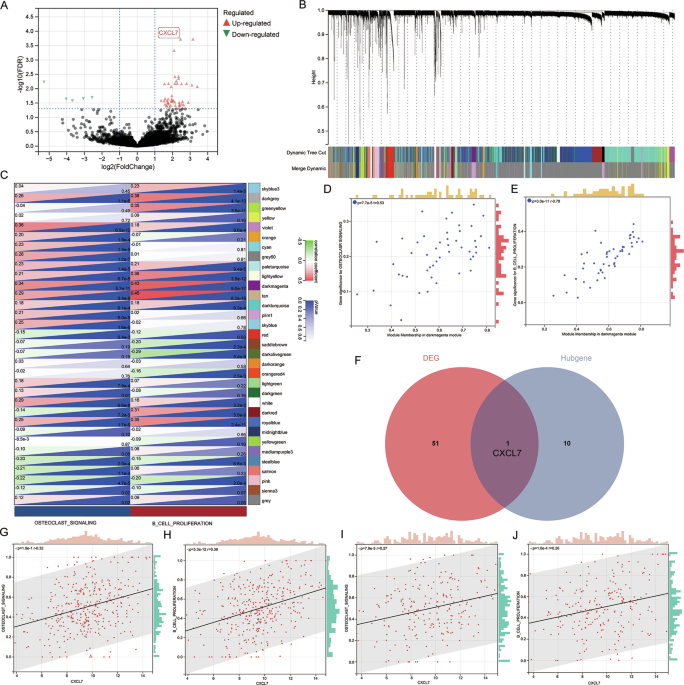
- Select a language for the TTS:
- UK English Female
- UK English Male
- US English Female
- US English Male
- Australian Female
- Australian Male
- Language selected: (auto detect) - EN
Play all audios:
ABSTRACT Osteolytic lesions and pathological fractures are hallmark manifestations of multiple myeloma (MM), profoundly influencing the quality of life and self-care ability of MM patients.
By analyzing transcriptome data and single-cell RNA-seq data from our center and the GEO database, a subset of MM cells with high expression levels of chemokine CXCL7 was identified. This
subset of MM cells possesses a high capacity for proliferation and activation of osteoclast signaling pathway. CXCL7 might be a crucial regulator of osteolytic damage in MM. Subsequently,
the association between the expression level of CXCL7 and pathological fractures in patients was investigated, and the impact of CXCL7 on MM proliferation was confirmed both in vivo and in
vitro. A mouse xenograft tumor model was established through intravenous injection of myeloma cell lines based on the homing ability of plasma cells. Moreover, the mechanism by which CXCL7
promotes the activation of osteoclast signaling pathways in MM was explored. Our findings reveal that elevated CXCL7 levels significantly enhance MM cell proliferation, increasing the risk
of pathological fractures in MM patients. Additionally, our mouse xenograft tumor model demonstrated that CXCL7 can induce femoral fractures and reduce bone mineral density. Concurrently, it
was discovered that CXCL7 could activate the JAK/STAT3 pathway via CXCR2 and upregulate the expression levels of MMP13 and C-myc, facilitating MM cell proliferation and activation of the
osteoclast signaling pathway. Our study offers novel insights into the pathogenic mechanism of osteolytic lesions and implies that targeting CXCL7 may present a new therapeutic avenue for
MM. SIMILAR CONTENT BEING VIEWED BY OTHERS LY6E AS A NEW PROGNOSTIC BIOMARKER OF MULTIPLE MYELOMA-RELATED BONE DISEASE Article Open access 03 April 2025 EZH2 SERVES AS A VIABLE THERAPEUTIC
TARGET FOR MYELOMA-INDUCED OSTEOLYTIC BONE DESTRUCTION Article Open access 31 January 2025 MIR-21 ANTAGONISM ABROGATES TH17 TUMOR PROMOTING FUNCTIONS IN MULTIPLE MYELOMA Article 06 July 2020
INTRODUCTION Multiple myeloma (MM) is a difficult-to-cure malignant tumor with a relatively slow progression and high intra-tumor heterogeneity [1]. MM presents with diverse clinical
manifestations, target organ damage, and prognosis among different patients [2]. The emergence of osteolytic lesions and the consequent occurrence of pathological fractures represent one of
the crucial factors affecting the quality of life and prognosis of patients with myeloma. Pathological fractures also serve as a significant cause for patients with myeloma to lose their
self-care capacity in the terminal stage [3, 4]. Osteolytic injury in myeloma is driven by two key factors. First, the proliferation of myeloma cells suppresses normal bone marrow function.
