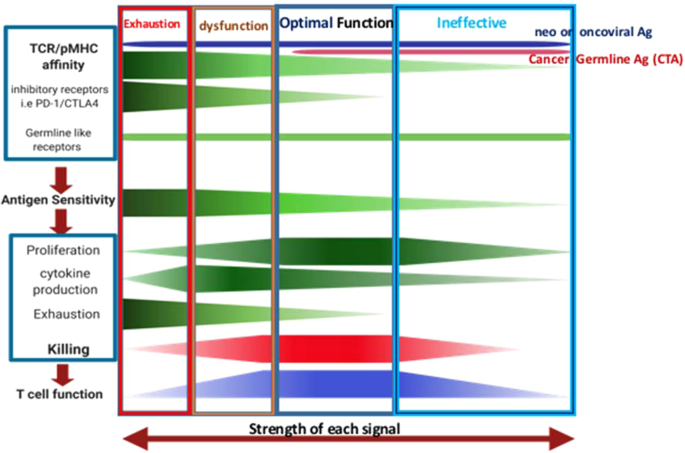
- Select a language for the TTS:
- UK English Female
- UK English Male
- US English Female
- US English Male
- Australian Female
- Australian Male
- Language selected: (auto detect) - EN
Play all audios:
ABSTRACT In this review, we will highlight the importance of cancer germline antigen-specific cytotoxic CD8+ T lymphocytes (CTL) and the factors affecting antitumor CTL responses. In light
of cancer immunotherapy, we will emphasis the need to further understand the features, characteristics, and actions of modulatory receptors of human cancer germline-specific CTLs, in order
to determine the optimal conditions for antitumor CTL responses. SIMILAR CONTENT BEING VIEWED BY OTHERS CYTOTOXIC CD8+ T CELLS IN CANCER AND CANCER IMMUNOTHERAPY Article Open access 15
September 2020 ΓΔ T CELLS ARE EFFECTORS OF IMMUNOTHERAPY IN CANCERS WITH HLA CLASS I DEFECTS Article Open access 11 January 2023 THE T CELL RECEPTOR REPERTOIRE OF TUMOR INFILTRATING T CELLS
IS PREDICTIVE AND PROGNOSTIC FOR CANCER SURVIVAL Article Open access 02 July 2021 INTRODUCTION Cancer is one of the leading causes of mortality worldwide, with lung, colorectal, and liver
cancer being the most common types of tumors.1,2 The small success rates in certain types of patients treated with current conventional cancer therapy such as radiotherapy and chemotherapy
is contributed to not only by the multidrug resistance of cancer cells, but also by the low immunogenicity of tumors and the suppressive tumor microenvironment to immune cells.3,4,5
Therefore, cancer immunotherapy in which treatment is administered to patients to promote or restore the priming and antitumor activities of T cells has been of increasing interest. It is
likely that combining immunotherapy with conventional treatment would allow better antitumor responsiveness and lead to cancer elimination. Numerous clinical studies have established that
the presence and enrichment of tumor-infiltrating T lymphocytes (TILs) in solid tumors is highly associated with better outcome in cancer patients.6,7,8,9,10 Importantly, tumors with
enriched TILs (hot tumors) are highly receptive to immunotherapy compared with TILs-diminished tumors (cold tumors).11,12 In addition, studies using murine models further showed that
adoptive transfer of T cells into tumor bearing mice exhibited significant tumor regression and improved overall response rate.13,14,15,16 Antitumor T-cell responses are critical to impeding
cancer progression and can be targeted for therapy: clinical trials that block inhibitory receptors (IRs), such as PD-1 and CTLA-4, have shown great success. However, not all patients
respond to the current immune-checkpoint inhibitors.17,18,19 In addition, the risk of causing autoimmune disease is high—largely due to the nonspecific nature of the approaches.20,21,22 New
therapies that target cancer-specific immune cells are needed to improve patient outcomes, but our understanding of how cancer-specific T-cell responses go awry in patients is limited. To
make progress, we must delineate the diverse mechanisms exploited by evolving tumors that cause T cells to lose their ability to detect and eliminate cancer cells in specific patients, and
determine to what extent these functions can be restored. Furthermore, it is key to investigate how potential toxic effects may be controlled and T-cell exhaustion could be prevented. The
presence of T cells with impaired function correlates with poor patient prognosis in several types of cancers and chronic infections.22 Effective immunotherapies can augment cancer-specific
immunity by improving the effector cell functions of cytotoxic T cells or the antigen presentation capacity of antigen presenting cells (APC) against cancer cells. However, multiple factors
will affect the quality of T-cell responses such as antigenic variation, the tumor microenvironment, and host genetics.23,24,25 Little is known in human settings about how these factors
influence the net function of cancer-specific cytotoxic T lymphocytes (CTL), or the extent to which they synergize to silence cancer-specific CTLs in patients. A clearer understanding of the
biologic and molecular aspects of CTL dysfunction in large patient cohorts is needed. We must not only develop more efficient strategies for checkpoint blockade, but also develop new
therapies (combination or monotherapies) to deliver specific T-cell immunotherapy with optimal activation to prevent T-cell exhaustion or host pathology. The current hypothesis is that
optimal cancer-specific CTL function is determined by antigen sensitivity, which is influenced by T-cell receptor (TCR) affinity to different tumor antigens peptide loaded onto MHC class Ia
molecules and/or nonclassical MHC class Ib molecules, such as HLA-E. In contrast to MHC class Ia, HLA-E are often highly upregulated in tumors. Furthermore, cancer-specific CTL function is
modulated by IRs and co-stimulatory receptors that accumulate during disease progression. Disturbance of the fine balance of these factors alters optimal T-cell function and contributes to
cancer development and metastases (Fig. 1). TUMOR ANTIGEN-TCR RECOGNITION AND ITS USAGE IN CANCER IMMUNOTHERAPY CANCER GERMLINE (TESTIS) ANTIGENS (CTA) Among the different classifications of
cancer antigens, the primary classes that are at present widely used in cancer immunotherapy research are cancer neoantigens, oncoviral antigens, and CTA. Neoantigens are a class of
antigens that is completely foreign and absent in normal human tissues and cells while present exclusively on cancer cells. In contrast, oncoviral antigens are peptides expressed by virally
associated tumors, such as oncoprotein E7 of human papillomavirus 16.26,27 Neoantigen-specific and oncoviral antigen-specific T cells bearing TCRs of full range of affinities to pMHC,
especially high affinity T cells. Following chronic stimulation of neoantigen-/oncoviral antigen-specific T cells, they can become exhausted and dysregulated, with expression of IRs such as
PD-1 and CTLA-4.28,29 CTAs are a class of antigens not typically expressed by normal cells outside of the testes—cells of which do not express MHC class I, nor present antigens to CD8+ T
cells. However, CTAs are frequently expressed on cancer cells.30 Human CTAs, such as synovial sarcoma X-2 (SSX-2), New York-esophageal squamous cell carcinoma-1 (NY-ESO-1), and melanoma
associated antigen A-1 or A-3 are over-expressed in different human cancers such as in melanoma and lung cancer.31,32,33 CTA-specific T cells are frequently detected in cancer patients and
contribute to tumor regression. Several studies using CTA vaccine strategy have demonstrated correlation between increased CTA-specific T-cell responses with progression-free survival.34,35
This benefit is further confirmed by another study showing that CTA+ patients receiving adoptive transfer of autologous T cells transduced with NY-ESO-1-specific TCR exhibited better
clinical responses.36 However, due to the nature of CTA antigens, TCRs used by T cells specific to these antigens possibly have lower affinity for pMHC and exhibit weaker antigen sensitivity
or lower functional avidity. Although CTA-specific T cells are less likely to express high level of activation-induced IRs,37,38,39 some of them can demonstrate upregulation of
germline-like IR expression, such as CD94/NKG2a with compromised antitumor activity.40 Exploring how to improve antitumor activities of CTA-specific CTLs could provide profound and broader
clinical benefits to cancer patients. ENGINEERED TCR-T CELLS STRATEGY FOR CANCER IMMUNOTHERAPY Engineered cancer-specific TCR-T cells is one of the current immunotherapy strategies designed
with the aim of improving antitumor T-cell responses in patients. It is a directed therapy using TCR of T cells that specifically target/recognize cells expressing particular antigen such as
CTAs. Careful selection of TCRs that selectively recognize cancer pMHC molecules on tumors but not on normal tissues—form a vital aspect of this approach.41,42 In humans, the TCR diversity
is estimated to be up to 1018 variations, due to the random DNA rearrangement of each of the TCR chain and in the random pairing of TCR α and β chains (as well as γδ TCRs) on individual T
cell.43,44,45 Each TCR recognizes specific antigen, such as viral, bacterial, or oncogenic antigens bounded on specific MHC type (with diverse MHC alleles present). Considering the large
variety in TCR repertoire and antigen specificities, the canonical method of selecting the correct TCRs for engineering is through sequencing TCR α chain and β chain mRNAs from in
vitro-cultured T cells of cancer patients, or using algorithmic predictions based of the CDR3 binding region of TCRs.42,46,47,48 The highly specific and directed nature of TCR–pMHC
