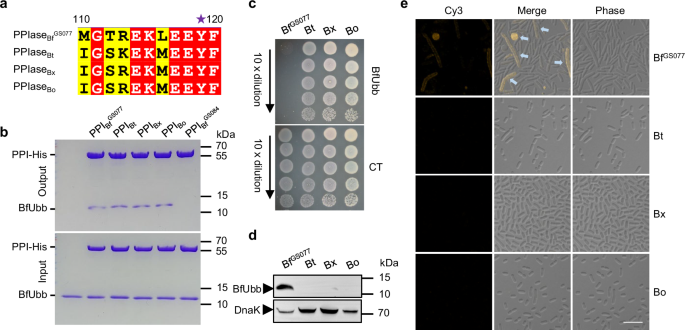
- Select a language for the TTS:
- UK English Female
- UK English Male
- US English Female
- US English Male
- Australian Female
- Australian Male
- Language selected: (auto detect) - EN
Play all audios:
ABSTRACT Efficient interbacterial competitions and diverse defensive strategies employed by various bacteria play a crucial role in acquiring a hold within a dense microbial community. The
gut symbiont _Bacteroides fragilis_ secretes an antimicrobial ubiquitin homologue (BfUbb) that targets an essential periplasmic PPIase to drive intraspecies bacterial competition. However,
the mechanisms by which BfUbb enters the periplasm and its potential for interspecies antagonism remain poorly understood. Here, we employ transposon mutagenesis and identify a highly
conserved TonB-dependent transporter SusCD (designated as ButCD) in _B. fragilis_ as the BfUbb transporter. As a putative protein-related nutrient utilization system, ButCD is widely
distributed across diverse _Bacteroides_ species with varying sequence similarity, resulting in distinct import efficiency of _Bacteroides_ ubiquitin homologues (BUbb) and thereby
determining the species-specific toxicity of BUbb. Cryo-EM structural and functional investigations of the BfUbb–ButCD complex uncover distinctive structural features of ButC that are
crucial for its targeting by BfUbb. Animal studies further demonstrate the specific and efficient elimination of enterotoxigenic _B. fragilis_ (ETBF) in the murine gut by BfUbb, suggesting
its potential as a therapeutic against ETBF-associated inflammatory bowel disease and colorectal cancer. Our findings provide a comprehensive elucidation of the species-specific toxicity
exhibited by BUbb and explore its potential applications. SIMILAR CONTENT BEING VIEWED BY OTHERS _BACTEROIDES FRAGILIS_ UBIQUITIN HOMOLOGUE DRIVES INTRASPECIES BACTERIAL COMPETITION IN THE
GUT MICROBIOME Article 11 December 2023 BTUB TONB-DEPENDENT TRANSPORTERS AND BTUG SURFACE LIPOPROTEINS FORM STABLE COMPLEXES FOR VITAMIN B12 UPTAKE IN GUT _BACTEROIDES_ Article Open access
05 August 2023 BARCODED OVEREXPRESSION SCREENS IN GUT BACTEROIDALES IDENTIFY GENES WITH ROLES IN CARBON UTILIZATION AND STRESS RESISTANCE Article Open access 05 August 2024 INTRODUCTION As
the most abundant phylum of Gram-negative bacteria in the human gut microbiota, _Bacteroidota_ coexist with billions of other microorganisms1. Together, these microbes are crucial for proper
digestion, nutrient absorption, immune system function and overall health of the host2,3,4. Although the majority of _Bacteroides_ are commonly regarded as commensals, certain species
within this genus can also exhibit pathogenicity when residing in the gut or other anatomical sites5,6. Among various pathogenic _Bacteroides_ species, enterotoxigenic _Bacteroides fragilis_
(ETBF) has gained increasing attention due to its association with intestinal disorders, including diarrhoea, inflammatory bowel disease (IBD), and colorectal cancer (CRC)7,8,9,10. In the
highly intricate and densely populated ecosystem of the human intestine, bacteria engage in exploitative and interference competitions, wherein they vie for limited resources and space11,12.
Exploitative competition ensues when bacteria outpace their competitors in resource consumption rates, while interference competition involves the production of inhibitory compounds aimed
at impeding the growth of other bacteria species13,14. These competitive interactions play a pivotal role in shaping microbial communities by exerting influence on population dynamics and
keeping ecological balance15. Exploitative competition serves as a crucial driving force for bacterial evolution and adaptation to diverse environmental conditions and niches13,15.
_Bacteroides_ are equipped with an extensive repertoire of polysaccharide utilization loci (PULs), encoding machinery for glycan degradation and uptake, enabling them to thrive in
fluctuating conditions within the human gut1,16. These PULs typically harbour a diverse array of carbohydrate-active enzymes (CAZymes) responsible for breaking down complex
polysaccharides17,18. Integral to each PUL systems are TonB-dependent transporters (TBDTs), such as the β-barrel forming porin SusC-like proteins, along with their closely associated cognate
SusD-like lipoproteins19,20. SusD functions as a discriminating lid on the bin-like structure of SusC, facilitating selective glycan import via a “pedal-bin” transport mechanism20.
Nevertheless, the function of numerous PULs in their ability to colonize and exploit a niche for _Bacteroides_ remains unidentified. In terms of interference competition, _Bacteroides_
species possess a diverse array of antagonistic factors to outcompete each other. Type VI secretion systems (T6SS) facilitate contact-dependent antagonism that can lead to interspecies
killing21,22,23,24, while diffusible toxins primarily result in intraspecies killing25,26,27,28,29,30,31,32. Our recent study, together with the findings of the Comstock group, demonstrated
that _Bacteroides fragilis_ secretes a ubiquitin homologue (BfUbb) to target an essential periplasmic peptidyl-prolyl isomerase (PPIase) for intraspecies antagonism25,33. Certain strains of
_B. fragilis_ can evade targeting and killing by BfUbb through a single mutation at Tyr119 in the targeted PPIase. However, the mechanism by which BfUbb gains access to the bacterial
periplasm remains elusive. More importantly, it is also unclear whether BfUbb can mediate interspecies antagonism and how its antagonistic range is determined. Here, we identified a unique
yet conserved TonB-dependent transporter SusCD variant in _B. fragilis_ (designated as ButCD for _Bacteroides_ ubiquitin homologues (BUbb) transporter SusCD) that is exploited by BfUbb for
uptake into recipient cells. Although homologues of the ButCD pair are widely distributed among other _Bacteroides_ species, several _Bacteroides_ species encoding BfUbb-targeted PPIase
avoid BfUbb killing due to the lower sequence identity between their ButCD and ButCDBf, which prevents the transport of BfUbb into cells. The role of ButCD in determining the
species-specificity of BUbb is further confirmed, as it is hijacked by BoUbb, another BUbb from _B. ovatus_, to antagonize several _Bacteroides_ species that are resistant to BfUbb. The
cryo-EM structure of BfUbb-ButCDBf complex reveals unique structural features of the BfUbb-targeted ButC (SusC-like) protein that facilities binding and passage of BfUbb through its porin
structure. Considering its specific and potent bactericidal activity against ETBF in mice, BfUbb could potentially assist in therapy for IBD and CRC. Collectively, our study provides a
comprehensive elucidation of the various factors contributing to species-specific toxicity exhibited by BUbb and explores its potential applications. RESULTS BFUBB IS INACCESSIBLE TO CERTAIN
_BACTEROIDES_ SPECIES BfUbb antagonizes certain strains of _B. fragilis_ by targeting an essential periplasmic PPIase, which possesses Tyr119 as the determinant for sensitivity to BfUbb33.
Homologues of this PPIase, containing Tyr119, are widely distributed across numerous sequenced _Bacteroides_ species (Supplementary Fig. 1 and Fig. 1a). Consistent with sequence alignment
results, the purified PPIases from different _Bacteroides_ species interacted directly with BfUbb in vitro (Fig. 1b). However, unlike _B. fragilis_ GS077, which is susceptible to BfUbb, _B.
thetaiotaomicron_ VPI-5482, _B. xylanisolvens_ AM54-2NS and _B. ovatus_ ATCC 8483 all carry the BfUbb-sensitive PPIase variant but resist BfUbb-mediated killing (Fig. 1c). Since BfUbb
requires access to the periplasm of recipient cells to exert its antibacterial function, we speculated that these _Bacteroides_ species, which would otherwise be sensitive to BfUbb killing
due to carrying a BfUbb-sensitive PPIase, have an altered or decreased BfUbb uptake. To assess the capacity of _B. thetaiotaomicron_, _B. xylanisolvens_, and _B. ovatus_ to uptake BfUbb, we
investigated the accessibility of BfUbb in these species. Notably, we detected BfUbb only in cells of BfUbb-susceptible _B. fragilis_ GS077 among the four tested _Bacteroides_ species (Fig.
1d). Additionally, we could visually detect uptake of BfUbb, labelled with a Cy3 fluorescent probe, in the BfUbb-sensitive strain _B. fragilis_ GS077 accompanied by an elongation and cell
rounding phenotype but not in the _B. thetaiotaomicron_, _B. xylanisolvens_, and _B. ovatus_ isolates (Fig. 1e). These results suggest that there exist cellular factors associated with BfUbb
import, which contributes to toxin-sensitivity among various _Bacteroides_ species. A UNIQUE SUSCD IS REQUIRED FOR BFUBB CYTOTOXICITY IN BFUBB-SENSITIVE STRAINS To identify _Bacteroides_
proteins involved in BfUbb uptake, we performed transposon mutagenesis in the BfUbb-sensitive strain _B. fragilis_ GS077 to identify mutants resistant to BfUbb killing when grown in the
presence of purified BfUbb under anaerobic conditions (Fig. 2a). We identified eight transposon mutants of GS077, which exhibited resistance towards BfUbb. Notably, all these mutants
harboured transposon insertions within the gene GS077_4426, encoding a member of the SusC-like protein (designated as SusC*) that functions as an outer membrane TonB-dependent transporter
(TBDT) (Fig. 2b). The domain architecture of SusC* is consistent with typical SusC proteins, featuring an N-terminal signal peptide (SP) for outer membrane translocation, an N-terminal
extension (NTE) domain with unknown function, a TonB box that interacts with the periplasmic protein TonB to energize transport, a β-barrel domain that forms the channel for substrate
uptake, and a plug domain that obstructs the channel (Fig. 2c). SusC-like proteins form a complex with the surface lipoprotein SusD-like proteins, and are usually encoded in adjacent genes.
