also search for this author inPubMed Google Scholar * Bruno Costa-Silva View author publications You
can also search for this author inPubMed Google Scholar * Shizhen Emily Wang View author publications You can also search for this author inPubMed Google Scholar * Shahin Rafii View author
publications You can also search for this author inPubMed Google Scholar * Nasser Khaled Altorki View author publications You can also search for this author inPubMed Google Scholar *
Charles M. Rudin View author publications You can also search for this author inPubMed Google Scholar * David R. Jones View author publications You can also search for this author inPubMed
Google Scholar * Patricia S. Steeg View author publications You can also search for this author inPubMed Google Scholar * Héctor Peinado View author publications You can also search for this
author inPubMed Google Scholar * Cyrus M. Ghajar View author publications You can also search for this author inPubMed Google Scholar * Jacqueline Bromberg View author publications You can
also search for this author inPubMed Google Scholar * Maria de Sousa View author publications You can also search for this author inPubMed Google Scholar * David Pisapia View author
publications You can also search for this author inPubMed Google Scholar * David Lyden View author publications You can also search for this author inPubMed Google Scholar CONTRIBUTIONS G.R.
designed the experimental approach, performed the experimental work, analysed the data, coordinated the project and wrote the manuscript. A.H. performed PT growth and exosome education in
vivo studies, cancer cell proliferation in vitro studies, cancer cell culture and exosome isolation, coordinated the project and wrote the manuscript. C.M.K. generated CEMIP OE, performed
molecular cloning work and genetic manipulation of cancer cells, cancer cell culture and exosome isolation, coordinated the project and wrote the manuscript. I.R.M. performed brain slice ex
vivo FACS analysis and exosome education in vivo studies, cancer cell culture and exosome isolation, coordinated the project, and wrote and reviewed the manuscript. L.S. performed brain
slice ex vivo experimental work, tissue processing and immunostaining, ex vivo and in vivo ImageJ data analysis and quantification, cancer cell invasion in vitro studies, western blot
analysis, cancer cell culture and exosome isolation, and contributed to figure panel assembly. D.F. performed density gradient exosome isolation, characterization and analysis, western blot
analysis and cancer cell culture. H.S.K. and P.R.O. performed RNA sequencing data analysis. I.S. performed tissue processing and immunostaining, ex vivo and in vivo ImageJ data analysis and
quantification, cancer cell culture and exosome isolation. I.C.-S. performed western blot analysis and assisted in analysis of human data. J.D., C.R.B. and T.M.L. performed in vitro BrEC
experimental work and FACS analysis. M.T.M., B.D.D. and H.M. performed exosome mass spectrometry and proteomic data analysis. A.B.-M. assisted in mouse studies and FACS analysis. H.W. and
X.J. assisted in tissue processing and immunostaining, cancer cell culture and exosome isolation. L.B., K.O., Q.L., Y.A., W.B. and H.W. received and processed human samples. Y.A., W.B. and
H.W. assisted in the maintenance of mouse colonies. Y.L., J.K.S., S.J.S., N.N., J.H.H., N.K.A., C.M.R., D.R.J. and D.P. provided human samples. V.K.R. generated and provided the N2LA human
lung cancer cell line. S.E.W. provided the 231-HM human breast cancer cell line. B.G. and P.S.S. provided the 231BR human breast cancer cell line and gave feedback on the project. S.R.
provided endothelial cell expertise and reagents. D.P. coordinated human studies and contributed to the experimental design. D.P., N.N. and P.S.G. analysed the human data. E.M. and H.Z. read
the manuscript and gave feedback on the project. B.C.-S., H.P., C.M.G. and J.B. contributed to hypothesis discussion, experimental design, data interpretation and project coordination.
M.d.S. coordinated the project, contributed to hypothesis discussion, experimental design, data interpretation and wrote the manuscript. D.L. conceived the hypothesis, led the project,
interpreted the data and wrote the manuscript. CORRESPONDING AUTHORS Correspondence to Maria de Sousa, David Pisapia or David Lyden. ETHICS DECLARATIONS COMPETING INTERESTS The authors
declare no competing interests. ADDITIONAL INFORMATION PUBLISHER’S NOTE Springer Nature remains neutral with regard to jurisdictional claims in published maps and institutional affiliations.
INTEGRATED SUPPLEMENTARY INFORMATION SUPPLEMENTARY FIGURE 1 BRAIN SLICE MODEL TO STUDY THE ROLE OF TUMOUR EXOSOMES IN METASTATIC COLONIZATION. A, Illustration of organotropic metastatic
derivatives1,2,3,4,5,6 of MDA-MB-231 breast cancer cell model (parental (gray) and brain (purple), lung (orange), and bone (green) metastatic) and respective cell-derived exosomes analyzed.