interaction prevent engineered TCR-T cell from targeting cells that do not express the specific tumor antigen and MHC restriction. Significant success has been highlighted in various
preclinical and clinical studies in human with different types of cancer including synovial, myeloma, colorectal carcinoma, and melanoma. A study in 2006 using engineered MART-1-specific
TCR-T cells demonstrated significant tumor regression in treated melanoma patients.49 Another study by Rosenberg et al. has shown that more than 19% of metastatic melanoma patients receiving
adoptive transfer of gp100-specific TCR-T cells exhibited strong antitumor T-cell responses posttreatment.50 Various other studies have gone into clinical trials including TCR-T cells that
target CTA pMHC molecules. Treatment using TCR-T cells targeting HLA-A0201-restricted NY-ESO-1 cancer antigen exhibited improved overall clinical response rate in more than 50% of melanoma,
synovial or multiple myeloma patients, with no off-site toxicities observed.36,51,52 Importantly, prolonged presence of TCR-T cells was detected in a clinical trial using HLA-A2-restricted
MAGE-A4-specific TCR-T cells in treated esophageal cancer patients, with three patients having minimal lesions at 27 months posttreatment.53 These clinical successes highlighted the
importance and potential benefits of using precise and directed cancer immunotherapy strategy specifically targeting tumor antigens-expressing cancer cells. CANCER IMMUNE EVASION AND THE
IMPACTS ON CTL RESPONSES However, cancers are now well-established to employ diverse mechanisms to escape antitumor immunity. Among the known escape mechanisms include having reduced
TCR/pMHC affinity, downregulation of the pMHC class Ia surface expression, chronic antigen exposure, and elevated expression of IRs such as PD-1. Particularly for CTLs, it can result in
having impaired activation and proliferation, decreased production of inflammatory cytokines, and limited or inefficient cancer cell killing. These factors contribute to anergic and
exhaustive state of T cells in tumor, preventing CTLs from performing optimally in their antitumor functions (Fig. 1). DOWNREGULATION OF MHC CLASS IA LEADS TO COMPROMISED T-CELL PRIMING,
ACTIVATION, AND INEFFECTIVE RESPONSES One of the first known hallmarks of cancer cells is their ability to downregulate MHC class Ia expression on their cell surface. This observation is
well-established in multiple cancer types, including in melanoma and lung cancers.54,55 The downregulation of MHC class Ia expression is thought to involve the dysregulation of antigen
processing machinery such as transporter-associated protein (TAP), tapasin, and calnexin. Resultant inefficient antigen processing leads to reduced presentation of stabilized pMHC at the
cancer cell surface.55 In turn, the lack of pMHC presentation can impair cancer-specific CTLs’ ability to identify and kill cancer cells, as the pMHC downregulation masks the dysregularity
and presence of oncogenic cells. Interestingly, our group has recently shown that this downregulation was not only observed on tumor cells, but also on the APCs in the tumor microenvironment
such as on the tumor-residing CD141+ conventional dendritic cells (cDC) and macrophages.40 As APCs are critical for mediating adaptive immune responses, the overall downregulation of pMHC
in the tumor microenvironment may lead to a compromised T-cell priming and activation. EXHAUSTED T CELLS EXHIBIT ELEVATED INHIBITORY RECEPTOR EXPRESSION It is now well-established that
chronic tumor antigen stimulation and the immunosuppressive tumor microenvironment have driven T cells to exhaustion. Exhausted TILs exhibit upregulated expression of multiple IRs such as
PD-1, CTLA-4, and Tim3. The immunosuppressive tumor microenvironment could present the necessary ligands to these IRs which, in turn, impairs, limits, and exhausts antitumor T-cell
activities. Due to their elevated presence in cancer, the primary focus of current immunotherapy has been on immune-checkpoint blockade treatment, in order to block the inhibitory effects of
IRs so as to allow T cells to recover their antitumor responses. CTLA-4 was the first IR found to negatively affect TILs’ functions. In healthy condition, CTLA-4 is an IR inducible upon
T-cell activation. CTLA-4 recognizes B7-1 (CD80) and B7-2 (CD86), the same ligands that can be recognized by the co-stimulatory receptor CD28. In contrast to CD28, CTLA-4 negatively
regulates the activation of T cells.56 In preclinical cancer models, CTLA-4 blocking antibody treatment in mice was found to successfully prevent tumor establishment and also promote tumor
rejection.57,58 However, in human clinical studies, fully humanized anti-CTLA-4 treatment, such as ipilimumab, was shown to increase the risk of autoimmune diseases such as colitis in
certain treated cancer patients.59,60 PD-1 is well-established to contribute to T-cell exhaustion in cancer. PD-1 interact with its ligands, PD-L1 or PD-L2, and can mediate the epigenetic
transformation of T cells, creating hyporesponsive a T-cell phenotype that resembles senescence, leading to cell cycle arrest and reduced cytolytic activities of CTLs.61,62,63 Activation of
PD-1 on T cells phosphorylates the downstream inhibitory molecule SHP-1, a protein known to negatively affect the cascade signaling of TCR by interfering with the phosphorylation of ZAP70
and PKCθ, impairing—among other factors—the calcium utilization of T cells.64,65 In tumors, both PD-1 and PD-L1 are known to be upregulated on TILs and cancer cells. Preclinical studies
blocking the PD-1/PD-L1 axis demonstrated increased overall T-cell activation, antitumor T-cell responses, and inhibition of murine tumor growth.66,67 In humans, fully humanized anti-PD-1
antibodies such as pembrolizumab and anti-PD-L1 antibodies such as atezolizumab have demonstrated significant tumor regression, such as in melanoma, lung and renal carcinoma
patients.68,69,70 Furthermore, it was found that metastatic patients with high PD-L1 expression treated with pembrolizumab exhibited active proliferation of cytotoxic TILs, with decreasing
tumor size.11 It is therefore not surprising that pembrolizumab was successfully certified by the US FDA in 2014 for the treatment of metastatic and late stage cancer patients. However, it
is worth noting that treatment with anti-PD-1 or anti-PD-L1 failed to improve outcome in certain cancer patients and cancer types. It is therefore likely that other mechanisms could be at
play, either by compensating loss of the PD-1/PD-L1 axis or by imposing inhibitory effects on TILs independently from influence of PD-1/PD-L1. OTHER KNOWN INHIBITORY RECEPTORS Other IRs have
also been discussed as potential targets for cancer immunotherapy, including Tim3, BTLA, and TIGIT. Tim3 was originally found to suppress the effector Th1 responses in murine model of
multiple sclerosis.71 In chronic viral infection, Tim3 expression is highly persistent and is associated with the inflammatory regulation of T cells.72 In cancer, Tim3+ TILs in lung cancer
patients exhibited significant defects in cytokine production and T-cell proliferation.3,73 Furthermore, co-expressed Tim3+ PD-1+ TILs were demonstrated to represent a deeply exhausted
T-cell population, with a strong association to tumor progression such as in prostate, colorectal, and hepatocellular carcinoma.74,75 In contrast, TIGIT was found to inhibit spontaneous
T-cell activation, and is primarily expressed following T-cell activation and on memory T cells.76 BTLA is also expressed following T-cell activation and phosphorylation of this receptor is
known to interfere with the downstream TCR-mediated signaling cascade.77 A recent study in our group has shown that the expression of BTLA, TIGIT, KLRG-1, and 2B4 is not enriched on TILs
compared with their counterparts in peripheral blood of cancer patients.78 Most importantly, we found that co-expression of specific IRs including Tim3 and PD-1 is highly prevalent on CD8+
TILs across different cancer types, such as in lung, kidney, and breast cancers. This suggests that certain IRs are likely more favored in the tumor microenvironment to suppress antitumor
CTLs responses. The co-expression of multiple IRs on TILs indicates the possibility that mono-immunotherapy targeting a single IR might not be enough, as there could be compensatory
inhibitory mechanisms taking place. Therefore, as others in the field have suggested, a combinatory immune-checkpoint blockade immunotherapy strategy could provide better clinical responses
in cancer patients. CO-STIMULATORY RECEPTORS AND THEIR IMPORTANCE IN ANTITUMOR CTLS RESPONSES In contrast to IRs, co-stimulatory receptors of T cells act as a secondary signal for an
effective TCR-mediated T-cell activation and immune response. Current well-established co-stimulatory receptors of T cells include CD28, 4-1BB, and ICOS. It is known that an overall