Downstream of _susC*_, a SusD-like protein (designated as SusD*) is predicted to be encoded by GS077_4425 (Fig. 2b). However, unlike the typical genetic architecture of PUL operons in
_Bacteroides_, which contains additional genes for glycan binding and digestion, there only exists a gene encoding a predicted zinc-dependent metalloprotease (GS077_4427, _ZnMc_) upstream of
_susCD*_ and no additional predicted typical polysaccharide utilization-related genes around (Fig. 2b and Supplementary Fig. 2). We further investigated if the _susCD*_ gene locus
contributed to BfUbb intoxication and uptake by deleting the _susC*_, _susD*_, or _ZnMc_ genes in GS077. Deletion of _susC*_ in GS077 did not alter growth when compared to wildtype in either
rich medium (BHI) or minimal medium (MMF) (Supplementary Fig. 3a). However, in _B. fragilis_ NCTC 9343, deletion of _susC*__Bf9343_ resulted in an extended exit from lag phase in BHI medium
but not in the final cell density when compared to wildtype (Supplementary Fig. 3b). Furthermore, during co-culture between _B. fragilis_ NCTC 9343 wildtype and Δ_susC*__Bf9343_, the
wildtype strain exhibited a growth advantage over the Δ_susC*__Bf9343_ mutant (Supplementary Fig. 3c). Interestingly, the SusD*Bf9343 protein is primarily detected during early exponential
phase, suggesting a role for SusCD* in prioritizing nutrients present in the environment34,35 (Supplementary Fig. 3d). Consistent with the transposon screen, deletion of _susC*_ or _susD*_
in GS077 eliminated its sensitivity to purified BfUbb or supernatant from BfUbb-encoding strain _B. fragilis_ NCTC 9343 (Fig. 2d). This sensitivity was restored by introducing a
SusCD*-expressing plasmid into the deletion strains (Fig. 2d). However, deletion of _ZnMc_ did not confer BfUbb tolerance to GS077 in agar spot assays (Fig. 2d). Further, BfUbb uptake was
detected in cells of wildtype, _susC*_ complemented mutant, _susD*_ complemented mutant and Δ_ZnMc_ mutant strains. However, BfUbb was not detected in Δ_susC*_ and Δ_susD*_ mutants (Fig.
2e). Consistently, cell-associated BfUbb-Cy3 fluorescence and BfUbb-triggered morphological changes were abolished upon deletion of _susC*_ or _susD*_ (Fig. 2f), indicating that both SusC*
and SusD* are indispensable for the internalization and cytotoxicity of BfUbb. SUSCD* IS CONSERVED ACROSS _B. FRAGILIS_ To evaluate the distribution of the SusC* homologues in _B. fragilis_
species, we conducted a comprehensive search and observed that SusC* is highly conserved not only in BfUbb-sensitive but also in BfUbb-resistant _B. fragilis_ strains (including
BfUbb-encoding strains) (Fig. 2c and Supplementary Fig. 4). Comparative analysis of amino acid sequences between SusC* and SusD* homologues from both BfUbb-sensitive and BfUbb-resistant _B.
fragilis_ strains revealed a sequence identity of 94%–100% for SusC* homologues with respect to SusC*GS077 (Fig. 2c and Supplementary Fig. 4), while SusD* homologues were found to be
88%-100% identical in protein sequence when compared to SusD*GS077 (Supplementary Fig. 5). Consistent with the substantial sequence identity of SusCD* between BfUbb-sensitive and resistant
_B. fragilis_ strains, the western blot and confocal microscopy experiments showed that the BfUbb-resistant strain exhibits a SusCD*-dependent uptake of BfUbb, albeit without displaying
elongation and cell rounding phenotypes (Fig. 2g, h). The distribution and conservation of SusCD* in _B. fragilis_ highlight its crucial and conserved role, underscoring the significance of
these proteins. BUTCD IS A BFUBB TRANSPORTER Several TBDTs have been identified as crucial bacterial surface receptors or outer membrane transporters, contributing to the strains’
susceptibility to secreted bacteriocins36,37,38,39. The TonB box precedes the N-terminal plug domain of TBDTs, interacting with the C-terminal domain (CTD) of TonB to provide energy for
substrate transport. Disruption of the TonB box abolishes the energy supply from TonB to TBDTs, resulting in transport failure but does not affect substrate binding20,39. In our study, we
observed a complete loss of BfUbb sensitivity in _B. fragilis_ GS077 when TonB box residues of SusC* (115DAVVV119) were deleted (ΔTonB box) or substituted with alanine (TonB box mutant,
115AAAAA119) (Fig. 3a), despite comparable levels of SusCD* expression (Supplementary Fig. 6). Subsequent immunoblotting and fluorescence microscopy analyses confirmed the absence of
detectable BfUbb in both _ΔtonB_ box and TonB box mutant cells (Fig. 3b, c). Collectively, these findings demonstrate that BfUbb utilizes SusCD* as the transporter for cellular uptake.
Therefore, we rename GS077_4425-4426 (SusCD*) as ButCD for _Bacteroides_ ubiquitin homologues (BUbb) transporter SusCD. TONB3-EXBBD COMPLEX IS THE ENGINE FOR BUTCD-MEDIATED BFUBB
TRANSPORTATION TonB-dependent transporters (TBDTs) actively utilize the proton motive force generated by the TonB-ExbB-ExbD (TBD) system, a nanomachine composed of three inner membrane
proteins (ExbB, ExbD, and TonB), to facilitate substrate import to the periplasm40,41. Therefore, we investigated the relationship between BfUbb transport and the TBD system. We performed
the genetic dissection of TBD-mediated BfUbb transport in BfUbb-resistant _B. fragilis_ GS084 to track BfUbb uptake independent of cell death. Analysis of the _B. fragilis_ GS084 genome
revealed six _tonB_, four _exbB_ and five _exbD_ homologues (Supplementary Fig. 7). To determine which TonB protein is involved in providing energy for BfUbb transport, we constructed six
individual _tonB_ deletions in GS084. Screening all six _tonB_ deletion mutants revealed that BfUbb was not imported in cells carrying a _tonB3_ deletion (Fig. 3d), but was restored when the
deletion was complemented with wild-type _tonB3_ (Fig. 3e, f). Consistent results were also observed in the BfUbb-sensitive strain GS077 (Fig. 3g), where deletion of _tonB3_ conferred
complete resistance to BfUbb, while BfUbb sensitivity was restored upon _tonB3_ complementation, further confirming that TonB3 facilitates ButCD-mediated transport of BfUbb (Fig. 3h).
Furthermore, GS077 exhibits a similar genomic architecture of the _tonB3_ gene locus to GS084, including two _exbB_ and two _exbD_ that is also observed in other _Bacteroides_ (Fig. 3i and
Supplementary Fig. 7). Deletion of either both _exbB_ or both _exbD_ abolished BfUbb transport. However, complementation with just one _exbB_ or _exbD_ is sufficient to restore BfUbb
transport (Fig. 3j, k), indicating that one _exbB_ or _exbD_ is adequate to stimulate TonB3 for BfUbb uptake in GS077. In summary, the TonB3-ExbBD system serves as the molecular motor
responsible for driving ButCD-mediated BfUbb transport. BUTCD IS DISTINCT FROM CANONICAL POLYSACCHARIDE TRANSPORTERS In contrast to the genomic architecture observed in classical
polysaccharide utilization associated _sus_CD pairs-containing loci, the _butCD_ locus lacks genes predicted to be involved in polysaccharide binding and digestion except for _ZnMc_,
suggesting this locus might confer a specialized function divergent from polysaccharide uptake. The absence of ButCD orthologues in GS077 was further confirmed by OrthoFinder42 and blastp,
underscoring the potential functional uniqueness of ButCD within the SusCD superfamily in GS077. To investigate the potential functionality of ButCD, we conducted an extensive search for
annotated genes within all _susCD_ pairs-containing loci present in strain GS077. These _susCD_ pairs present in GS077 were further grouped into 59 putative _susCD_ pairs-containing loci
(some of which contained multiple _susCD_ pairs), predicted their biological functions, and classified them accordingly (Supplementary Fig. 8). By comparing annotated PULs from PULDB43
(www.cazy.org/PULDB) along with manual proofreading, 43 of 59 putative _susCD_ pairs-containing loci were further assigned as putative PULs, potentially associated with polysaccharide
metabolism and transport (Supplementary Fig. 8). These PULs can be categorized into three subtypes based on the diversity and copy number of glycoside hydrolases: Glycan transport_Sub 1 (≤2
glycoside hydrolases), Sub2 (>2 glycoside hydrolases), and Sub3 (no typical glycoside hydrolase; with genes putatively involved in polysaccharide metabolism, such as sulfatase43,44, among
others) (Supplementary Fig. 8). However, a subset of _susCD_ pairs-containing loci (12/59) lack genes with discernible functional annotations and are therefore tentatively designated as
_susCD_ pairs-containing loci with unknown functions (Supplementary Fig. 8; designated as “function unknown”). Additionally, four _susCD_ pairs-containing loci containing putative
metal-dependent peptidase domains may participate in polypeptide transport (Fig. 4a), including the GS077_4426 _susCD_ pairs identified for BfUbb import and the GS077_3053 _susCD_ pairs
potentially associated with transport of an unidentified protein class. The peptidases within the GS077_0109 _susCD_ pairs may be non-functional due to the presence of a stop codon
truncating the immediate gene downstream of its _susD_. Moreover, SusC in the GS077_1440 _susCD_ pairs lacks a complete beta-barrel domain and may not meet the requirements for
transportation due to the presence of an early stop codon in its reading frame. In summary, among various types of _susCD_ pairs, _butCD_ is predicted to have a distinct functionality
compared to other _susCD_ pairs present in GS077, which may confer its ability to import BfUbb. BUTCD DETERMINES THE SPECIES-SPECIFIC TOXICITY OF BFUBB The presence of ButCD, the essential
transporter of BfUbb, was observed across all _Bacteroides_ species, but not in _Phoecicola_ (except for _Phoecicola coprocola_), _Parabacteroides_ or _Alistipes_ according to the NCBI Nr
database. Subsequently, we examined the similarity of ButC homologues in all _Bacteroides_ species. A phylogenetic analysis based on the sequence alignment of ButC homologues from
_Bacteroides_ (NCBI Nr database, _Bacteroides_ limited, Coverage > 90%) categorizes diverse _Bacteroides_ species into two discrete clades, sharing greater than 60% and less than 60%
identity with ButCGS077, respectively (Fig. 4b). However, the phylogenetic analysis of ButC homologues and the species encoding them revealed that the phylogeny of these homologues does not
align with the phylogeny of their encoding species (Fig. 4b and Supplementary Fig. 9), suggesting horizontal gene transfer or rapid evolutionary diversification of the _butCD_ genes. A
similar observation was also found on the inconsistency between phylogenetic studies of T6SS E/I cassettes and species phylogenies24. Based on this homology analysis, we found that the ButCD
locus of _B. uniformis_ exhibited the highest degree of identity to _B. fragilis_ GS077, followed by the average sequence identity of the ButCD homologues from _B. intestinalis_, _B.