Schematic of the brain slice _ex vivo_ model optimized for the study of exosome-mediated cancer cell brain colonization. B, Schematic of the brain slice model used to study the effects of
exosome pre-treatment on the brain microenvironment and cancer cell phenotypes (left, cancer cell number—whole brain slice mount; and right, cancer cell invasion—brain slice transversal
section). Invading cells (white arrows) inside the region of interest, denoted by the blue square, are comprised of all cancer cells below the first layer of brain cells on the top of the
brain slice. C, Representative whole slice fluorescence images of BrT1 GFP+ cells growing on top of brain slices pre-treated with exosomes or PBS. D, Left, representative immunofluorescence
microscopy images of proliferating Ki-67+ BrT1 GFP+ cells invading brain slices pre-treated with exosomes. White and red arrows indicate invading Ki-67- or Ki-67+ cells, respectively. Right,
quantification of Ki-67+ invading cancer cell number. E, Left, representative fluorescence images of 231 parental mCherry+ cells growing on top of brain slices pre-treated with exosomes or
PBS. Right, quantification of 231 parental mCherry+ cell number. F, Left, immunoblot of CEMIP, small EV and exosomal markers (HSP70, Syntenin-1 and CD81) and ACTB in fractions obtained by
OptiPrep™ density gradient ultracentrifugation7 of BrT1 exosomes. Right, densitometry quantification of protein expression in the initial exosome population (_Input_) and across fractions
with different density, given in arbitrary units [a.u.]. Small EV and exosome-containing fractions are shown between dashed lines. The number of cells per FOV are averages ± SEM, from _n_ =
3, 4 (D) and _n_ = 3 (E) individual brain slices, scoring two fields per slice. A representative experiment of three (D, E) or four (F) independent biological replicates is shown. Brain
slice images (C) are representative of three independent biological replicates. Brain slice sections are stained with DAPI (blue), and dotted blue lines delineate the top and bottom limits
of the brain slice (D). Scale bars, 500µm (C) and 100µm (D, E). Error bars depict mean ± SEM. _P_ values were calculated by ANOVA (E) or two-sided Student’s t-test (D). See Supplementary
Fig. 6 for unprocessed blots. See Supplementary Table 1 for statistics source data. SUPPLEMENTARY FIGURE 2 EXOSOMAL CEMIP MODULATES THE BRAIN VASCULAR NICHE TO SUPPORT METASTASIS. A, Left,
immunoblot of CEMIP expression in cell and exosomal protein extracts from BrT1 WT and BrT1 CEMIP knockout (KO1 and KO2) cells. Immunoblotting for exosomal markers (Syntenin-1 and CD81) and
ACTB is shown below. Right, densitometry quantification of CEMIP normalized to the CEMIP expression in BrT1 WT exosomes. CEMIP expression was normalized to ACTB expression for each sample.
B, Transmission electron microscopy (TEM) of BrT1 WT and BrT1 CEMIP-KO1 and -KO2 exosomes. C, Size distribution and protein content analysis of BrT1 WT and BrT1 CEMIP-KO1 and -KO2 exosomes.
Exosome size (mode, nm) and number were evaluated by NanoSight particle tracking. Protein content per exosome ([particle]/[protein]) was assessed by factoring in the protein concentration.