activation of T cells without co-signaling can lead to T-cell anergy, a state of hypo-responsiveness and growth arrest, and contribute toward dysregulated immune tolerance and impaired
effector functions.79 CD28 is the best characterized co-stimulatory receptor of activated T cells, acquired only following activation. Its ligation to CD80 or CD86 promotes T-cell
proliferation, cytokine production, cell survival, and stabilization of cytokine mRNAs.80,81,82 Studies using a CD28-deficient murine model highlighted the necessity of CD28. In this study,
its absence was shown to result in an ineffective effector response and a lack of viral control.83 In addition, adoptive transfer of CD80-transfectant tumor cells into mice produced a strong
CD28-responsive CTL-mediated immune responses and tumor rejection.84 As CD28 and CTLA-4 share the same ligands of CD80 and CD86,56 these two receptors heavily compete for the same ligand
recognition and therefore can affect and define the overall activatory or inhibitory outcome of T-cell activities. 4-1BB: similarly to CD28, both 4-1BB and ICOS are only expressed by T cells
following T-cell activation. For 4-1BB, its ligation to 4-1BBL, expressed mainly on dendritic cells, allows conducive effector CTL responses.85,86 A preclinical study using 4-1BB agonist in
combination with anti-PD-1 treatment in a tumor bearing murine model demonstrated an elevated IFNγ response, mediated primarily by the effector memory CTLs.87 In a human setting, a phase I
clinical trial with 4-1BB agonist (utomilumab) showed not only its safety, but also that the agonistic antibody induced higher overall response rates in melanoma patients.88 However, <4%
of nonmelanoma cancer patients with solid tumors treated with this regiment exhibited improved responses. Another anti-4-1BB antibody (urelumab) used in clinical trial for treatment of
advanced cancers unfortunately induced severe liver toxicity in some of the patients treated, likely due to nonspecific immune cells activation that may cause tissue damage.89 ICOS, also
known as CD278, is present on activated T cells and interacts with its ligand, ICOS-L, expressed mainly on APCs. Activation of ICOS was first known to enhanced T-cell proliferation.90,91
Interestingly, preclinical studies demonstrated that ICOS was upregulated following anti-CTLA-4 treatment in tumor bearing murine models, suggesting that it could be an important mediator of
better T-cell responses in the anti-CTLA-4 treated patients.92,93 GERMLINE-LIKE RECEPTORS AND THEIR POTENTIAL ROLE IN OPTIMAL CTL RESPONSES Progression of CTLs into an exhausted or
dysfunctional state is mainly caused by chronic antigen exposure, inefficient T-cell activation and the upregulation of activation-associated IR expression, such as PD-1. The
immunosuppressive tumor microenvironment nudges TILs into an exhausted state, and prevents optimal antitumor T-cell responses (Fig. 1). Constitutive markers of T cells include germline-like
receptors such as CD103 and NKG2a, which, with their ligands continually presented robustly on most cancer cells, are much less studied for their function as co-stimulatory or co-IR for
CTLs. These germline-like receptors could be critical to understanding the optimal conditions for effective antitumor T-cell immune responses. CD103, MORE THAN A TISSUE RESIDENCY MARKER
CD103, also known as integrin alpha E, was first discovered as a T-cell marker for tissue retention and residency, especially in the gut and lung. It forms heterodimeric pairing with
integrin beta 7 to form a complete complex recognized by E-cadherin, a molecule expressed at moderate level primarily by epithelial cells. Interaction between CD103 and E-cadherin allows
attachment of CD103+ immune cells to tissue.94,95 Also termed as CD103+ tissue-resident memory T cells (CD103+ Trm cells), they lack tissue exit markers such as CD62L, CCR7, S1PR1, and
CX3CR1, while upregulating Notch transcription factor and pro-survival molecules such as Bcl-2 and BIRC3.96,97,98,99,100,101,102 CD103 expression is known to be acquired during naive T-cell
development, following initial antigen pMHC contact and in the presence of TGF-β1.103,104 Notably, Batf3-dependent murine DC and human CD1c+ cDC were defined as the primary producer of
TGF-β1 and antigenic contact during the development process.105,106,107 Recently, our group has surprisingly found the presence of matured CD103+ CTA-specific CTLs from the peripheral blood
of cancer patients.108 Most importantly, this unique T-cell population can self-produce activated TGF-β1 through its own membrane β8 expression to sustain its CD103 expression (Fig. 2).
CD103+ T cells were found to help mediate better immune surveillance. For example, enrichment of Trm cells in tissue resulted in faster clearance of influenza murine lung infection and HSV
murine skin infection.96,109 In cancer, murine studies have shown that the presence of CD103 on TILs contributes to elevated T-cell infiltration into solid tumors, with better tumor
control.110,111 Consistent with its known role as a retention marker, our group and others have shown that CD103+ TILs can home and localize better to E-cadherin+ tumor islets.97,108,111
Importantly, we found that they clustered at a higher density within and surrounding the E-cadherin+ tumor islets, compared with the CD103− TILs108 (Fig. 2). In addition, epithelial-derived
cancer cells are also known to over-express E-cadherin on their surface. Altogether, it is therefore likely that CD103+ TILs could play a significant role in inducing better antitumor immune
responses. Interestingly, studies by Mami-Chouaib’s group have demonstrated an accumulation of CD103 molecules within the point of contact between CD103+ T cells and autologous E-cadherin+
cancer cells.112,113 The lack of E-cadherin on cancer cells can also diminish the movement of CD103 into the interface. Surprisingly, they showed that CD103 accumulation does overlap with an
enriched granzyme B presence within the synapse. This suggests the possibility that CD103 could provide additional co-stimulatory signaling for antitumor CTL cytotoxic responses, upon its
interaction with E-cadherin. This notion is supported by other studies associating the enrichment of CD103+ TILs with better clinical outcome in patients with either melanoma, colorectal or
lung cancer.100,114,115 Also, preclinical studies found that the lack of E-cadherin on some melanoma cells can impair the overall antitumor responsiveness of murine CD103+ Trm cells, even
after anti-CTLA-4 and anti-PD-1 treatments.116 However, it was unclear how exactly the presence of CD103 contributes toward tumor control. As mentioned previously, TCR-CTA pMHC recognition
is of low affinity, and this can reduce the overall activation and response of CTA-specific CTLs. Using circulation-derived, TCR-matched CD103+ and CD103− CTA-specific CTLs, our group
recently showed that the presence of CD103 can help to significantly elevate TCR antigen sensitivity.108 In particular, we found that TCR-matched CD103+ CTA-specific CTLs produce and secrete
elevated inflammatory cytokines such as IFNγ compared with the TCR-matched CD103− CTLs. Most importantly, this unique T-cell population exhibits faster recognition of individual cancer
cells and induces rapid antitumor cytotoxicity, primarily through degranulation-mediated cytotoxicity. This form of cytotoxicity is supported by a previous study showing elevated granzyme B
accumulation in the CD103+ T-cell immunological synapse.112,113 Using live cell imaging, we further demonstrated that CD103+ CTA-specific CTLs induce faster E-cadherin+ cancer cell killing
compared with the TCR-matched CD103− CTLs.108 This rapid killing allowed significantly higher numbers of accumulative E-cadherin+ cancer cell deaths over time. The elevated antigen
sensitivity, however, was impaired following either treatment with anti-CD103, or in the absence of E-cadherin on cancer cells. It is therefore very likely that the presence of CD103 on
CTA-specific CTLs and its engagement with E-cadherin on cancer cells help to either improve the rapidness of immunological synapse formation, strengthening the interaction kinetics of
TCR–pMHC binding and/or activate downstream signaling that acts as a co-signal to the TCR-mediated activatory signaling. An efficient antitumor response requires high energy usage,
particularly in producing and trafficking cytokines and cytolytic molecules into the immunological synapse via the action of cytoskeletal activities.117,118 It is therefore not surprising
that our group found that the CD103+ CTA-specific CTLs have elevated glucose consumption over time, with higher maximal capacity to metabolize glucose compared with the TCR-matched CD103−