cellulosilyticus_, _B. nordii_, _B. ovatus_, _B. xylanisolvens,_ and _B. thetaiotaomicron_ (Fig. 4c). Although all these _Bacteroides_ species encode a BfUbb-sensitive PPIase (Supplementary
Fig. 1), their sensitivity to BfUbb is correlated with the degree of sequence identity in the ButCD homologues. Among these species, _B. uniformis_ GS313, which has a higher ButCD sequence
identity, showed a certain level of sensitivity towards BfUbb, whereas those with a lower ButCD sequence identity exhibited BfUbb resistance (Fig. 4d). These findings suggest that a narrow
range of moderate sequence identity in ButCD can determine the capability and efficiency of BfUbb uptake in recipients. Furthermore, we assessed whether _Bacteroides_ species carrying
BfUbb-sensitive PPIase were resistant to BfUbb due to their ButCD homologues being unable to import BfUbb. We introduced a plasmid expressing ButCDGS077 into BfUbb-resistant _Bacteroides_
species, such as _B. ovatus_, _B. xylanisolvens,_ and _B. thetaiotaomicron_. Western blot analysis and florescence microscopy revealed that these strains gained the ability to transport
BfUbb (Fig. 4e, f). The acquisition of BfUbb access conferred them with sensitivity to BfUbb (Fig. 4g), indicating the simultaneous attainment of both BfUbb sensitivity and import
capability. Additionally, expression of either ButCGS077 or ButDGS077 alone in _B. thetaiotaomicron_ did not confer sensitivity to this species towards BfUbb (Supplementary Fig. 10a),
indicating that both ButC and ButD from _B. fragilis_ are required for BfUbb transport. Our collective findings demonstrate that ButCD plays a pivotal role in determining the import capacity
of BfUbb, thereby influencing the susceptibility of specific _Bacteroides_ species to BfUbb and shaping its antagonistic range. BUTCD DETERMINES THE SPECIES-SPECIFIC TOXICITY OF DISTINCT
_BACTEROIDES_ UBIQUITIN HOMOLOGUES During our exploration of human gut metagenome datasets, we discovered another human ubiquitin (HmUbb) homologue from _B. ovatus_ (BoUbb), exhibiting about
61% sequence identity with both BfUbb and HmUbb. However, the prevalence of the gene encoding BoUbb in the human gut metagenomes is notably lower compared to BfUbb25, with only four strains
of _B. ovatus_ encoding BoUbb among the sequenced _B. ovatus_ genomes in the NCBI database (~600 strains). Among the 1267 human metagenomic samples in “3 consortium gene catalogue” (3CGC)
dataset44, only 1 of 499 metagenomic samples (SRR1778453) with detectable _B. ovatus_ (~0.2%) also contained the BoUbb gene (Supplementary Table 1). Similar to BfUbb, BoUbb possesses a
distinctive disulfide bond at its C-terminus (Fig. 4h), which was demonstrated to be crucial in mediating intraspecies antagonism by BfUbb33, indicating that BfUbb is likely not the sole
example of an antibacterial ubiquitin homologue. However, contrary to the behaviour of BfUbb, screening results revealed that BoUbb exhibited varying levels of antagonism against strains of
_Bacteroides_ species, including _B. ovatus_, _B. xylanisolvens,_ and _B. thetaiotaomicron_, while being ineffective against strains such as _B. fragilis_ and _B. uniformis_ GS313 (Fig. 4i).
This observation aligns with the sequence identity of ButCD in distinct _Bacteroides_ species (Fig. 4c). Consistent with BfUbb, BoUbb exhibits in vitro binding to PPIases containing Tyr119
and disrupts the cell wall integrity of susceptible cells (Supplementary Fig. 10b, c). We therefore assessed the entry capability of BoUbb in four _Bacteroides_ species and microscopy of
Cy3-labelled BoUbb uptake was correlated to the species’ sensitivity to killing when exposed to BoUbb (Fig. 4j). Moreover, deletion of _butC_ rendered _B. thetaiotaomicron_ resistant to
BoUbb, whereas complementation with a plasmid expressing ButCDBt, and not ButCDBf, reversed this outcome (Supplementary Fig. 10d), verifying the ability of the ButCDBt in recognizing and
importing BoUbb. These findings indicate that alterations in strain specificity between BoUbb and BfUbb primarily arise from their accessibility into recipient cells, which is contingent
upon the distinctive characteristics of ButCD. CRYO-EM STRUCTURE OF BFUBB-BUTCD COMPLEX To elucidate the features of ButCD required for BfUbb recognition and uptake, we determined the
structure of the BfUbb-ButCD complex using single-particle cryo-EM. We isolated BfUbb-bound ButCD complexes directly from _B. fragilis_ GS077 (Supplementary Fig. 11a) and subjected the
purified complex to cryo-EM data collection. After initial two-dimensional classification, the ButCD complex exists as a dimer of ButCD in solution (designated as ButC2D2), which aligns with
previous findings that SusCD-like systems exist as a dimerized complex (Supplementary Fig. 11b)19,20. Further ab-initio reconstruction and hetero-refinement revealed that, contrary to prior
reports indicating the presence of three distinct conformations of open and closed dimeric SusC2D2 transporters (open-open, closed-open and closed-closed) within one dataset20, only the
open-open conformation of ButC2D2 was observed (Supplementary Fig. 11c). We also found that ~30% of the dataset exhibited additional density exclusively within one ButC barrel of the ButC2D2
dimer, which was well fitted by the BfUbb protein structure. No particle populations of a ButC2D2 dimer with both barrels exhibiting a BfUbb were observed (Supplementary Fig. 11c).
Consequently, we determined two ButC2D2 dimeric structures in distinct states: apo-ButC2D2 dimer (designated as the AA state; 2.97 Å) and BfUbb-ButC2D2 complex (designated as the CA state;
3.05 Å) including one apo-ButCD (Fig. 5a, Supplementary Fig. 11d–g and Supplementary Table 2). We further explored the structural and biochemical characteristics of the ButCD complex in
substrate recognition. Given the similar structure of apo-ButCD in both AA and CA states (Supplementary Fig. 12a), we used the structures of the BfUbb-ButCD complex and apo-ButCD derived
from the same ButC2D2 dimer (CA) for the following structural analysis. In both structures, ButC, lacking the N-terminal extension (NTE) domain, consists of an N-terminal TonB box and plug
domain that is inserted into a 22-strand β-barrel with multiple extracellular loops (Fig. 5a). Hinge loops L7 and L8 located close to ButD are responsible for binding ButD (Fig. 5a).
Consistent with the previously elucidated conformation of SusCD in its open state, ButD exhibits an upward tilt and primarily interacts with hinge loops L7 and L8 of ButC, thereby exposing
the substrate binding site and plug domain within the interior of the barrel structure (Fig. 5a). In the BfUbb-ButCD complex, BfUbb is centrally positioned at the extracellular entrance of
ButC, where it predominantly interacts with the extracellular loop regions of ButC while exhibiting no interactions with ButD or the plug region of ButC (Fig. 5a). Structural alignment of
BfUbb-bound and apo-ButCD shows no significant conformational changes in ButCD upon binding by BfUbb (Supplementary Fig. 12b-e). These observations suggest that the BfUbb-bound ButCD complex
represents a transport intermediate state where BfUbb is transferred to the entrance of ButC. To better understand the selection of ButCD as a transporter for BfUbb, we superimposed and
compared our ButCD structure with previously determined structures of SusCD-like complexes. Structural comparisons between ButCD, RagAB from _Porphyromonas gingivalis_, and BT1762-1763 from
_B. thetaiotaomicron_ in their open states show that the SusD-like (lid) proteins (ButD, BT1762, RagB) are angled at different degrees compared to their cognate SusC-like (barrel) proteins
(ButC, BT1763, RagA, respectively) (Fig. 5b and Supplementary Fig. 12f, g). We found that the aperture angle between the SusD lid and SusC barrel is 84.98° in ButCD, versus 70.25° for RagAB
and 47.17° for BT1762-1763. This difference is primarily driven by the differences observed in the hinge loops L7 and L8 of the SusC-like proteins. (Fig. 5c and Supplementary Fig. 12h).
Additional comparisons of the BfUbb-ButCD complex structure reveal that the position of the SusD-like proteins, RagB or BT1762, when aligned with its cognate SusC-like proteins, RagA and
BT1763, respectively, could sterically prevent access to a larger substrate such as BfUbb (Fig. 5d, e). Moreover, when compared to RagA and BT1763, extracellular loops L3, L4, L5, L9 and L10
of ButC remain in close proximity, restricting the size of the entrance to ButC (Fig. 5f, g). This structural arrangement of ButC potentially allows for establishing extensive polar
interactions required for BfUbb capture (Fig. 5h). To validate the observations above, we engineered specific amino acid mutants in both proteins to assess their impact on BfUbb transport.
Alanine substitutions of BfUbb residues interacting with ButC in cryo-EM (both K6 and T14, or D32, N60, or all four residues (designated as 4M) of BfUbb) decreased BfUbb import in recipient
cells at varying degrees, which resulted in corresponding reductions in cellular toxicity of sensitive cells (Fig. 5i, j). Alanine substitution of specific ButC amino acids (Y434, R628, N972
or Y1010/Q1014/K1020) involved in the interaction with BfUbb resulted in reduced import efficiency (Supplementary Fig. 12i), leading to altered sensitivity at varying degrees (Fig. 5k).