D, Quantification of BrT1 WT and 231 BrT1 CEMIP-KO1 and -KO2 GFP+ cell number on top of brain slices. E, Left, representative fluorescence microscopy image of BrT1 GFP+ cells growing on top
of the brain slice. Right, representative fluorescence microscopy image of BrT1 GFP+ cells invading the brain slice in transversal section. F, Quantification of proliferation of BrT1 WT and
BrT1 CEMIP-KO1 and -KO2 cells _in vitro_ over three days. G, Quantification of transwell Matrigel invasion of BrT1 WT and BrT1 CEMIP-KO1 and -KO2 cells _in vitro_ over 24 hours. H,
Quantification of BrT1 KO2 GFP+ cells on top of brain slices pre-treated with exosomes or PBS. The number of cells per FOV are averages ± SEM, from _n_ = 8, 9, 9 (D), _n_ = 9, 7, 9, 9 (H)
individual brain slices, scoring two fields per slice; and the number of invading cells per FOV are averages ± SEM, from _n_=3 individual transwell cultures (G), scoring a representative
field per transwell membrane. One of three (A - C, D, H) independent biological replicates is shown. Graphs depicting _in vitro_ proliferation and invasion (F and G) display three
independent biological replicates. TEM images and immunofluorescence brain slice images (B and E) are representative of three independent biological replicates. Scale bars, 200 nm (B), and
100 µm (E). Error bars depict mean ± SEM. _P_ values were calculated by ANOVA (C - D, and F - H). See Supplementary Fig. 6 for unprocessed blots. See Supplementary Table 1 for statistics
source data. SUPPLEMENTARY FIGURE 3 CEMIP LOSS DOES NOT AFFECT PRIMARY TUMOUR GROWTH OR _IN SITU_ GROWTH IN THE BRAIN. A, Left, quantification of brain metastatic _in situ_ growth in mice
intracranially injected with BrT1 WT or BrT1 CEMIP-KO cells. Cranial bioluminescence signal (Total photon flux—photons/second (p/s)) in mice over 3-weeks post-intracranial injection of
GFP-labelled BrT1 WT or BrT1 CEMIP-KO luciferase-positive cells. Right, representative IVIS image of brain signal at week 3. B, Quantification of primary tumour growth8 in mice injected with
BrT1 WT or BrT1 CEMIP-KO cells. One experiment with _n_=5 mice per experimental group was performed (A, B). See Supplementary Table 1 for statistics source data. SUPPLEMENTARY FIGURE 4
EXOSOMAL CEMIP AFFECTS BREC BIOLOGY AND INDUCES VASCULAR REMODELING. A, Schematic of the brain slice model setup for the study of exosome interaction with brain microenvironment resident
cells. B, Representative fluorescence image of BrT1 exosomes (green) interacting with CD31+ BrECs (red) _in vivo_ 24 hours post-intracardiac injection of labelled exosomes. C, Representative
fluorescence image of BrT1 exosomes (green) and associated extravasated rhodamine-labelled Dextran (red) in the brain 24 hours post-intracardiac injection of labelled exosomes (right,
enlarged inset). D, Schematic of the ETF assay setup for studying exosome-dependent vascular network formation by isolated BrECs9,10. E, Left, immunoblot of CEMIP expression in cells and
exosomes of 231 parental Control and 231 parental CEMIP overexpressing (OE) models generated11,12. ACTB and the exosomal marker CD81 are shown below. Right, densitometry quantification of
CEMIP expression is normalized to expression in 231 parental Control exosomes. CEMIP expression was normalized to ACTB expression for each sample. F, Quantification of junction (top) and
isolated segment (bottom) number in the vasculature formed upon pre-treatment with exosomes or PBS. G, Left, representative fluorescence image of tumour vasculature (red) in BrT1 brain
metastases (white). Right, quantification of metastatic tumour and normal vessel diameter in brains from mice injected intracardiacally with BrT1 WT, BrT1 CEMIP-KO1 or -KO2 cells. H,
Schematic of the brain slice model setup for studying exosome-induced gene expression changes in stromal cells of the brain. Brain slices were pre-treated with BrT1 WT, BrT1 CEMIP-KO1 or
-KO2 cell-derived fluorescently-labelled exosomes. I, Flow cytometry analysis of exosome uptake. Percentage of exosome-positive (Exo+) endothelial (CD45_−_ CD31+) and microglial (CD45+
CD11blow CD49dlow)13 cells in brain slices is shown. J, Representative confocal images of the adhesion of fluorescently-labelled BrT1 WT, BrT1 CEMIP-KO1 or -KO2 exosomes with endothelial
cells (CD31+) in the brain slice. Arrows indicate co-localization of exosomes (green) with endothelial cells (red). For _in vivo_ experiments, _n_=4 mice were analyzed per group (G).