CTLs.108 However, in the tumor microenvironment, enhanced glucose consumption by cancer cells can deprive TILs of the essential glucose needed for proper immune functioning.119
Interestingly, a recent study by the Kupper group has demonstrated that virus-specific CD103+ Trm cells are highly capable of utilizing exogenous free fatty acids via their mitochondria, and
this contributed toward enforcing protective immunity against virus-infected tissue.120 Importantly, our group has further shown in cancer settings that the CD103+ CTA-specific CTLs have
elevated overall mitochondrial activities and maximal mitochondrial spare capacity.108 This therefore suggests that in the case of glucose-deprived microenvironments such as in cancer,
CD103+ CTA-specific CTLs could compensate the lack of glucose metabolism with other non-glucose metabolisms to meet their high energy demand (Fig. 2). UPREGULATED CD94/NKG2A AND ITS LIGAND
HLA-E IN CANCER Another germline-like receptor that we found highly expressed in cancer is the heterodimeric CD94/NKG2a.40 This receptor was originally found on NK cells, with its first
known function being to limit NK cell cytotoxicity and prevent autoimmunity in healthy tissue.121,122,123,124,125 Our group was able to establish CTA-specific CTL clones with matched TCR,
but different surface expression, such as with either CD103 or CD94/NKG2a.40,108 Using the NKG2a+ and NKG2a− TCR-matched CTA-specific CTLs in a recent study, we found that exposure of
CD94/NKG2a+ CTLs to HLA-E-enriched cancer cells significantly impaired the degranulation-mediated cytotoxicity and the production and secretion of inflammatory cytokines and chemokines such
as IFNγ and CCL-5.40 This was further demonstrated in an ex vivo approach, using freshly isolated CD94/NKG2a+ TILs from HLA-E-enriched tumor tissue. In addition to its effect on killing, the
enriched HLA-E and CD94/NKG2a interaction significantly affected IL-2-dependent T-cell proliferation, in which the production and secretion of IL-2 were detrimentally impaired and the
expression of IL-2 receptor alpha (CD25) was also reduced. Similarly to previous studies, however, the use of anti-NKG2a can reverse the negative effect of NKG2a on antigen-specific CTL
activities.40,126,127 This mechanism is mediated through CD94/NKG2a interaction with the nonclassical MHC class Ib molecule, HLA-E on normal cells. In contrast to the MHC class Ia, numerous
studies have now found that HLA-E as well as HLA-G are enriched in various types of cancer.128,129,130,131 Tumors with either upregulated HLA-E or dually upregulated HLA-E and HLA-G are
associated with poorer clinical outcome in patients.129 Importantly, our group recently demonstrated a significant correlation between HLA-E-enriched tumors with CD94/NKG2a-enriched TILs, in
four different cancer types.40 This suggest that CD94/NKG2a and HLA-E could negatively affect antitumor TILs responses. A recent phase II clinical trial using anti-NKG2a and anti-EGFR to
treat head-and-neck cancer patients showed an improved overall response rate in the majority of patients treated, further highlighting the potential of HLA-E and NKG2a as cancer
immunotherapy target combination.126 However, it was unclear the mechanisms by which HLA-E and CD94/NKG2a could interfere with the antitumor CTL responses. Overall, these examples of
germline-like receptors such as NKG2a and CD103 highlight the significance of identifying and exploring germline-like receptors, particularly of cancer-specific T cells. CYTOKINE DEFECT AND
THE IMPLICATION ON IDENTIFICATION OF CANCER-SPECIFIC CTLS IN PATIENTS Alongside directed cytotoxicity, CTLs function as to produce important inflammatory cytokines such as IFNγ and TNFα.
IFNγ was first discovered to interfere with viral replication in host, and the activation of IFNγ receptor-mediated JAK1/STAT-1 signaling enables the formation of immunoproteasome and the
expression of antigen processing machinery-associated genes such as TAP and tapasin on target cells.132,133,134,135 In contrast, TNFα ligation to TNF receptors 1 and 2 promotes
receptor-ligand-mediated apoptosis events and prevents the expression of antiapoptotic factors such as Bcl-2.136,137 In cancer, preclinical studies using IFNγ-deficient tumor bearing murine
model demonstrated reduced migration of activated T cells into the tumor sites, with enhanced tumor progression.138,139 Surprisingly, a recent study in our group found a sizeable numbers of
SSX-2-specific CTL clones from a cancer patient that lack any IFNγ mRNA and protein expression.140 These IFNγ− CTL clones, however, have normal production of TNFα and other cytokines. In
addition to these clones, a sizeable portion of short-term expanded NY-ESO-1-specific and SSX-2-specific CTL lines from two other cancer patients exhibited hypermethylation of their IFNγ
gene promoter. DNA hypermethylation prevents the recruitment of essential DNA transcription machinery proteins such as DNA polymerase onto the gene, which can limit the level of specific DNA
transcription and protein synthesis in a cell.141,142 The inaccessibility to the IFNγ gene promoter can therefore result in a dysregulated cytokine production and contribute to an
ineffective antitumor CTA-specific CTL responses. In addition to IFNγ, other cytokines deficiency in tandem may also be exhibited and be detrimental to the antitumor responses of
CTA-specific CTLs. Current methods to identify cancer-specific CTLs rely on the IFNγ expression and production following stimulation, such as by using intracellular cytokine assay and
enzyme-linked immunosorbent assay.139,143,144 Our group has recently demonstrated a certain portion of CTA-specific CTLs could be deficient in their IFNγ expression.140 This suggest that the
commonly used techniques might have overlooked a great proportion of CTA-specific CTLs and could potentially lead to unintended biases in cancer-specific T cells analysis. It is therefore
important that more diverse methods that can detect multiple cytokines be considered for a proper identification and analysis of CTA-specific CTLs from cancer patients. CONCLUDING REMARKS
Optimal cancer-specific CTL function is determined by antigen sensitivity, which is influenced by TCR affinity to different tumor antigens peptide loaded onto MHC class Ia molecules and/or
nonclassical MHC molecules such as HLA-E. In contrast to MHC class Ia molecules, HLA-E are highly upregulated on cancer cells and APCs in the cancer microenvironment. Furthermore,
cancer-specific CTL function is modulated by IRs and co-stimulatory receptors that accumulate during disease progression. Disturbance of the fine balance of these factors alters optimal
T-cell function and contributes to cancer development and metastasis. Understanding the quality and key features of human cancer germline antigen-specific T cells in cancer patients and
applying this knowledge to immune therapy should be one of the key priorities to consider. In comparison to exhausted T cells—which are likely unrestorable—this approach may help to restore
dysfunctional as well as push ineffective immune cells to their optimal condition—through manipulating TCR or co-receptors of T cells (Fig. 1). REFERENCES * Ferlay, J. et al. Cancer
incidence and mortality worldwide: sources, methods and major patterns in GLOBOCAN 2012. _Int J. Cancer_ 136, E359–E386 (2015). CAS PubMed Google Scholar * Bray, F. et al. Global cancer
statistics 2018: GLOBOCAN estimates of incidence and mortality worldwide for 36 cancers in 185 countries. _CA Cancer J. Clin._ 68, 394–424 (2018). PubMed Google Scholar * Fourcade, J. et
al. Upregulation of Tim-3 and PD-1 expression is associated with tumor antigen-specific CD8+ T cell dysfunction in melanoma patients. _J. Exp. Med._ 207, 2175–2186 (2010). CAS PubMed
PubMed Central Google Scholar * Binnewies, M. et al. Understanding the tumor immune microenvironment (TIME) for effective therapy. _Nat. Med._ 24, 541–550 (2018). CAS PubMed PubMed
Central Google Scholar * Vasan, N., Baselga, J. & Hyman, D. M. A view on drug resistance in cancer. _Nature_ 575, 299–309 (2019). CAS PubMed PubMed Central Google Scholar * Galon,
J. et al. Type, density, and location of immune cells within human colorectal tumors predict clinical outcome. _Science_ 313, 1960–1964 (2006). CAS PubMed Google Scholar * Kawai, O. et
al. Predominant infiltration of macrophages and CD8(+) T Cells in cancer nests is a significant predictor of survival in stage IV nonsmall cell lung cancer. _Cancer_ 113, 1387–1395 (2008).
CAS PubMed Google Scholar * Ferrone, C. & Dranoff, G. Dual roles for immunity in gastrointestinal cancers. _J. Clin. Oncol._ 28, 4045–4051 (2010). CAS PubMed PubMed Central Google
Scholar * Burton, A. L. et al. Prognostic significance of tumor infiltrating lymphocytes in melanoma. _Am. Surg._ 77, 188–192 (2011). PubMed Google Scholar * Azimi, F. et al.