Altogether, ButCD exhibits appropriate structural and biochemical characteristics that facilitate the recognition and import of BfUbb. Additionally, we substituted ButCBf amino acids R628
and N972 with the corresponding amino acids from the ButCBt protein and found that corresponding mutations, R628Q, N972D, and R628Q/N972D, in _B. fragilis_ strain GS077 disrupted BfUbb
transport and conferred resistance to toxicity, supporting a role for residues R628 and N972 of ButCBf in substrate specificity (Supplementary Fig. 12j, k and Supplementary Fig. 13). These
findings suggest the existence of a sophisticated mechanism through which BfUbb selectively recognizes ButCD, thereby contributing to the species-specific toxicity of BfUbb. BFUBB ELIMINATES
ENTEROTOXIGENIC _BACTEROIDES FRAGILIS_ IN THE ANIMAL GUT Enterotoxigenic _Bacteroides fragilis_ (ETBF) is strongly associated with the development of inflammatory bowel disease (IBD) and
colorectal cancer (CRC)7,8,9. Sequence examination of several characterized disease-associated ETBF strains7,8,9,45,46 reveals that they are likely sensitive to BfUbb due to the presence of
the highly conserved ButCD homologues (>99.7% sequence identity) and the inclusion of the BfUbb-binding residue, Tyr119, within the targeted PPIase (Fig. 6a). Therefore, we examined
whether BfUbb could decrease the abundance of these ETBF in the mammalian gut, which may serve as a potential therapeutic intervention for human diseases associated with ETBF. Consistent
with the finding above, both purified and secreted BfUbb efficiently inhibit cell viability in a disease-related ETBF ATCC 43860 strain in vitro (Supplementary Fig. 14a, b). The MIC50 of
BfUbb against this ETBF strain is 100-fold lower than that of tetracycline and chloramphenicol (Fig. 6b and Supplementary Fig. 14c). The replacement of ETBF’s PPIase110-120aa with that from
BfUbb-resistant _B. fragilis_ strains reversed the sensitivity of ETBF to BfUbb, confirming that the BfUbb-sensitivity of ETBF is also determined by the PPIase (Fig. 6c and Supplementary
Fig. 14b). To investigate whether BfUbb could decrease ETBF abundance in the mammalian gut, we co-colonized three groups of antibiotic-treated mice with both BfUbb-sensitive ETBF and an
equal amount of BfUbb-resistant ETBF-PPIGS084 (Fig. 6d). One day post BfUbb gavage (L100-55 polymer encapsulated BfUbb), the abundance of the BfUbb-sensitive ETBF decreased ~100-fold
compared to vehicle alone (Fig. 6e, Group 1 and 2). After 7 days of daily BfUbb administration, the population of the BfUbb-sensitive ETBF dropped ~ 1000-fold, while the BfUbb-resistant
ETBF-PPIGS084 remained colonization (Fig. 6e, Group 2). In contrast, ETBF in both the BfUbb-untreated and control groups showed stable colonization within the mouse intestine and maintained
high abundance (Fig. 6e, Groups 1 and 3). Altogether, these results indicate that BfUbb administration can specifically and effectively eliminate certain disease-associated ETBFs in vitro
and in vivo, suggesting its potential as a supporting therapeutic against ETBF-involved disorders. DISCUSSION In the present study, we demonstrate that ButCD serves as a distinct and
conserved TonB-dependent outer-membrane transporter in _B. fragilis_, facilitating the transport of BfUbb and governing antagonistic range of BfUbb across different _Bacteroides_ species.
Here, we further identify molecular strategies employed by other resistant _Bacteroides_ strains to evade BfUbb-mediated killing. Previously, we identified a single mutation of Tyr119 on the
BfUbb-targeted PPIase, leading to evasion of BfUbb targeting by certain strains of _B. fragilis_ and conferring resistance against intraspecies competition (Fig. 6f). Additionally, in order
to evade interspecies antagonism, those _Bacteroides_ strains harbouring susceptible PPIase successfully avoid killing from BfUbb by virtue of their ButCD homologues which lack the ability
to import BfUbb (Fig. 6f). Our findings collectively elucidate the diverse and nuanced strategies employed by _Bacteroides_ strains to mount a defence against a BfUbb threat. Consistent with
findings above, the strain-level analysis on human gut metagenome samples (3 consortium gene catalogue (3CGC44) dataset) revealed that although all other _Bacteroides_ species encoded
BfUbb-sensitive PPIase (Supplementary Fig. 1), there was no significant disparity in the relative abundance of other _Bacteroides_ species between samples with detectable BfUbb-encoding _B.
fragilis_ strains and those without (Supplementary Fig. 15a and Supplementary Table 3). However, a significant decrease in the relative abundance of sensitive PPIase-encoding _B. fragilis_
strains was observed in the samples where BfUbb-encoding strains were detected compared to those where they were undetected (Supplementary Fig. 15b and Supplementary Table 3). These findings
further support the intraspecies antagonistic specificity of BfUbb and suggest that alterations in the abundance of sensitive PPIase-encoding _B. fragilis_ strains may be associated with
variations in the presence of BfUbb-encoding strains. However, the abundance of non-_B. fragilis Bacteroides_ species is not correlated with the presence of BfUbb in the community. ButCD in
_B. ovatus_, _B. xylanisolvens,_ and _B. thetaiotaomicron_ exhibits a high degree of amino acid sequence conservation (Fig. 4c and Supplementary Fig. 13), allowing BoUbb to effectively
exploit the ButCD of these diverse _Bacteroides_ species and confer a broader range of antagonism (Fig. 4i). However, due to less amino acid sequence conservation of ButCD from _B. fragilis_
compared to other _Bacteroides_ species (Fig. 4c and Supplementary Fig. 13), BfUbb is unable to enter the periplasm of non-_B. fragilis_ species efficiently, resulting in a narrow target in
species antagonism (Fig. 4d). Therefore, the differences in strain specificity between BoUbb and BfUbb primarily stem from their ability to enter recipient cells, which is determined by the
unique characteristics of ButCD within recipient cells. These findings offer a comprehensive elucidation of the species-specific toxicity exhibited by BfUbb and the broader range of
antagonism demonstrated by BoUbb. SusCD complexes, which serve as transporters of glycans, are abundant and widely distributed in _Bacteroides_. While several TBDTs associated with
oligopeptide or bacteriocin import have been identified, no analogous cases have been reported in _Bacteroides_. ButCD, responsible for BfUbb import, exhibits distinct characteristics
compared to other SusCD proteins in terms of gene loci and evolutionary classification, strongly suggesting that the native substrate(s) of ButCD are different from glycans. Considering the
presence of a zinc-dependent metalloprotease upstream of the ButC gene locus, it is plausible that this ButCD plays a crucial role in recognizing and importing protein substrates as a source
of amino acids for their incorporation into newly synthesized proteins. Therefore, BfUbb is internalized through a potential protein-specific nutrient uptake system, as hypothesized by
Chatzidaki-Livanis et al.25. However, further investigations are required to elucidate exact function and biological substrates of ButCD. It has been proposed that the archetypal SusCD-like
systems generally have a total substrate size limit of ~5 kDa20. Our western blot analyses for detecting BfUbb transport did not show any degradation of BfUbb (~8.8 kDa), raising an
intriguing question regarding whether BfUbb requires unfolding and refolding during import or whether ButCD can directly transport larger substrates such as BfUbb. Further structural and
biochemical investigations are necessary to elucidate how ButCD recognizes and transports BfUbb. Although the TonB-ExbB-ExbD system has been extensively investigated in Gram-negative
bacteria and plays a pivotal role in bacterial survival under nutrient-limited conditions40,41, the inclusion of two ExbBs and two ExbDs within one TBD system has not been widely reported.
Considering the lack of significant similarity between 2 ExbBs or 2 ExbDs, and that complementation with either _exbB_ or _exbD_ is sufficient to restore normal BfUbb transport, it remains
unknown whether all these ExbBs and ExbDs are expressed and form the TBD complex, as well as what proportions they adopt to constitute this complex. BfUbb shows the potential to eliminate
disease-associated ETBF. With its precise targeting, BfUbb is anticipated to minimize disturbance to the gut microbiota compared to conventional antibiotic treatments. Considering
significant involvement of ETBF in the onset of IBD and CRC, BfUbb holds potential as a viable therapeutic option against ETBF-associated diseases, particularly in early-stage intervention.
Given that the _bft_ gene, which encodes the _B. fragilis_ toxin, is located on a transposable element that has mobilized across the _B. fragilis_ species47, including those carrying
non-susceptible PPIase, it is highly plausible that certain ETBF strains exhibit resistance to BfUbb. Therefore, a limitation arises due to the insensitivity of certain ETBF strains to BfUbb
exposure, restricting its applicability in managing all ETBF-associated diseases. METHODS BACTERIAL STRAINS AND GROWTH CONDITIONS Bacterial strains used in this study are listed in
Supplementary Table 4. All _Bacteroides_ strains were cultured in liquid brain heart infusion medium with L-cysteine (1 g/L), hemin (5 mg/L), and vitamin K1 (0.25 mg/L) (BHI), or on BHI agar
plates at 37 °C in an anaerobic chamber48. _E. coli_ strains were grown aerobically in lysogeny broth (LB) medium at 37 °C. Antibiotics were added to the medium as follows when required:
kanamycin 100 mg/mL, ampicillin 50 mg/mL, gentamicin 200 mg/mL, erythromycin 25 mg/mL, chloramphenicol 10 mg/mL, and tetracycline 10 mg/mL. Anhydrotetracycline (aTC) was dissolved in 100%
ethanol at 2 mg/mL as stock solution and diluted 10,000 × when used for counter selection. PLASMID CONSTRUCTION All plasmids used in this study are presented in Supplementary Table 5. For
recombinant protein expression in _E. coli_, BfUbb or BoUbb lacking its SPI signal was cloned into the pET28a vector, introducing an N-terminal 6 × His-SUMO (small ubiquitin-like motif) tag.