Individual vessel diameter was obtained from the average of three measurements along the extension of the vessel. Metastatic tumour and normal brain vascular diameters were scored in up to 5
individual metastatic lesions across two sagittal sections from different brain areas per individual presenting brain metastases (G). The number of junctions and isolated segments per FOV
are averages ± SEM, from _n_ = 5, 7, 8, 7, 8, 8 individual µ-slide angiogenesis chamber wells (F), scoring a representative field per µ-slide well. A representative experiment is shown from
three independent biological replicates (E - F). Graphs depicting endothelial and microglial cell exosome uptake and tumour vasculature diameter (I and G) display the average of three and
two independent biological replicates, respectively. Immunofluorescence images of _in vivo_ exosome uptake by BrECs, vascular leakiness and confocal images of the interaction and BrEC
exosome uptake in the brain slice (B, C and J) are representative of three independent biological replicates. Scale bars, 50µm (B), 50μm and 100μm (C), 100µm (G) and 75µm (J). Error bars
depict mean ± SEM. _P_ values were calculated by ANOVA (F, G, I). See Supplementary Fig. 6 for unprocessed blots. See Supplementary Table 1 for statistics source data. SUPPLEMENTARY FIGURE 5
CEMIP IS A BIOMARKER OF BRM AND IS PRESENT IN EXOSOMES FROM PATIENTS. A, Kaplan-Meier survival curve for brain metastasis patients depicting time to last follow up (LFU) or death from time
of primary tumour diagnosis based on low (green) or high (red) CEMIP expression in brain metastatic tumour. B, Representative image of CEMIP expression in 231 parental and BrT1 cells _in
vitro_ by immunohistochemistry. C, Immunoblot of CEMIP expression in exosomes collected from culture of human brain and bone metastatic tissue explants resected from patients. ACTB was used
as loading control. Marker indicated by m. D, Immunoblot of CEMIP expression in exosomes collected from culture of human non-small cell lung cancer primary tumour tissue resected from
patients. ACTB was used as a loading control. IHC images (B) and immunoblots (C, D) are representative of one experiment. Scale bars, 100µm (B). _P_ values was calculated by Log-rank
(Mantel-Cox) test (A). See Supplementary Fig. 6 for unprocessed blots. See Supplementary Table 1 for statistics source data. SUPPLEMENTARY FIGURE 6 UNPROCESSED WESTERN BLOTS CONTINUED.
Western blot replicates for Fig. 1e (Replicate C is shown in figure). Sample order is the same as specified in respective manuscript figure. Western blot replicates for Supplementary Fig. 1f
(Replicate B is shown in figure). Sample order is the same as specified in respective manuscript figure. Western blot replicates for Supplementary Fig. 2a (Replicate A is shown in figure).
Sample order is the same as specified in respective manuscript figure. Western blot replicates for Supplementary Fig. 4e (Replicate A is shown in figure). Sample order is the same as
specified in respective manuscript figure. Western blots for Supplementary Fig. 5c. Sample order is the same as specified in respective manuscript figure. Western blots for Supplementary
Fig. 5d. Sample order is the same as specified in respective manuscript figure. SUPPLEMENTARY INFORMATION SUPPLEMENTARY INFORMATION Supplementary Figures 1–6 and table titles/legends.
REPORTING SUMMARY SUPPLEMENTARY TABLE 1 Statistics source data. SUPPLEMENTARY TABLE 2 Mass spectrometry data. SUPPLEMENTARY TABLE 3 RNA sequencing data. SUPPLEMENTARY TABLE 4 Heatmap of
significant genes differentially expressed in brain endothelial and microglial cells following BrT1 exosome treatment, relative to the WT condition. SUPPLEMENTARY TABLE 5 Top significant IPA
canonical pathways - BrECs and microglia – 231 BrT1 exosomes and exosomal CEMIP specific – List of canonical pathways affected by 231 BrT1 exosome and exosomal CEMIP treatment in BrECs and
microglia. SUPPLEMENTARY TABLE 6 Heatmap of significant genes differentially expressed in brain endothelial and microglial cells following exosomal CEMIP treatment, relative to the WT
condition. SUPPLEMENTARY TABLE 7 Top 10 significant Gene Ontology - Biological Processes - BrECs and microglia – exosomal CEMIP specific - List of significant Biological Processes affected
by exosomal CEMIP treatment in BrECs and microglia. SUPPLEMENTARY TABLE 8 Patient data. SUPPLEMENTARY TABLE 9 Correlation of CEMIP expression in PTs and metastatic status. SUPPLEMENTARY
TABLE 10 Antibodies and primers. RIGHTS AND PERMISSIONS Reprints and permissions ABOUT THIS ARTICLE CITE THIS ARTICLE Rodrigues, G., Hoshino, A., Kenific, C.M. _et al._ Tumour exosomal CEMIP
protein promotes cancer cell colonization in brain metastasis. _Nat Cell Biol_ 21, 1403–1412 (2019). https://doi.org/10.1038/s41556-019-0404-4 Download citation * Received: 30 May 2019 *
Accepted: 19 September 2019 * Published: 04 November 2019 * Issue Date: November 2019 * DOI: https://doi.org/10.1038/s41556-019-0404-4 SHARE THIS ARTICLE Anyone you share the following link
with will be able to read this content: Get shareable link Sorry, a shareable link is not currently available for this article. Copy to clipboard Provided by the Springer Nature SharedIt
content-sharing initiative