Tumor-infiltrating lymphocyte grade is an independent predictor of sentinel lymph node status and survival in patients with cutaneous melanoma. _J. Clin. Oncol._ 30, 2678–2683 (2012). PubMed
Google Scholar * Tumeh, P. C. et al. PD-1 blockade induces responses by inhibiting adaptive immune resistance. _Nature_ 515, 568–571 (2014). CAS PubMed PubMed Central Google Scholar *
Ribas, A. et al. Oncolytic virotherapy promotes intratumoral T Cell infiltration and improves anti-PD-1 immunotherapy. _Cell_ 170, 1109–1119.e10 (2017). CAS PubMed PubMed Central Google
Scholar * Boni, A. et al. Adoptive transfer of allogeneic tumor-specific T cells mediates effective regression of large tumors across major histocompatibility barriers. _Blood_ 112,
4746–4754 (2008). CAS PubMed PubMed Central Google Scholar * Klebanoff, C. A. et al. Determinants of successful CD8+ T-cell adoptive immunotherapy for large established tumors in mice.
_Clin. Cancer Res._ 17, 5343–5352 (2011). CAS PubMed PubMed Central Google Scholar * Radvanyi, L. G. et al. Specific lymphocyte subsets predict response to adoptive cell therapy using
expanded autologous tumor-infiltrating lymphocytes in metastatic melanoma patients. _Clin. Cancer Res._ 18, 6758–6770 (2012). CAS PubMed PubMed Central Google Scholar * Besser, M. J. et
al. Adoptive transfer of tumor-infiltrating lymphocytes in patients with metastatic melanoma: intent-to-treat analysis and efficacy after failure to prior immunotherapies. _Clin. Cancer
Res._ 19, 4792–4800 (2013). CAS PubMed Google Scholar * Hodi, F. S. et al. Improved survival with ipilimumab in patients with metastatic melanoma. _N. Engl. J. Med._ 363, 711–723 (2010).
CAS PubMed PubMed Central Google Scholar * Robert, C. et al. Ipilimumab plus dacarbazine for previously untreated metastatic melanoma. _N. Engl. J. Med._ 364, 2517–2526 (2011). CAS
PubMed Google Scholar * Gibney, G. T., Weiner, L. M. & Atkins, M. B. Predictive biomarkers for checkpoint inhibitor-based immunotherapy. _Lancet Oncol._ 17, e542–e551 (2016). CAS
PubMed PubMed Central Google Scholar * Brahmer, J. R. et al. Phase I study of single-agent anti-programmed death-1 (MDX-1106) in refractory solid tumors: safety, clinical activity,
pharmacodynamics, and immunologic correlates. _J. Clin. Oncol._ 28, 3167–3175 (2010). CAS PubMed PubMed Central Google Scholar * Robert, C. et al. Pembrolizumab versus ipilimumab in
advanced melanoma. _N. Engl. J. Med._ 372, 2521–2532 (2015). CAS PubMed Google Scholar * McLane, L. M., Abdel-Hakeem, M. S. & Wherry, E. J. CD8 T cell exhaustion during chronic viral
infection and cancer. _Annu. Rev. Immunol._ 37, 457–495 (2019). CAS PubMed Google Scholar * Crespo, J. et al. T cell anergy, exhaustion, senescence, and stemness in the tumor
microenvironment. _Curr. Opin. Immunol._ 25, 214–221 (2013). CAS PubMed PubMed Central Google Scholar * Pauken, K. E. & Wherry, E. J. Overcoming T cell exhaustion in infection and
cancer. _Trends Immunol._ 36, 265–276 (2015). CAS PubMed PubMed Central Google Scholar * Singer, M. et al. A distinct gene module for dysfunction uncoupled from activation in
tumor-infiltrating T cells. _Cell_ 166, 1500–1511.e9 (2016). CAS PubMed PubMed Central Google Scholar * Mesri, E. A., Feitelson, M. A. & Munger, K. Human viral oncogenesis: a cancer
hallmarks analysis. _Cell Host Microbe_ 15, 266–282 (2014). CAS PubMed PubMed Central Google Scholar * Jiang, T. et al. Tumor neoantigens: from basic research to clinical applications.
_J. Hematol. Oncol._ 12, 93 (2019). PubMed PubMed Central Google Scholar * van Rooij, N. et al. Tumor exome analysis reveals neoantigen-specific T-cell reactivity in an
ipilimumab-responsive melanoma. _J. Clin. Oncol._ 31, e439–e442 (2013). PubMed Google Scholar * Snyder, A. et al. Genetic basis for clinical response to CTLA-4 blockade in melanoma. _N.
Engl. J. Med._ 371, 2189–2199 (2014). PubMed PubMed Central Google Scholar * Whitehurst, A. W. Cause and consequence of cancer/testis antigen activation in cancer. _Annu. Rev. Pharm.
Toxicol._ 54, 251–272 (2014). CAS Google Scholar * dos Santos, N. R. et al. Heterogeneous expression of the SSX cancer/testis antigens in human melanoma lesions and cell lines. _Cancer
Res._ 60, 1654–1662 (2000). PubMed Google Scholar * Hofmann, O. et al. Genome-wide analysis of cancer/testis gene expression. _Proc. Natl Acad. Sci. USA_ 105, 20422–20427 (2008). CAS
PubMed PubMed Central Google Scholar * Greve, K. B. et al. SSX2-4 expression in early-stage non-small cell lung cancer. _Tissue Antigens_ 83, 344–349 (2014). CAS PubMed Google Scholar
* Odunsi, K. et al. Efficacy of vaccination with recombinant vaccinia and fowlpox vectors expressing NY-ESO-1 antigen in ovarian cancer and melanoma patients. _Proc. Natl Acad. Sci. USA_
109, 5797–5802 (2012). CAS PubMed PubMed Central Google Scholar * Dhodapkar, M. V. et al. Induction of antigen-specific immunity with a vaccine targeting NY-ESO-1 to the dendritic cell
receptor DEC-205. _Sci. Transl. Med._ 6, 232ra51 (2014). PubMed PubMed Central Google Scholar * Robbins, P. F. et al. Tumor regression in patients with metastatic synovial cell sarcoma
and melanoma using genetically engineered lymphocytes reactive with NY-ESO-1. _J. Clin. Oncol._ 29, 917–924 (2011). PubMed PubMed Central Google Scholar * Thomas, S. et al. Human T cells
expressing affinity-matured TCR display accelerated responses but fail to recognize low density of MHC-peptide antigen. _Blood_ 118, 319–329 (2011). CAS PubMed Google Scholar * Aleksic,
M. et al. Different affinity windows for virus and cancer-specific T-cell receptors: implications for therapeutic strategies. _Eur. J. Immunol._ 42, 3174–3179 (2012). CAS PubMed PubMed
Central Google Scholar * Schrama, D., Ritter, C. & Becker, J. C. T cell receptor repertoire usage in cancer as a surrogate marker for immune responses. _Semin. Immunopathol._ 39,
255–268 (2017). CAS PubMed Google Scholar * Abd Hamid, M. et al. Enriched HLA-E and CD94/NKG2A interaction limits antitumor CD8(+) tumor-infiltrating T lymphocyte responses. _Cancer
Immunol. Res._ 7, 1293–1306 (2019). PubMed Google Scholar * Debets, R. et al. TCR-engineered T cells to treat tumors: seeing but not touching?. _Semin. Immunol._ 28, 10–21 (2016). CAS
PubMed Google Scholar * Ping, Y., Liu, C. & Zhang, Y. T-cell receptor-engineered T cells for cancer treatment: current status and future directions. _Protein Cell_ 9, 254–266 (2018).