PPIase genes from various _Bacteroides_ strains were cloned into a pET15b vector including an N-terminal signal peptide pelB for periplasmic expression and linked with a C-terminal 6 × His
tag. For in situ genetic manipulation in _Bacteroides_, ~ 900 bp overlap upstream and downstream of the target region were cloned into pSIE-Bfe1-CmR vector containing Bfe1 as counter
selective marker49. A 6 × His tag was introduced into C-terminus of ButD in situ via pSIE-Bfe1-CmR to obtain ButCD complex. For expression or complementation in _Bacteroides_, full length
_tonB3_, _exbB1_, _exbB2_, _exbB1B2_, _exbD1_, _exbD2, exbD1D2_, and their ~ 250 bp upstream were cloned into pNBU2_CmR vector, respectively. Genes like _butC_, _butD_, _butCD, butC__Bt__D,_
and _butCD__Bt_ were cloned into pNBU2_CmR vector, introducing a _tonB3_ promotor to enhance expression level50. Plasmids expressing BfUbb mutants or ButC mutants were obtained using quick
change strategy and other constructs were generated using the Gibson assembly strategy. All plasmids were verified by DNA sequencing. PROTEIN EXPRESSION AND PURIFICATION _E. coli_ BL21 (DE3)
carrying a plasmid expressing 6 × His-SUMO-BfUbb construct were grown in 2 L LB medium to an optical density at 600 nm (OD600) of 0.8, then induced by addition of 0.3 mM
isopropyl-β-d-thiogalactopyranoside (IPTG) and cultures were further incubated for 12 h at 18 °C. Bacterial cells were collected by centrifugation and the pellets were resuspended in 15 mL
TBS buffer (20 mM Tris-HCl, pH 8.0, 150 mM NaCl) each liter culture. Bacterial cells were lysed using a high-pressure cell crusher (Union-Biotech), the supernatants were collected, run
through Ni-NTA agarose resin (Qiagen), washed with 20 mM Tris-HCl, pH 8.0, 150 mM NaCl and 20 mM imidazole. The SUMO tag was removed with homemade 6 × His-tagged ULP1 protease at 25 °C for 3
h and proteins were further purified using Superdex 75 gel-filtration chromatography51 (GE Healthcare Life Sciences). As for BfUbb mutants and BoUbb, the same purification strategy was
applied. For purification of PPIases from different _Bacteroides_, plasmids encoding PPIases with a C-terminal hexahistidine were transformed into _E. coli_ BL21 (DE3) and purified by Ni
affinity chromatography first (Qiagen), further purified through an anion-exchange column (Hitrap Q, GE Healthcare) and Superdex 200 Increase gel-filtration chromatography (GE Healthcare)
pre-equilibrated with TBS buffer. Homologous recombination was used to add a 6 × His-tag to the C terminus of genomic ButD in _B. fragilis_ GS077 strain52. To obtain ButCD-BfUbb complex,
overnight cultures of the _B. fragilis_ GS077 strain was grown about 12 h in BHI medium under anaerobic condition, and subcultured for 4 h next morning in fresh BHI to an OD600 ~ 0.8, then
further cultured for 3 h treated with purified BfUbb at a final concentration of 10 μg/mL. Cells were harvested from 14 L BHI cultures through centrifugation, and the resulting pellets were
resuspended in TBS buffer. Subsequently, high-pressure cell crusher (Union-Biotech) was employed for lysing the cells, followed by centrifugation at 17,000 _g_ for 20 min to eliminate
cellular debris. After ultra-centrifugation at 150,000 _g_ for 60 min, the pellet was extracted with 100 mL 1.5% LDAO (n-Dodecyl-N,N-Dimethylamine-N-Oxide) in TBS buffer for 12 h by gentle
stirring at 4 °C before ultracentrifugation for 30 min at 150,000 _g_. The supernatant was loaded onto a Ni-NTA column and after washing with TBS buffer containing 0.2% LDAO and 20 mM
imidazole, protein was eluted with TBS buffer containing 0.2% LDAO and 300 mM imidazole. Protein was further purified by gel filtration using a Superdex 200 column in TBS buffer containing
0.1% LDAO. The protein from peak fraction was concentrated to 17 mg/mL for the cryo-EM experiments. PROTEIN INTERACTION ANALYSIS EXPERIMENT Purified BfUbb (50 μg) and PPIase (80 μg) proteins
were combined in a 3:1 ratio to test the binding of BfUbb to PPIases from other _Bacteroides_ strains in vitro. 20 μL of Ni-agarose resin (GE Healthcare Life Sciences) was added after 1 h
of incubation at 4 °C, and incubation continued for an additional 30 min. With TBS buffer containing 0.05% Triton X-100, the resins were washed three times. Using 300 mM imidazole in the TBS
buffer, protein bonded to the resin was eluted. SDS-PAGE was used to evaluate the protein samples. Following the methods described above, PPIase from various _Bacteroides_ strains was also
tested for its ability to bind to BoUbb in vitro. OVERLAY ASSAY Unless otherwise indicated, 50 ng of purified BfUbb and its variants were spotted onto standard 9 cm diameter BHI petri dishes
and air-dried prior to overlaying with 4 mL of 0.8% BHI soft agar containing 100 μL of exponential phase (OD600 ~ 0.6) strains under investigation. Following anaerobic overnight incubation
at 37 °C, the inhibitory zone was analyzed. AGAR SPOT ASSAY In brief, strains were cultivated anaerobically to exponential phase in 1 mL of BHI medium at 37 °C, and the density was adjusted
to an OD600 ~ 0.8. In total, 200 μg pure toxin protein or 100 μL filtered supernatant concentrate was spread onto BHI plates and dried, unless otherwise indicated in the figure legend. After
a 10-fold gradient dilution, 2.5 μL of each strain was spotted on plates containing pure toxin protein or filtered supernatant concentrate. After anaerobic overnight incubation at 37 °C,
the inhibitory effect was assessed. When testing the toxicity of donor’s supernatant, 10 mL culture (OD600 ~ 0.8) was centrifuged at 9000 _g_ for 10 min, filtered with a 0.22 μm filter,
concentrated to ~ 500 μL. TRANSPOSON MUTAGENESIS Random mutagenesis of _B. fragilis_ GS077 was conducted using the transposon containing plasmid pMUT2D_TetR and individual mutants were
screened using the agar spot assay for those that were no longer inhibited by BfUbb. To create pMUT2D_TetR, pSAM_BfN30 was modified by replacing the erythromycin resistance gene _ermG_ with
the tetracycline resistance gene _TetR_. The construct was verified by sequencing and transformed into _E. coli_ S17-1 ʎ _pir_. This strain was used for conjugation with _B. fragilis_ GS077
as described elsewhere53. Clones with transposon insertions were selected on 15 cm BHI agar with gentamicin, tetracycline, and 1 mg BfUbb. Transposon mutants gained BfUbb resistance were
further confirmed on a BfUbb containing BHI plate then genomic DNA was purified for arbitrarily-primed PCR. The insertion sites were identified by genome walking and Sanger sequencing
followed a described protocol54. Primers used for genome walking are listed in Supplementary Table 6. DELETION MUTATION AND COMPLEMENTATION Plasmids were transferred into _Bacteroides_
strains by mating with _E. coli_ S17-1 ʎ _pir_. Overnight cultures of _E. coli_ S17-1 donor strains were diluted 100-fold in 2 mL LB medium containing ampicillin and _Bacteroides_ recipients
diluted 100-fold in 10 mL BHI medium. When the recipient strain reached an OD600 of 0.1–0.2 and the donor strain reached an OD600 of 0.2–0.6, donor and recipient strains were mixed at a
1:10 donor: recipient culture volume ratio, centrifuged at 9000 _g_ for 10 min, resuspended in 100 μL of BHI liquid medium and spotted on 6 cm non-selective BHI agar plates for 20 h at 37 °C
under aerobic condition to allow for conjugation. Mating lawns were resuspended in 1 mL LB, then 100 μL of suspension or 10-fold dilution was plated on BHI agar plates containing gentamicin
and erythromycin or chloramphenicol. After cointegrates were verified by PCR, each strain was grown overnight in 1 mL BHI, then 100 μL of 10−3 dilution was plated onto BHI plate containing
200 ng/mL aTC. After 36 – 48 h, single colonies were restreaked and analyzed by PCR and DNA sequencing to distinguish wild type and mutant and confirm the loss of the selection marker. As
for complementation, plasmids containing corresponding genes were inserted into _attN_ site of each deletion mutant by conjugation and verified by PCR. WESTERN IMMUNOBLOT ANALYSIS For BfUbb
access evaluation, strains to be tested were inoculated into 1 mL of BHI medium overnight, diluted into 1 mL fresh BHI medium at a ratio of 1:5, incubated for 1 h with 10 μg of purified
BfUbb or BfUbb mutants, otherwise indicated in figure legends, then washed three times with PBS, lysed with lysis buffer (Sangon) and boiled in 6× sample buffer. Equivalent amounts of
bacterial lysates were separated by electrophoresis using 15% Tricine-SDS-PAGE or 10% Glycine-SDS-PAGE gels. The contents of the gels were transferred onto polyvinylidene difluoride
membranes (Millipore), which were blocked with 5% skim milk before being probed with the primary antibodies (Rabbit anti-BfUbb, this study, 1:500; Rabbit anti-DnaK, Cusabio
#CSB-PA633459HA01EGW, 1:2500; Mouse anti-His, Abclonal #AE003, 1:5000) as indicated and horseradish peroxidase-conjugated secondary antibody (goat anti-rabbit, MBL #458, 1:5000; goat
anti-mouse, MBL #330, 1:5000). FLUORESCENCE MICROSCOPY Strains were anaerobically grown overnight in BHI and then transferred to 1 mL of BHI with 5 μg/mL of BoUbb at 1:100 for continued
culture for 4 h to investigate the morphology of BoUbb-treated strains. The medium was then supplemented with 5 μg/mL of FM 1-43 dye, and incubated for 1 h. The bacteria were examined using
a Zeiss LSM900 laser scanning confocal microscope. To assess the internalization of BfUbb into _Bacteroides_, BfUbb was first fluorescently labelled using a Cy3-SE fluorescent dye (Solarbio,
Beijing, China) and purified using gel filtration chromatography as previously described55. Strains were anaerobically cultivated overnight in BHI before being sub-cultured into 1 mL of BHI
containing 40 μg of fluorescent dye Cy3 labelled BfUbb at 1:25 for continued incubation for 4 h. The samples were then washed at least three times with BHI medium and suspended in 100 μL of
BHI medium before observation. The BfUbb-Cy3 treated bacteria were examined using a Zeiss LSM900 laser scanning confocal microscope. GROWTH CURVE MEASUREMENT Bacteria single colony was
picked from a fresh plate and inoculated into pre-reduced 5 mL BHI medium for overnight culture. Then, strains to be tested were diluted 1:100 in 10 mL fresh BHI medium or 1:50 in 10 mL
fresh minimum media, respectively. Bacteria samples were collected every 1.5 h and OD600 readings were recorded using microplate spectrophotometer (Tecon). Prism version 9.3.0 (GraphPad
Software, San Diego, CA) was used to calculate the data, which are shown as the mean of biological triplicates with the SEM depicted as error bars. CO-CULTURE To simplify screening on BHI
plates, the donor and recipient strains were rendered erythromycin- or chloramphenicol-resistant by harbouring a pNBU2-ermG or pNBU2-CmR plasmid. Donor and recipient strains were cultivated
overnight in 5 mL of BHI medium as start culture, then sub-cultured together into 10 mL fresh BHI medium at 1:100. The co-culture mixture was collected after 8 h incubation and separately
plated on selective BHI plates containing erythromycin or chloramphenicol to count the donor and recipient cell number. CRYO-EM SAMPLE PREPARATION AND DATA COLLECTION Aliquots of 4 µL of the
BfUbb-ButCDBf complex at a concentration of ~17 mg/mL were applied onto glow-discharged holey carbon-coated grids (Quantifoil Au R1.2/1.3, 200 mesh, Beijing Zhongjingkeyi Technology,
Beijing, China). Following a 5 s incubation on the grids under 100% humidity, the grids were blotted for 3 s at 8 °C using a blot force of 0, and then plunge-frozen into liquid ethane using
a Vitrobot Mark IV (Thermo Fisher Scientific, Waltham, MA). The grids were transferred to a Titan Krios (Thermo Fisher Scientific) operating at 300 kV for data acquisition. EPU software
(Thermo Fisher Scientific) was used for automated data collection on a Falcon 4i counting camera with a defocus range of −0.5 – −2.0 μm and at a nominal magnification of 105,000x, resulting
in a calibrated pixel size of 1.18 Å. The accumulated dose was set to 60 electrons per Å2 and a total of 31 frames per movie. Data acquisition parameters can be found in Supplementary Table
2. IMAGE PROCESSING A total of 5468 multi-frame movies were collected and subsequently processed using cryoSPARC56. Drift correction and dose-weighting were carried out using Patch Motion
Corr. Contrast transfer function (CTF) estimation of motion-corrected micrographs was conducted using Patch CTF in cryoSPARC. Approximately 208,926 particles were automatically picked from
500 micrographs, extracted, and classified in 2D to provide templates for template-based picking. Out of 5468 micrographs, a total of 2,571,527 particles were extracted with a box size of
300 pixels for two rounds of 2D classification, after which 995,386 particles were used for 3D classification. Two rounds of ab-initio reconstruction and heterogeneous refinement were used
to discard the remaining bad particles, resulting in two class particles. The two class particles were then subjected to non-uniform refinement and local refinement, with C1 and C2 symmetry,
respectively, resulting in two maps at 2.97 Å and 3.05 Å. A flowchart showing the data processing is shown in Supplementary Fig. 11. MODEL BUILDING INTO CRYO-EM MAPS The initial model of
ButCD from AlphaFold2 and BfUbb crystal structure (PDB ID:8HM1) were docked into electron microscopy density map using UCSF Chimera57, and manually adjusted in Coot58, followed by refinement
using Phenix59 in real space with secondary structure and geometry restraints to prevent structure overfitting. Statistics of 3D reconstruction and model refinement are summarized in
Supplementary Table 2. QUANTIFICATION OF LID OPENING IN DIVERSE SUSCD-LIKE SYSTEMS To measure the degree of lid opening in diverse SusCD-like systems, we selected one amino acid residue
(ButC_F728, RagA_Q670, and BT1762_Y636) located in the hinge loop L7 of the SusC-like protein as the original point, which is stable during the conformational changes20. Subsequently, we
chose an amino acid residue (ButC_W352, RagA_W321, and BT1762_W295) from the SusC-like protein to form a reference line approximately aligned with the outer membrane (OM) plane, and another
amino acid (ButD_Y71, RagB_G78, and BT1762_G64) from SusD-like proteins facing the cavity of barrel as another reference line to establish a comparable angle. The Cα atoms of these selected
amino acid residues align closed during structural comparisons, respectively. SEQUENCE ALIGNMENT AND PHYLOGENETIC ANALYSIS _B. fragilis_ ButC (GS077_4426) and PPIase (GS077_2615) was used in
a tblastn (BLAST + , v.2.12.0 + ) query (_e_-value≤ 1e − 10) against an in-house database composed of whole genome sequences of BfUbb sensitive strains and resistant strains. For BfUbb
homologues in bacteria, the NCBI Nr database was searched (tblastn, _e_-value ≤ 1e − 10, bacteria limited and _B. fragilis_ excluded) using _B. fragilis_ BfUbb (BF9343_3779) and the top 100
hits were saved, combined, and sorted by bitscore. For ButC homologues in _Bacteroides, Phoecicola_, _Parabacteroides,_ or _Alistipes_, the NCBI Nr database was searched (tblastn, _e_-value
≤ 1e − 10, _Bacteroides_ limited) using _B. fragilis_ ButC and the top 5000 hits were saved, combined, and sorted by bitscore. Only sequences with query coverage > 90% were included in
subsequent analysis. For SusCD superfamily protein in _B. fragilis_ GS077, we identified all possible surface lipoprotein SusD in GS077 through homology to IPR012944 (RagB/SusD domain). All
possible TonB-dependent transporters (TBDTs) in GS077 were identified through homology with IPR000531 (TonB-dependent receptor-like, beta-barrel). Only those TBDTs with surface lipoprotein
SusD present downstream were identified as candidate SusCD pairs in GS077. The sorted list was parsed such that a taxonomically broad selection of top hits was retained, and the associated
proteins were aligned together with the query sequence using MAFFT (v.7.487). The sequence alignment of the SusCD protein superfamily was also conducted by MAFFT, retaining the blocks in the
alignment results. Maximum-likelihood phylogenetic trees were constructed using IQTREE (v.2.1.4_beta) after automatic model selection with nodal support tested via 1000 ultrafast
phylogenetic bootstraps. _SUSCD_ PAIRS-CONTAINING LOCI CLASSIFICATION OF _B. FRAGILIS_ GS077 We first located and queried these candidate _susCD_ gene clusters, and predicted and classified
the possible biological functions of these candidate _susCD_ pairs-containing loci. By comparing the gene functions in these 59 _susCD_ pairs-containing loci, these _susCD_ pairs-containing
loci were initially classified. By comparing the _susCD_ pairs-containing loci annotated in PULDB and combining with manual annotation and proofreading, the putative PULs (43 of 59 _susCD_
pairs-containing loci) in GS077 were determined. The classification of PUL is based on the diversity and copy number of glycoside hydrolases. Specifically, if a PUL has more types of
glycoside hydrolases, then the PUL may be involved in the utilization and transport of complex polysaccharides. If the _susCD_ pairs-containing loci only encodes peptidases and related
transporters but not polysaccharide utilization-related genes, then the _susCD_ pairs-containing loci may only be involved in peptide transport. ELIMINATION OF ENTEROTOXIGENIC _BACTEROIDES
FRAGILIS_ IN MICE All animal experiments were supervised and approved by the Animal Research Ethical Inspection Form of Shandong University School of Life Sciences (SYDWLL-2021-16).
Seven-week-old C57BL/6 J female mice were purchased from GemPharmatech Co., Ltd. (Jiangsu, China). The mice were housed in laboratory cages under controlled conditions (25 ± 2 °C, 45 ± 5%
humidity, 12 h light-dark cycle) with free access to autoclaved water and irradiated food. All the mice were given a week to acclimatize and were healthy prior to our studies described
below. Antibiotic cocktails (10 mg each of vancomycin, metronidazole, neomycin, and ampicillin per mice) were administered by oral gavage daily for 5 days. Subsequently, all
antibiotic-treated mice had free access to autoclaved water supplemented with antibiotic cocktails (0.5 g/L vancomycin, 1.0 g/L metronidazole, 1.0 g/L neomycin, and 1.0 g/L ampicillin) and
irradiated feed for 7 days. After antibiotic treatment, faecal pellets were collected and tested for bacterial growth on selective BHI agar (200 μg/mL gentamycin and 10 μg/mL chloramphenicol
or 10 μg/mL erythromycin). Only mice without detectable bacterial growth on either medium were included in the study. The antibiotic-treated mice were randomly divided into three groups
(five mice per group). All antibiotic-treated mice were inoculated with an equal mixture comprising 2.5 × 109 c.f.u. of both the BfUbb-sensitve ETBF strains and 2.5 × 109 c.f.u. of the
BfUbb-insensitive ETBF-PPIGS084 strains. The faecal pellets were collected at the indicated time points. Colonization was monitored in fresh faecal samples that were weighed, mashed, and
vortexed in 1 ml PBS buffer and diluted to count c.f.u. The diluted faecal samples were separately plated on selective BHI agar (200 μg/mL gentamycin and 10 μg/mL chloramphenicol or 10 μg/mL
erythromycin). To avoid protein inactivation during the oral route of BfUbb administration, L100-55 polymers were used to deliver BfUbb, as described previously60. Briefly, a total of 50 mg
of BfUbb was dispersed into 5 mL of CaCl2 (0.5 M) solution first. Under gentle stirring conditions, use an injection pump to inject 5 mL of L100-55 polymer solution (10 mg/mL) into the
mixture at a flow rate of 20 mL/h for 15 min. After the injection of L100-55 polymer solution, gentle stirring is still required for about 30–60 min. Then, HCl solution (pH = 1) was added to
the mixture to maintain pH = 4. After centrifugation (200 _g_, 1 min), microspheres were collected and then stored at 4 °C before use. BfUbb was orally administered daily for 7 days, and
100 μL microspheres per mice per day. The control group was administered an equal amount of L100-55 polymer solution. MIC TEST The strains to be examined (OD600 ~ 0.6) were diluted 200-fold
into 200 μL BHI medium with gradient doses of BfUbb, tetracycline, or chloramphenicol. Cell culture plates were incubated anaerobically for 12 h at 37 °C, and the OD600 was measured. For
every gradient, three repeats were performed. STRAIN-LEVEL RELATIVE ABUNDANCE ESTIMATION IN METAGENOMIC SAMPLES MetaPhlAn 461 and StrainPhlAn 4.162 was used to estimate the relative
abundance of the indicated _Bacteroides_ species or _B. fragilis_ with a specific genotype in the metagenomics sample. To estimate the relative abundance of the indicated _Bacteroides_
species, we utilized the species-specific marker genes obtained from MetaPhlAn’s reference species-specific marker genes database (updated to March 2024). Since the marker genes of _B.
fragilis_ (t__SGB1853, t__SGB1855, t__SGB104919 partial marker genes) do not encompass _PPIase_ and _BfUbb_ gene, we developed a customized database by incorporating the coding sequences of
BfUbb and PPIase orthologues into the species-specific marker genes database of MetaPhlAn 4 to assess the relative abundance of _B. fragilis_ with a specific genotype, following the
guidelines provided in the MetaPhlAn 4 tutorial (refer to section “Customizing the database”). The PPIase subtypes in _B. fragilis_ can be categorized as BfUbb-sensitive _PPIase_ (_B.
fragilis_ GS077_2615 Sensitive _PPIase_: 100% nucleotide sequence identity) and BfUbb-insensitive _PPIase_ (_B. fragilis_ 9343_3784 type Insensitive _PPIase_: 98% nucleotide sequence
identity; _B. fragilis_ GS084_0204 Insensitive _PPIase_: 90% nucleotide sequence identity), based on their sensitivity to BfUbb. To ensure accurate classification of distinct subtypes of
_PPIase_, we have selected a hypervariable region within the full-length _PPIase_ gene as a marker, considering the high degree of nucleotide sequence identity of full-length _PPIase_ gene.
Specifically, we aimed to maintain <90% nucleotide sequence identity between each marker gene (BF9343_3784: 300-490 nt; GS077_2615: 300-490 nt; GS084_3784 500-948 nt). The full-length
_BfUbb_ gene serves as a marker gene for identifying BfUbb-encoding _B. fragilis_ strains. The newly added marker genes were validated for correct indexing using the ‘extract_markers.py’
script in StrainPhlAn. Subsequently, the trimmed metagenomics input was mapped to the custom reference database of MetaPhlAn to determine the relative abundance of specific _Bacteroides_
species or _B. fragilis_ genotypes (using MetaPhlAn output parameters “-t rel_ab”). The resulting data from MetaPhlAn was subsequently utilized as input in StrainPhlan4.1 with default
settings. Since the sample sizes in BfUbb-detected group and BfUbb-undetected group are unequal, unpaired two-tailed Welch’s _t_-test were employed to analyze the statistical significance.