PubMed Google Scholar * Davis, M. M. & Bjorkman, P. J. T-cell antigen receptor genes and T-cell recognition. _Nature_ 334, 395–402 (1988). CAS PubMed Google Scholar *
Nikolich-Zugich, J., Slifka, M. K. & Messaoudi, I. The many important facets of T-cell repertoire diversity. _Nat. Rev. Immunol._ 4, 123–132 (2004). CAS PubMed Google Scholar *
Murugan, A. et al. Statistical inference of the generation probability of T-cell receptors from sequence repertoires. _Proc. Natl Acad. Sci. USA_ 109, 16161–16166 (2012). CAS PubMed PubMed
Central Google Scholar * Nishimura, M. I. et al. T-cell receptor repertoire in tumor-infiltrating lymphocytes. Analysis of melanoma-specific long-term lines. _J. Immunother. Emphas. Tumor
Immunol._ 16, 85–94 (1994). CAS Google Scholar * Howie, B. et al. High-throughput pairing of T cell receptor alpha and beta sequences. _Sci. Transl. Med._ 7, 301ra131 (2015). PubMed
Google Scholar * Pasetto, A. et al. Tumor- and neoantigen-reactive T-cell receptors can be identified based on their frequency in fresh tumor. _Cancer Immunol. Res._ 4, 734–743 (2016). CAS
PubMed PubMed Central Google Scholar * Morgan, R. A. et al. Cancer regression in patients after transfer of genetically engineered lymphocytes. _Science_ 314, 126–129 (2006). CAS
PubMed PubMed Central Google Scholar * Johnson, L. A. et al. Gene therapy with human and mouse T-cell receptors mediates cancer regression and targets normal tissues expressing cognate
antigen. _Blood_ 114, 535–546 (2009). CAS PubMed PubMed Central Google Scholar * Rapoport, A. P. et al. NY-ESO-1-specific TCR-engineered T cells mediate sustained antigen-specific
antitumor effects in myeloma. _Nat. Med._ 21, 914–921 (2015). CAS PubMed PubMed Central Google Scholar * Robbins, P. F. et al. A pilot trial using lymphocytes genetically engineered with
an NY-ESO-1-reactive T-cell receptor: long-term follow-up and correlates with response. _Clin. Cancer Res._ 21, 1019–1027 (2015). CAS PubMed Google Scholar * Kageyama, S. et al. Adoptive
transfer of MAGE-A4 T-cell receptor gene-transduced lymphocytes in patients with recurrent esophageal cancer. _Clin. Cancer Res._ 21, 2268–2277 (2015). CAS PubMed Google Scholar *
Koopman, L. A. et al. Multiple genetic alterations cause frequent and heterogeneous human histocompatibility leukocyte antigen class I loss in cervical cancer. _J. Exp. Med._ 191, 961–976
(2000). CAS PubMed PubMed Central Google Scholar * Leone, P. et al. MHC class I antigen processing and presenting machinery: organization, function, and defects in tumor cells. _J. Natl
Cancer Inst._ 105, 1172–1187 (2013). CAS PubMed Google Scholar * Sansom, D. M. CD28, CTLA-4 and their ligands: who does what and to whom?. _Immunology_ 101, 169–177 (2000). CAS PubMed
PubMed Central Google Scholar * Leach, D. R., Krummel, M. F. & Allison, J. P. Enhancement of antitumor immunity by CTLA-4 blockade. _Science_ 271, 1734–1736 (1996). CAS PubMed Google
Scholar * Kwon, E. D. et al. Manipulation of T cell costimulatory and inhibitory signals for immunotherapy of prostate cancer. _Proc. Natl Acad. Sci. USA_ 94, 8099–8103 (1997). CAS PubMed
PubMed Central Google Scholar * Hodi, F. S. et al. Biologic activity of cytotoxic T lymphocyte-associated antigen 4 antibody blockade in previously vaccinated metastatic melanoma and
ovarian carcinoma patients. _Proc. Natl Acad. Sci. USA_ 100, 4712–4717 (2003). CAS PubMed PubMed Central Google Scholar * Phan, G. Q. et al. Cancer regression and autoimmunity induced by
cytotoxic T lymphocyte-associated antigen 4 blockade in patients with metastatic melanoma. _Proc. Natl Acad. Sci. USA_ 100, 8372–8377 (2003). CAS PubMed PubMed Central Google Scholar *
Agata, Y. et al. Expression of the PD-1 antigen on the surface of stimulated mouse T and B lymphocytes. _Int. Immunol._ 8, 765–772 (1996). CAS PubMed Google Scholar * Pauken, K. E. &
Wherry, E. J. SnapShot: T cell exhaustion. _Cell_ 163, 1038–1038 e1 (2015). CAS PubMed Google Scholar * Patsoukis, N. et al. PD-1 alters T-cell metabolic reprogramming by inhibiting
glycolysis and promoting lipolysis and fatty acid oxidation. _Nat. Commun._ 6, 6692 (2015). CAS PubMed Google Scholar * Zaugg, K. et al. Carnitine palmitoyltransferase 1C promotes cell
survival and tumor growth under conditions of metabolic stress. _Genes Dev._ 25, 1041–1051 (2011). CAS PubMed PubMed Central Google Scholar * Sheppard, K. A. et al. PD-1 inhibits T-cell
receptor induced phosphorylation of the ZAP70/CD3zeta signalosome and downstream signaling to PKCtheta. _FEBS Lett._ 574, 37–41 (2004). CAS PubMed Google Scholar * Kuang, D. M. et al.
Activated monocytes in peritumoral stroma of hepatocellular carcinoma foster immune privilege and disease progression through PD-L1. _J. Exp. Med._ 206, 1327–1337 (2009). CAS PubMed PubMed
Central Google Scholar * Zhang, L., Gajewski, T. F. & Kline, J. PD-1/PD-L1 interactions inhibit antitumor immune responses in a murine acute myeloid leukemia model. _Blood_ 114,
1545–1552 (2009). CAS PubMed PubMed Central Google Scholar * Topalian, S. L. et al. Safety, activity, and immune correlates of anti-PD-1 antibody in cancer. _N. Engl. J. Med._ 366,
2443–2454 (2012). CAS PubMed PubMed Central Google Scholar * Hamid, O. et al. Safety and tumor responses with lambrolizumab (anti-PD-1) in melanoma. _N. Engl. J. Med._ 369, 134–144
(2013). CAS PubMed PubMed Central Google Scholar * Powles, T. et al. MPDL3280A (anti-PD-L1) treatment leads to clinical activity in metastatic bladder cancer. _Nature_ 515, 558–562
(2014). CAS PubMed Google Scholar * Koguchi, K. et al. Dysregulated T cell expression of TIM3 in multiple sclerosis. _J. Exp. Med._ 203, 1413–1418 (2006). CAS PubMed PubMed Central
Google Scholar * Jones, R. B. et al. Tim-3 expression defines a novel population of dysfunctional T cells with highly elevated frequencies in progressive HIV-1 infection. _J. Exp. Med._
205, 2763–2779 (2008). CAS PubMed PubMed Central Google Scholar * Gao, X. et al. TIM-3 expression characterizes regulatory T cells in tumor tissues and is associated with lung cancer
progression. _PLoS One_ 7, e30676 (2012). CAS PubMed PubMed Central Google Scholar * Sakuishi, K. et al. Targeting Tim-3 and PD-1 pathways to reverse T cell exhaustion and restore
anti-tumor immunity. _J. Exp. Med._ 207, 2187–94. (2010). CAS PubMed PubMed Central Google Scholar * Japp, A. S. et al. Dysfunction of PSA-specific CD8+ T cells in prostate cancer
patients correlates with CD38 and Tim-3 expression. _Cancer Immunol. Immunother._ 64, 1487–1494 (2015). CAS PubMed Google Scholar * Yu, X. et al. The surface protein TIGIT suppresses T
cell activation by promoting the generation of mature immunoregulatory dendritic cells. _Nat. Immunol._ 10, 48–57 (2009). CAS PubMed Google Scholar * Sedy, J. R. et al. B and T lymphocyte
attenuator regulates T cell activation through interaction with herpesvirus entry mediator. _Nat. Immunol._ 6, 90–98 (2005). CAS PubMed Google Scholar * Li, X. et al. A comprehensive
analysis of key immune checkpoint receptors on tumor-infiltrating T cells from multiple types of cancer. _Front. Oncol._ 9, 1066 (2019). PubMed PubMed Central Google Scholar * Chen, L.
& Flies, D. B. Molecular mechanisms of T cell co-stimulation and co-inhibition. _Nat. Rev. Immunol._ 13, 227–242 (2013). PubMed PubMed Central Google Scholar * Lindstein, T. et al.
Regulation of lymphokine messenger RNA stability by a surface-mediated T cell activation pathway. _Science_ 244, 339–343 (1989). CAS PubMed Google Scholar * Linsley, P. S. et al. Binding
of the B cell activation antigen B7 to CD28 costimulates T cell proliferation and interleukin 2 mRNA accumulation. _J. Exp. Med._ 173, 721–730 (1991). CAS PubMed Google Scholar * Boise,
L. H. et al. CD28 costimulation can promote T cell survival by enhancing the expression of Bcl-XL. _Immunity_ 3, 87–98 (1995). CAS PubMed Google Scholar * Cook, C. H. et al.