It is noteworthy that in the majority of samples, we consistently observed a lower abundance of PPIase-encoding _B. fragilis_ strains compared to the abundance of _B. fragilis_ (determined
using the default MetaPhlAn 4 markers) (Supplementary Table 3). Given that _PPIase_ is an essential gene of _B. fragilis_, it would be expected that the abundance of PPIase-encoding _B.
fragilis_ strains should be close to that of _B. fragilis_. Therefore, a limitation in our strain-level analysis lies in the potential underestimation of the abundance of PPIase-encoding _B.
fragilis_ strains. STATISTICS AND REPRODUCIBILITY At least three separate experiments were conducted independently, yielding consistent results. Unless otherwise stated, data are presented
as the arithmetic mean ± s.d. GraphPad Prism v.9.3.0. (GraphPad) was used for all statistical analyses. Unpaired two-tailed Student’s _t_-tests were employed to analyze the statistical
significance between two groups, unless otherwise stated. The following is an annotation of the significance of mean comparison: *_P_ < 0.05; **_P_ < 0.01; ***_P_ < 0.001; ****_P_
< 0.0001; NS, not significant. Statistics were evaluated significant when _P_ < 0.05. No statistical method was used to predetermine sample size. No data were excluded from the
analyses. The experiments were not randomized. The Investigators were not blinded to allocation during experiments and outcome assessment. REPORTING SUMMARY Further information on research
design is available in the Nature Portfolio Reporting Summary linked to this article. DATA AVAILABILITY The atomic coordinates and cryo-EM maps generated in this study have beendeposited in
the Protein Data Bank (PDB) and Electron Microscopy Data Bank (EMDB) under the accession code: 8YPT, 8YPU and EMD-39493, EMD-39494, respectively. The raw metagenomics data used in this study
are available in the NCBI database under accession number SRR1778453 or listed in the ‘Metagenomics Sample-SRA ID’ column of Supplementary Table 3. Source data are provided with this paper.
REFERENCES * Wexler, A. G. & Goodman, A. L. An insider’s perspective: _Bacteroides_ as a window into the microbiome. _Nat. Microbiol._ 2, 17026 (2017). * Round, J. L. & Mazmanian,
S. K. The gut microbiota shapes intestinal immune responses during health and disease. _Nat. Rev. Immunol._ 9, 313–323 (2009). Article CAS PubMed PubMed Central Google Scholar *
Lozupone, C. A., Stombaugh, J. I., Gordon, J. I., Jansson, J. K. & Knight, R. Diversity, stability and resilience of the human gut microbiota. _Nature_ 489, 220–230 (2012). Article ADS
CAS PubMed PubMed Central Google Scholar * Chen, J. et al. New insights into the mechanisms of high-fat diet mediated gut microbiota in chronic diseases. _iMeta_ 2, e69 (2023). *
Zafar, H. & Saier, M. H., Jr. Gut _Bacteroides_ species in health and disease. _Gut Microbe._ 13, 1–20 (2021). * Wexler, H. M. _Bacteroides_: the good, the bad, and the nitty-gritty.
_Clin. Microbiol. Rev._ 20, 593–621 (2007). Article CAS PubMed PubMed Central Google Scholar * Chung, L. et al. _Bacteroides fragilis_ toxin coordinates a pro-carcinogenic inflammatory
cascade via targeting of colonic epithelial Cells. _Cell Host Microbe._ 23, 203–214, (2018). Article CAS PubMed PubMed Central Google Scholar * Wu, S. et al. A human colonic commensal
promotes colon tumorigenesis via activation of T helper type 17 T cell responses. _Nat. Med._ 15, 1016–1022 (2009). Article CAS PubMed PubMed Central Google Scholar * Dejea, C. M. et
al. Patients with familial adenomatous polyposis harbor colonic biofilms containing tumorigenic bacteria. _Science_ 359, 592–597 (2018). Article ADS CAS PubMed PubMed Central Google
Scholar * El Tekle, G. & Garrett, W. S. Bacteria in cancer initiation, promotion and progression. _Nat. Rev. Cancer_ 23, 600–618 (2023). Article CAS PubMed Google Scholar *
Cornforth, D. M. & Foster, K. R. Competition sensing: the social side of bacterial stress responses. _Nat. Rev. Microbiol._ 11, 285–293 (2013). Article CAS PubMed Google Scholar *
Peterson, S. B., Bertolli, S. K. & Mougous, J. D. The central role of interbacterial antagonism in bacterial life. _Curr. Biol._ 30, R1203–R1214 (2020). Article CAS PubMed PubMed
Central Google Scholar * Svanback, R. & Bolnick, D. I. Intraspecific competition drives increased resource use diversity within a natural population. _Proc. Biol. Sci._ 274, 839–844
(2007). PubMed Google Scholar * Garcia-Bayona, L. & Comstock, L. E. Bacterial antagonism in host-associated microbial communities. _Science_ 361, eaat2456 (2018). * Ghoul, M. &
Mitri, S. The ecology and evolution of microbial competition. _Trends Microbiol._ 24, 833–845 (2016). Article CAS PubMed Google Scholar * Thomas, F., Hehemann, J.-H., Rebuffet, E.,
Czjzek, M. & Michel, G. Environmental and gut _Bacteroides_: the food connection. _Front. Microbiol._ 2, 93 (2011). * Wardman, J. F., Bains, R. K., Rahfeld, P. & Withers, S. G.
Carbohydrate-active enzymes (CAZymes) in the gut microbiome. _Nat. Rev. Microbiol._ 20, 542–556 (2022). Article CAS PubMed Google Scholar * Brown, H. A. & Koropatkin, N. M. Host
glycan utilization within the _Bacteroidetes_ Sus-like paradigm. _Glycobiology_ 31, 697–706 (2021). Article CAS PubMed Google Scholar * Glenwright, A. J. et al. Structural basis for
nutrient acquisition by dominant members of the human gut microbiota. _Nature_ 541, 407–411 (2017). Article ADS CAS PubMed PubMed Central Google Scholar * Gray, D. A. et al. Insights
into SusCD-mediated glycan import by a prominent gut symbiont. _Nat. Commun._ 12, 44 (2021). * Robitaille, S. et al. Community composition and the environment modulate the population
dynamics of type VI secretion in human gut bacteria. _Nat. Ecol. Evol._ 7, 2092–2107 (2023). * Coyne, M. J. & Comstock, L. E. Type VI secretion systems and the gut microbiota.
_Microbiol. Spectr._ https://doi.org/10.1128/microbiolspec.PSIB-0009-2018 (2019). * Chatzidaki-Livanis, M., Geva-Zatorsky, N. & Comstock, L. E. _Bacteroides fragilis_ type VI secretion
systems use novel effector and immunity proteins to antagonize human gut _Bacteroidales_ species. _Proc. Natl Acad. Sci. USA_ 113, 3627–3632 (2016). Article ADS CAS PubMed PubMed Central
Google Scholar * Wexler, A. G. et al. Human symbionts inject and neutralize antibacterial toxins to persist in the gut. _Proc. Natl Acad. Sci. USA_ 113, 3639–3644 (2016). Article ADS
CAS PubMed PubMed Central Google Scholar * Chatzidaki-Livanis, M. et al. Gut symbiont _Bacteroides fragilis_ secretes a Eukaryotic-like ubiquitin protein that mediates intraspecies
antagonism. _mBio_. 8, e01902–17 (2017). * Shumaker, A. M., McEneany, V. L., Coyne, M. J., Silver, P. A. & Comstock, L. E. Identification of a fifth antibacterial toxin produced by a
single _Bacteroides fragilis_ strain. _J. Bacteriol._ 201, e00577–18 (2019). * McEneany, V. L., Coyne, M. J., Chatzidaki-Livanis, M. & Comstock, L. E. Acquisition of MACPF
domain-encoding genes is the main contributor to LPS glycan diversity in gut _Bacteroides_ species. _Isme J._ 12, 2919–2928 (2018). Article CAS PubMed PubMed Central Google Scholar *
Roelofs, K. G., Coyne, M. J., Gentyala, R. R., Chatzidaki-Livanis, M. & Comstock, L. E. _Bacteroidales_ secreted antimicrobial proteins target surface molecules necessary for gut
colonization and mediate competition In Vivo. _mBio._ 7, e01055–16 (2016). * Chatzidaki-Livanis, M., Coyne, M. J. & Comstock, L. E. An antimicrobial protein of the gut symbiont
_Bacteroides fragilis_ with a MACPF domain of host immune proteins. _Mol. Microbiol._ 94, 1361–1374 (2014). Article CAS PubMed PubMed Central Google Scholar * Bao, Y. et al. A common
pathway for activation of host-targeting and bacteria-targeting toxins in human intestinal bacteria. _mBio_. 12, e0065621 (2021). * Evans, J. C. et al. A proteolytically activated
antimicrobial toxin encoded on a mobile plasmid of _Bacteroidales_ induces a protective response. _Nat. Commun._ 13, 4258 (2022). * Matano, L. M., Coyne, M. J., Garcia-Bayona, L. &
Comstock, L. E. Bacteroidetocins target the essential outer membrane protein BamA of _Bacteroidales_ symbionts and pathogens. _mBio_. 12, e0228521 (2021). * Jiang, K. et al. _Bacteroides
fragilis_ ubiquitin homologue drives intraspecies bacterial competition in the gut microbiome. _Nat. Microbiol._ 9, 70–84 (2024). Article CAS PubMed Google Scholar * Schwaim, N. D. III,
Townsend, G. E. II & Groisman, E. A. Prioritization of polysaccharide utilization and control of regulator activation in _Bacteroides thetaiotaomicron_. _Mol. Microbiol._ 104, 32–45
(2017). Article Google Scholar * Pudlo, N. A. et al. Symbiotic human gut bacteria with variable metabolic priorities for host mucosal glycans. _mBio_. 6, e01282–15 (2015). * White, P. et
al. Exploitation of an iron transporter for bacterial protein antibiotic import. _Proc. Natl Acad. Sci. USA_ 114, 12051–12056 (2017). Article ADS CAS PubMed PubMed Central Google
Scholar * Gomez-Santos, N., Glatter, T., Koebnik, R., Swiatek-Polatynska, M. A. & Sogaard-Andersen, L. A TonB-dependent transporter is required for secretion of protease PopC across the
bacterial outer membrane. _Nat. Commun._ 10, 1360 (2019). * Kleanthous, C. Swimming against the tide: progress and challenges in our understanding of colicin translocation. _Nat. Rev.
Microbiol._ 8, 843–848 (2010). Article CAS PubMed Google Scholar * Silale, A. & van den Berg, B. TonB-dependent transport across the bacterial outer membrane. _Annu. Rev. Microbiol._
77, 67–88 (2023). Article CAS PubMed Google Scholar * Noinaj, N. et al. TonB-dependent transporters: regulation, structure, and function. _Annu. Rev. Microbiol._ 64, 43–60 (2010).
Article CAS PubMed PubMed Central Google Scholar * Braun, V., Ratliff, A. C., Celia, H. & Buchanan, S. K. Energization of outer membrane transport by the ExbB ExbD molecular motor.