CD28/B7-mediated co-stimulation is critical for early control of murine cytomegalovirus infection. _Viral Immunol._ 22, 91–103 (2009). CAS PubMed PubMed Central Google Scholar *
Douin-Echinard, V. et al. The expression of CD70 and CD80 by gene-modified tumor cells induces an antitumor response depending on the MHC status. _Cancer Gene Ther._ 7, 1543–1556 (2000). CAS
PubMed Google Scholar * Shuford, W. W. et al. 4-1BB costimulatory signals preferentially induce CD8+ T cell proliferation and lead to the amplification in vivo of cytotoxic T cell
responses. _J. Exp. Med._ 186, 47–55 (1997). CAS PubMed PubMed Central Google Scholar * Vidric, M. et al. Cooperation between 4-1BB and ICOS in the immune response to influenza virus
revealed by studies of CD28/ICOS-deficient mice. _J. Immunol._ 175, 7288–7296 (2005). CAS PubMed Google Scholar * Chen, S. et al. Combination of 4-1BB agonist and PD-1 antagonist promotes
antitumor effector/memory CD8 T cells in a poorly immunogenic tumor model. _Cancer Immunol. Res._ 3, 149–160 (2015). CAS PubMed Google Scholar * Segal, N. H. et al. Phase I study of
single-agent utomilumab (PF-05082566), a 4-1BB/CD137 agonist, in patients with advanced cancer. _Clin. Cancer Res._ 24, 1816–1823 (2018). CAS PubMed Google Scholar * Ascierto, P. A. et
al. Clinical experiences with anti-CD137 and anti-PD1 therapeutic antibodies. _Semin. Oncol._ 37, 508–516 (2010). CAS PubMed Google Scholar * Dong, C. et al. ICOS co-stimulatory receptor
is essential for T-cell activation and function. _Nature_ 409, 97–101 (2001). CAS PubMed Google Scholar * Fos, C. et al. ICOS ligation recruits the p50alpha PI3K regulatory subunit to the
immunological synapse. _J. Immunol._ 181, 1969–1977 (2008). CAS PubMed Google Scholar * Chen, H. et al. Anti-CTLA-4 therapy results in higher CD4+ICOShi T cell frequency and IFN-gamma
levels in both nonmalignant and malignant prostate tissues. _Proc. Natl Acad. Sci. USA_ 106, 2729–2734 (2009). CAS PubMed PubMed Central Google Scholar * Fu, T., He, Q. & Sharma, P.
The ICOS/ICOSL pathway is required for optimal antitumor responses mediated by anti-CTLA-4 therapy. _Cancer Res._ 71, 5445–5454 (2011). CAS PubMed Google Scholar * Pauls, K. et al. Role
of integrin alphaE(CD103)beta7 for tissue-specific epidermal localization of CD8+ T lymphocytes. _J. Invest. Dermatol._ 117, 569–575 (2001). CAS PubMed Google Scholar * Kutlesa, S. et al.
E-cadherin-mediated interactions of thymic epithelial cells with CD103+ thymocytes lead to enhanced thymocyte cell proliferation. _J. Cell Sci._ 115(Pt 23), 4505–4515 (2002). CAS PubMed
Google Scholar * Mackay, L. K. et al. The developmental pathway for CD103(+)CD8+ tissue-resident memory T cells of skin. _Nat. Immunol._ 14, 1294–1301 (2013). CAS PubMed Google Scholar *
Sathaliyawala, T. et al. Distribution and compartmentalization of human circulating and tissue-resident memory T cell subsets. _Immunity_ 38, 187–197 (2013). CAS PubMed Google Scholar *
Skon, C. N. et al. Transcriptional downregulation of S1pr1 is required for the establishment of resident memory CD8+ T cells. _Nat. Immunol._ 14, 1285–1293 (2013). CAS PubMed PubMed
Central Google Scholar * Kumar, B. V. et al. Human tissue-resident memory T cells are defined by core transcriptional and functional signatures in lymphoid and mucosal sites. _Cell Rep._
20, 2921–2934 (2017). CAS PubMed PubMed Central Google Scholar * Djenidi, F. et al. CD8+CD103+ tumor-infiltrating lymphocytes are tumor-specific tissue-resident memory T cells and a
prognostic factor for survival in lung cancer patients. _J. Immunol._ 194, 3475–3486 (2015). CAS PubMed Google Scholar * Hombrink, P. et al. Programs for the persistence, vigilance and
control of human CD8(+) lung-resident memory T cells. _Nat. Immunol._ 17, 1467–1478 (2016). CAS PubMed Google Scholar * Ganesan, A. P. et al. Tissue-resident memory features are linked to
the magnitude of cytotoxic T cell responses in human lung cancer. _Nat. Immunol._ 18, 940–950 (2017). CAS PubMed PubMed Central Google Scholar * Ling, K. L. et al. Modulation of CD103
expression on human colon carcinoma-specific CTL. _J. Immunol._ 178, 2908–2915 (2007). CAS PubMed Google Scholar * Pallett, L. J. et al. IL-2(high) tissue-resident T cells in the human
liver: sentinels for hepatotropic infection. _J. Exp. Med._ 214, 1567–1580 (2017). CAS PubMed PubMed Central Google Scholar * Yu, C. I. et al. Human CD1c+ dendritic cells drive the
differentiation of CD103+ CD8+ mucosal effector T cells via the cytokine TGF-beta. _Immunity_ 38, 818–830 (2013). CAS PubMed PubMed Central Google Scholar * Graham, J. B., Da Costa, A.
& Lund, J. M. Regulatory T cells shape the resident memory T cell response to virus infection in the tissues. _J. Immunol._ 192, 683–690 (2014). CAS PubMed Google Scholar * Enamorado,
M. et al. Enhanced anti-tumour immunity requires the interplay between resident and circulating memory CD8(+) T cells. _Nat. Commun._ 8, 16073 (2017). CAS PubMed PubMed Central Google
Scholar * Abd Hamid, M. et al. Self-maintaining CD103(+) cancer-specific T cells are highly energetic with rapid cytotoxic and effector responses. _Cancer Immunol. Res._ 8, 203–216 (2020).
PubMed Google Scholar * Piet, B. et al. CD8(+) T cells with an intraepithelial phenotype upregulate cytotoxic function upon influenza infection in human lung. _J. Clin. Invest._ 121,
2254–2263 (2011). CAS PubMed PubMed Central Google Scholar * Sandoval, F. et al. Mucosal imprinting of vaccine-induced CD8(+) T cells is crucial to inhibit the growth of mucosal tumors.
_Sci. Transl. Med._ 5, 172ra20 (2013). PubMed PubMed Central Google Scholar * Webb, J. R. et al. Tumor-infiltrating lymphocytes expressing the tissue resident memory marker CD103 are
associated with increased survival in high-grade serous ovarian cancer. _Clin. Cancer Res._ 20, 434–444 (2014). CAS PubMed Google Scholar * Le Floc’h, A. et al. Alpha E beta 7 integrin
interaction with E-cadherin promotes antitumor CTL activity by triggering lytic granule polarization and exocytosis. _J. Exp. Med._ 204, 559–570 (2007). PubMed PubMed Central Google
Scholar * Le Floc’h, A. et al. Minimal engagement of CD103 on cytotoxic T lymphocytes with an E-cadherin-Fc molecule triggers lytic granule polarization via a phospholipase Cgamma-dependent
pathway. _Cancer Res._ 71, 328–338 (2011). PubMed Google Scholar * Quinn, E. et al. CD103+ intraepithelial lymphocytes-a unique population in microsatellite unstable sporadic colorectal
cancer. _Eur. J. Cancer_ 39, 469–475 (2003). CAS PubMed Google Scholar * Malik, B. T. et al. Resident memory T cells in the skin mediate durable immunity to melanoma. _Sci. Immunol._ 2,
eaam6346 (2017). PubMed PubMed Central Google Scholar * Shields, B. D. et al. Loss of E-cadherin inhibits CD103 antitumor activity and reduces checkpoint blockade responsiveness in
melanoma. _Cancer Res._ 79, 1113–1123 (2019). CAS PubMed PubMed Central Google Scholar * Chang, C. H. et al. Posttranscriptional control of T cell effector function by aerobic
glycolysis. _Cell_ 153, 1239–1251 (2013). CAS PubMed PubMed Central Google Scholar * Menk, A. V. et al. Early TCR signaling induces rapid aerobic glycolysis enabling distinct acute T
cell effector functions. _Cell Rep._ 22, 1509–1521 (2018). CAS PubMed PubMed Central Google Scholar * Chang, C. H. et al. Metabolic competition in the tumor microenvironment is a driver
of cancer progression. _Cell_ 162, 1229–1241 (2015). CAS PubMed PubMed Central Google Scholar * Pan, Y. et al. Survival of tissue-resident memory T cells requires exogenous lipid uptake
and metabolism. _Nature_ 543, 252–256 (2017). CAS PubMed PubMed Central Google Scholar * Braud, V. M. et al. HLA-E binds to natural killer cell receptors CD94/NKG2A, B and C. _Nature_
391, 795–799 (1998). CAS PubMed Google Scholar * Lee, N. et al. HLA-E is a major ligand for the natural killer inhibitory receptor CD94/NKG2A. _Proc. Natl Acad. Sci. USA_ 95, 5199–5204
(1998). CAS PubMed PubMed Central Google Scholar * Tomasec, P. et al. Surface expression of HLA-E, an inhibitor of natural killer cells, enhanced by human cytomegalovirus gpUL40.
_Science_ 287, 1031 (2000). CAS PubMed Google Scholar * Ulbrecht, M. et al. Cutting edge: the human cytomegalovirus UL40 gene product contains a ligand for HLA-E and prevents NK
cell-mediated lysis. _J. Immunol._ 164, 5019–5022 (2000). CAS PubMed Google Scholar * Braud, V. M. et al. Expression of CD94-NKG2A inhibitory receptor is restricted to a subset of CD8+ T
cells. _Trends Immunol._ 24, 162–164 (2003). CAS PubMed Google Scholar * Andre, P. et al. Anti-NKG2A mAb is a checkpoint inhibitor that promotes anti-tumor immunity by unleashing both T
and NK cells. _Cell_ 175, 1731–1743.e13 (2018). CAS PubMed PubMed Central Google Scholar * van Montfoort, N. et al. NKG2A blockade potentiates CD8 T cell immunity induced by cancer
vaccines. _Cell_ 175, 1744–1755.e15 (2018). PubMed PubMed Central Google Scholar * Levy, E. M. et al. Human leukocyte antigen-E protein is overexpressed in primary human colorectal
cancer. _Int. J. Oncol._ 32, 633–641 (2008). CAS PubMed Google Scholar * de Kruijf, E. M. et al. HLA-E and HLA-G expression in classical HLA class I-negative tumors is of prognostic value
for clinical outcome of early breast cancer patients. _J. Immunol._ 185, 7452–7459 (2010). PubMed Google Scholar * Gooden, M. et al. HLA-E expression by gynecological cancers restrains
tumor-infiltrating CD8(+) T lymphocytes. _Proc. Natl Acad. Sci. USA_ 108, 10656–10661 (2011). CAS PubMed PubMed Central Google Scholar * Bossard, C. et al. HLA-E/beta2 microglobulin
overexpression in colorectal cancer is associated with recruitment of inhibitory immune cells and tumor progression. _Int J. Cancer_ 131, 855–863 (2012). CAS PubMed Google Scholar *
Farrar, M. A., Fernandez-Luna, J. & Schreiber, R. D. Identification of two regions within the cytoplasmic domain of the human interferon-gamma receptor required for function. _J. Biol.
Chem._ 266, 19626–19635 (1991). CAS PubMed Google Scholar * Dick, T. P. et al. Coordinated dual cleavages induced by the proteasome regulator PA28 lead to dominant MHC ligands. _Cell_ 86,
253–262 (1996). CAS PubMed Google Scholar * Groettrup, M. et al. Interferon-gamma inducible exchanges of 20S proteasome active site subunits: why?. _Biochimie_ 83, 367–372 (2001). CAS
PubMed Google Scholar * Seliger, B. et al. Downregulation of the constitutive tapasin expression in human tumor cells of distinct origin and its transcriptional upregulation by cytokines.
_Tissue Antigens_ 57, 39–45 (2001). CAS PubMed Google Scholar * Grell, M. et al. Induction of cell death by tumour necrosis factor (TNF) receptor 2, CD40 and CD30: a role for TNF-R1
activation by endogenous membrane-anchored TNF. _EMBO J._ 18, 3034–3043 (1999). CAS PubMed PubMed Central Google Scholar * Wajant, H., Pfizenmaier, K. & Scheurich, P. Tumor necrosis
factor signaling. _Cell Death Differ._ 10, 45–65 (2003). CAS PubMed Google Scholar * Nakajima, C. et al. A role of interferon-gamma (IFN-gamma) in tumor immunity: T cells with the
capacity to reject tumor cells are generated but fail to migrate to tumor sites in IFN-gamma-deficient mice. _Cancer Res._ 61, 3399–3405 (2001). CAS PubMed Google Scholar * Shen, C. et
al. Frequency and reactivity of antigen-specific T cells were concurrently measured through the combination of artificial antigen-presenting cell, MACS and ELISPOT. _Sci. Rep._ 7, 16400
(2017). PubMed PubMed Central Google Scholar * Abd Hamid, M. et al. Defective interferon gamma production by tumor-specific CD8(+) T cells is associated with 5’methylcytosine-guanine
hypermethylation of interferon gamma promoter. _Front. Immunol._ 11, 310 (2020). PubMed PubMed Central Google Scholar * Samten, B. et al. CREB, ATF, and AP-1 transcription factors
regulate IFN-gamma secretion by human T cells in response to mycobacterial antigen. _J. Immunol._ 181, 2056–2064 (2008). CAS PubMed Google Scholar * Moore, L. D., Le, T. & Fan, G. DNA
methylation and its basic function. _Neuropsychopharmacology_ 38, 23–38 (2013). CAS PubMed Google Scholar * Feske, M. et al. Enhancement of human antigen-specific memory T-cell responses
by interleukin-7 may improve accuracy in diagnosing tuberculosis. _Clin. Vaccin. Immunol._ 15, 1616–1622 (2008). CAS Google Scholar * Sargentini, V. et al. Cytometric detection of
antigen-specific IFN-gamma/IL-2 secreting cells in the diagnosis of tuberculosis. _BMC Infect. Dis._ 9, 99 (2009). PubMed PubMed Central Google Scholar Download references
ACKNOWLEDGEMENTS This work was supported by Chinese Academy of Medical Sciences (CAMS) Innovation Fund for Medical Sciences (CIFMS), China (grant number: 2018-I2M-2-002) and MRC Human
Immunology Unit Core and Nuffield Department of Medicine, Oxford University. Illustrations were created with BioRender (Biorender.com). We thank Rhys Woods for proofreading and editing, and
Zixi Yin for assisting with designing the illustrations. AUTHOR INFORMATION AUTHORS AND AFFILIATIONS * Nufield Department of Medicine, Chinese Academy of Medical Science Oxford Institute
(COI), University of Oxford, Oxford, UK Megat Abd Hamid, Yanchun Peng & Tao Dong * MRC Human Immunology Unit, MRC Weatherall Institute of Molecular Medicine, University of Oxford,
Oxford, UK Yanchun Peng & Tao Dong Authors * Megat Abd Hamid View author publications You can also search for this author inPubMed Google Scholar * Yanchun Peng View author publications
You can also search for this author inPubMed Google Scholar * Tao Dong View author publications You can also search for this author inPubMed Google Scholar CORRESPONDING AUTHOR
Correspondence to Tao Dong. ETHICS DECLARATIONS COMPETING INTERESTS The authors declare no competing interests. RIGHTS AND PERMISSIONS OPEN ACCESS This article is licensed under a Creative
Commons Attribution 4.0 International License, which permits use, sharing, adaptation, distribution and reproduction in any medium or format, as long as you give appropriate credit to the
original author(s) and the source, provide a link to the Creative Commons license, and indicate if changes were made. The images or other third party material in this article are included in
the article’s Creative Commons license, unless indicated otherwise in a credit line to the material. If material is not included in the article’s Creative Commons license and your intended
use is not permitted by statutory regulation or exceeds the permitted use, you will need to obtain permission directly from the copyright holder. To view a copy of this license, visit
http://creativecommons.org/licenses/by/4.0/. Reprints and permissions ABOUT THIS ARTICLE CITE THIS ARTICLE Abd Hamid, M., Peng, Y. & Dong, T. Human cancer germline antigen-specific
cytotoxic T cell—what can we learn from patient. _Cell Mol Immunol_ 17, 684–692 (2020). https://doi.org/10.1038/s41423-020-0468-x Download citation * Received: 08 April 2020 * Revised: 10
May 2020 * Accepted: 12 May 2020 * Published: 25 May 2020 * Issue Date: July 2020 * DOI: https://doi.org/10.1038/s41423-020-0468-x SHARE THIS ARTICLE Anyone you share the following link with
will be able to read this content: Get shareable link Sorry, a shareable link is not currently available for this article. Copy to clipboard Provided by the Springer Nature SharedIt
content-sharing initiative KEYWORDS * cytotoxic T cell * immune check point receptor * immune therapy * T cell receptor * cancer antigen