_J. Bacteriol._ 205, e0003523 (2023). * Emms, D. M. & Kelly, S. OrthoFinder: phylogenetic orthology inference for comparative genomics. _Genome Biol._ 20, 238 (2019). * Terrapon, N. et
al. PULDB: the expanded database of polysaccharide utilization loci. _Nucleic Acids Res._ 46, D677–D683 (2018). Article CAS PubMed Google Scholar * Li, J. et al. An integrated catalog of
reference genes in the human gut microbiome. _Nat. Biotechnol._ 32, 834–841 (2014). Article CAS PubMed Google Scholar * Liu, Q.-Q. et al. Enterotoxigenic _Bacteroides fragilis_ induces
the stemness in colorectal cancer via upregulating histone demethylase JMJD2B. _Gut. Microbe._ 12, 1788900 (2020). * Hecht, A. L., Casterline, B. W., Choi, V. M. & Wardenburg, J. B. A
two-component system regulates _Bacteroides fragilis_ toxin to maintain intestinal homeostasis and prevent lethal disease. _Cell Host Microbe._ 22, 443–448 (2017). Article CAS PubMed
PubMed Central Google Scholar * Franco, A. A. et al. Molecular evolution of the pathogenicity island of enterotoxigenic _Bacteroides fragilis_ strains. _J. Bacteriol._ 181, 6623–6633
(1999). Article CAS PubMed PubMed Central Google Scholar * Liu, C. et al. Enlightening the taxonomy darkness of human gut microbiomes with a cultured biobank. _Microbiome_ 9, 119
(2021). * Garcia-Bayona, L. & Comstock, L. E. Streamlined genetic manipulation of diverse _Bacteroides_ and _Parabacteroides_ isolates from the human gut microbiota. _mBio_. 10,
e01762–19 (2019). * Parker, A. C., Seals, N. L., Baccanale, C. L. & Rocha, E. R. Analysis of six _tonB_ gene homologs in _Bacteroides fragilis_ revealed that _tonB3_ is essential for
survival in experimental intestinal colonization and intra-abdominal infection. _Infect. Immun._ 90, e0046921 (2022). * Jiang, K. et al. A strategy to enhance the insecticidal potency of
Vip3Aa by introducing additional cleavage sites to increase its proteolytic activation efficiency. _Eng. Microbiol._ 3, 100083 (2023). Article CAS Google Scholar * Li, R., Li, A., Zhang,
Y. & Fu, J. The emerging role of recombineering in microbiology. _Eng. Microbiol._ 3, 100097 (2023). Article CAS Google Scholar * Goodman, A. L. et al. Identifying genetic
determinants needed to establish a human gut symbiont in its habitat. _Cell Host Microbe._ 6, 279–289 (2009). Article CAS PubMed PubMed Central Google Scholar * Andrew, F. J., Hicks, L.
D. & Minnick, M. F. A system for transposon mutagenesis of _Bartonella bacilliformis_. _J. Microbiol. Methods_ 203, 106623 (2022). * Jiang, K. et al. Functional characterization of
Vip3Aa from _Bacillus thuringiensis_ reveals the contributions of specific domains to its insecticidal activity. _J. Biol. Chem._ 299, 103000 (2023). * Punjani, A., Rubinstein, J. L., Fleet,
D. J. & Brubaker, M. A. cryoSPARC: algorithms for rapid unsupervised cryo-EM structure determination. _Nat. Methods_ 14, 290–296 (2017). Article CAS PubMed Google Scholar *
Pettersen, E. F. et al. UCSF chimera − a visualization system for exploratory research and analysis. _J. Comput. Chem._ 25, 1605–1612 (2004). Article CAS PubMed Google Scholar * Emsley,
P. & Cowtan, K. _Coot_: model-building tools for molecular graphics. _Acta Crystallogr. Sect. D. Struct. Biol._ 60, 2126–2132 (2004). Article ADS Google Scholar * Liebschner, D. et
al. Macromolecular structure determination using X-rays, neutrons and electrons: recent developments in _Phenix_. _Acta Crystallogr. Sect. D. Struct. Biol._ 75, 861–877 (2019). Article ADS
CAS Google Scholar * Wang, K. et al. Microbial-host-isozyme analyses reveal microbial DPP4 as a potential antidiabetic target. _Science_ 381, eadd5787 (2023). * Blanco-Míguez, A. et al.
Extending and improving metagenomic taxonomic profiling with uncharacterized species using MetaPhlAn 4. _Nat. Biotechnol._ 41, 1633–1644 (2023). Article PubMed PubMed Central Google
Scholar * Truong, D. T., Tett, A., Pasolli, E., Huttenhower, C. & Segata, N. Microbial strain-level population structure and genetic diversity from metagenomes. _Genome Res._ 27,
626–638 (2017). Article CAS PubMed PubMed Central Google Scholar Download references ACKNOWLEDGEMENTS We thank Dr. Andrew L. Goodman (Yale University) for technical supports and Drs.
Chun-Jun Guo (Cornell University) and Yongjian Huang (Vanderbilt University) for discussion and careful review of the manuscript. The cryo-EM data were collected at Biomedical Research
Center for Structural Analysis, Shandong University, and we thank Drs. Lei Qi and Dongfang He for their help of data collection. We are grateful to Dianli Zhao and Kang Li (Cryo-EM facility
for Marine Biology, Laoshan laboratory) for their help of cryo-EM sample screening. We also thank Yuyu Guo, Sen Wang, Jingyao Qu, Xiaoju Li, Haiyan Sui and Chengjia Zhang from the core
facilities for life and environmental sciences, SKLMT of Shandong University for their assistance in laser-scanning confocal microscopy and TEM experiments. The present study was supported
by the National Key R&D Program of China (grant no. 2022YFA1304200 to X.G.), the National Natural Science Foundation of China (grant nos. 32122007 to X.G. and 323B2003 to W.X.L.), the
Shandong Provincial Natural Science Foundation (grant nos. ZR2021JQ09 and ZR2023ZD58 to X.G.) and the Taishan Young Scholars Program (no. tsqn202408046 to K.J.). AUTHOR INFORMATION Author
notes * These authors contributed equally: Ming Tong, Jinghua Xu, Weixun Li and Kun Jiang. AUTHORS AND AFFILIATIONS * State Key Laboratory of Microbial Technology, Shandong University,
Qingdao, 266237, China Ming Tong, Jinghua Xu, Weixun Li, Kun Jiang, Yan Yang, Zhe Chen, Xuyao Jiao, Xiangfeng Meng, Mingyu Wang, Shuang-Jiang Liu & Xiang Gao * NHC Key Laboratory of
Digestive Diseases, Division of Gastroenterology and Hepatology, Renji Hospital, School of Medicine, Shanghai Jiao Tong University, Shanghai Institute of Digestive Disease, Shanghai Cancer
Institute, Shanghai, 200001, China Jie Hong * Institute of Evolution and Marine Biodiversity, KLMME, Ocean University of China, Qingdao, 266003, China Hongan Long * State Key Laboratory of
Microbial Resources, Institute of Microbiology, Chinese Academy of Sciences, Beijing, 100101, China Shuang-Jiang Liu * Department of Microbial Pathogenesis and Microbial Sciences Institute,
Yale University School of Medicine, New Haven, CT, 06536, USA Bentley Lim Authors * Ming Tong View author publications You can also search for this author inPubMed Google Scholar * Jinghua
Xu View author publications You can also search for this author inPubMed Google Scholar * Weixun Li View author publications You can also search for this author inPubMed Google Scholar * Kun
Jiang View author publications You can also search for this author inPubMed Google Scholar * Yan Yang View author publications You can also search for this author inPubMed Google Scholar *
Zhe Chen View author publications You can also search for this author inPubMed Google Scholar * Xuyao Jiao View author publications You can also search for this author inPubMed Google
Scholar * Xiangfeng Meng View author publications You can also search for this author inPubMed Google Scholar * Mingyu Wang View author publications You can also search for this author
inPubMed Google Scholar * Jie Hong View author publications You can also search for this author inPubMed Google Scholar * Hongan Long View author publications You can also search for this
author inPubMed Google Scholar * Shuang-Jiang Liu View author publications You can also search for this author inPubMed Google Scholar * Bentley Lim View author publications You can also
search for this author inPubMed Google Scholar * Xiang Gao View author publications You can also search for this author inPubMed Google Scholar CONTRIBUTIONS Conceptualization, X.G.;
methodology, X.G., M.T., J.H.X., W.X.L., K.J., Y.Y., Z.C., X.Y.J, X.F.M., M.Y.W., J.H., H.A.L., S.J.L., and B.L.; investigation, M.T., J.H.X., W.X.L., K.J., Y.Y., Z.C., and X.Y.J.;
validation, M.T., J.H.X., W.X.L., K.J., and Z.C.; writing—original draft, X.G., M.T., J.H.X., W.X.L., K.J., X.Y.J, and B.L.; writing—review and editing, X.G., W.X.L., M.T., K.J., J.H.X., and
B.L.; resources, X.G., X.F.M., M.Y.W., J.H., B.L., and S.J.L.; funding acquisition, X.G., W.X.L., and K.J.; supervision, X.G. CORRESPONDING AUTHOR Correspondence to Xiang Gao. ETHICS
DECLARATIONS COMPETING INTERESTS The authors declare no competing interests. PEER REVIEW PEER REVIEW INFORMATION _Nature Communications_ thanks the anonymous reviewers for their contribution
to the peer review of this work. A peer review file is available. ADDITIONAL INFORMATION PUBLISHER’S NOTE Springer Nature remains neutral with regard to jurisdictional claims in published
maps and institutional affiliations. SUPPLEMENTARY INFORMATION SUPPLEMENTARY INFORMATION PEER REVIEW FILE REPORTING SUMMARY SOURCE DATA SOURCE DATA RIGHTS AND PERMISSIONS OPEN ACCESS This
article is licensed under a Creative Commons Attribution-NonCommercial-NoDerivatives 4.0 International License, which permits any non-commercial use, sharing, distribution and reproduction
in any medium or format, as long as you give appropriate credit to the original author(s) and the source, provide a link to the Creative Commons licence, and indicate if you modified the
licensed material. You do not have permission under this licence to share adapted material derived from this article or parts of it. The images or other third party material in this article
are included in the article’s Creative Commons licence, unless indicated otherwise in a credit line to the material. If material is not included in the article’s Creative Commons licence and
your intended use is not permitted by statutory regulation or exceeds the permitted use, you will need to obtain permission directly from the copyright holder. To view a copy of this
licence, visit http://creativecommons.org/licenses/by-nc-nd/4.0/. Reprints and permissions ABOUT THIS ARTICLE CITE THIS ARTICLE Tong, M., Xu, J., Li, W. _et al._ A highly conserved SusCD
transporter determines the import and species-specific antagonism of _Bacteroides_ ubiquitin homologues. _Nat Commun_ 15, 8794 (2024). https://doi.org/10.1038/s41467-024-53149-w Download
citation * Received: 01 July 2024 * Accepted: 01 October 2024 * Published: 10 October 2024 * DOI: https://doi.org/10.1038/s41467-024-53149-w SHARE THIS ARTICLE Anyone you share the following
link with will be able to read this content: Get shareable link Sorry, a shareable link is not currently available for this article. Copy to clipboard Provided by the Springer Nature
SharedIt content-sharing initiative