Second, the interaction between myeloma cells and the bone marrow microenvironment activates osteoclast-related pathways, leading to osteolytic damage [5]. Patients with MM often experience
relatively long overall survival. In fact, as opposed to the complete eradication of monoclonal plasma cells, modulating the biological behavior of myeloma cells to extend the survival
period with tumor and concurrently control the degree of target organ damage could be a more efficacious therapeutic modality. Identifying key genes that influence myeloma cell behavior,
particularly those involved in regulating osteoclast signaling, is therefore of significant clinical importance. Through a comprehensive analysis of RNA-seq and single-cell sequencing data
of myeloma, we identified that in myeloma, CXCL7 has the capacity to facilitate osteolytic destruction and the proliferation of myeloma. Chemokine CXCL7 pertains to a category of chemotactic
cytokines and assumes a crucial role in regulating the migration and functionality of immune cells [6]. In tumors, chemokines not only direct the migration of immune cells to influence the
anti-tumor immune response [7] but also regulate the proliferation, invasion, metastasis, and angiogenesis of tumor cells by activating diverse signaling pathways such as STAT3 and NF-κB [8,
9]. However, the exact mechanisms by which CXCLs influence tumors remain unclear. Plasma cells, derived from B cells, are influenced by chemokines that regulate their infiltration,
proliferation, and interactions with stromal cells in tissues, affecting their overall biological functions. That is to say, chemokines might play a decisive role in immune cell tumors.
Previous studies have demonstrated that CXCL7 exerts a role in promoting proliferation and invasion in malignant tumors such as breast cancer, lung cancer, and renal cancer, and is
associated with an adverse prognosis [10,11,12]. However, the role of CXCL7 in MM remains indistinct. This study aims to explore the role and related mechanisms of CXCL7 in MM to furnish a
theoretical basis for identifying novel therapeutic targets. RESULTS CXCL7 IS ONE OF THE KEY GENES ASSOCIATED WITH PATHOLOGICAL FRACTURES IN MYELOMA Our research aimed to identify key genes
affecting MM cell proliferation and osteoclast signaling. We collected bone marrow specimens from 30 newly diagnosed MM (NDMM) patients: 15 with more than five osteolytic lesions and
pathological fractures, and 15 without osteolytic damage. After CD138 magnetic bead sorting, we performed high-throughput transcriptome sequencing, identifying 52 differentially expressed
genes (|logFC | > 1, FDR < 0.01) (via DESeq2) (Fig. 1A). Next, we analyzed the GSE24080 dataset, which includes two cohorts of NDMM patients: the Total Therapy 2 [13] (TT2) cohort (340
cases) and the Total Therapy 3 [14] (TT3) cohort (214 cases). TT2 cohort was designated as a training set, while TT3 cohort was utilized as a validation set. WGCNA analysis categorized the
23,517 genes in the TT2 set into 33 modules (Fig. 1B). Subsequently, ssGSEA was adopted to quantify the activation of “B cell proliferation” and “osteoclast signaling pathway” for all
patients. Correlations between the co-expressed gene modules from WGCNA and pathway activation were examined. The results demonstrated that the dark magenta module significantly facilitated
the activation of the “B cell proliferation” (_P_ = 5.6e−11) and “osteoclast signaling” (_P_ = 9.0e−17) pathways (Fig. 1C). Then, the correlations of all genes within the dark magenta module
with these two traits were computed (Fig. 1D–E). Based on the truncation criterion (|Module membership | > 0.70), 11 genes were identified as hub genes. By intersecting the
differentially expressed genes and the hub genes, we found that CXCL7 was a potential key regulator (Fig. 1F). Further analysis in the TT2 cohort confirmed that CXCL7 expression was
positively correlated with both “B cell proliferation” (_P_ < 0.0001) (Fig. 1G) and “osteoclast signaling” (_P_ < 0.0001) (Fig. 1H). This correlation was also validated in the TT3
cohort (Fig. 1I–J). MM CELLS FEATURING A HIGH EXPRESSION LEVEL OF CXCL7 POSSESS ENHANCED OSTEOCLAST SIGNALING PATHWAY ACTIVITY Given the high intratumor heterogeneity of MM, we aim to
further explore the characteristics of MM cell subsets with elevated CXCL7 expression. Bone marrow samples were collected from three NDMM patients with pathological fractures for single-cell
RNAseq. The data from individual cells across all patients were integrated and analyzed using UMAP downscaling (Fig. 2A–C). In addition to high CXCL7 expression in monocytes, we observed a
significant upregulation of CXCL7 in some plasma cells as well (Fig. 2D). Further analysis revealed 16 distinct clonal subpopulations of MM cells (Fig. 2E). CXCL7 expression was most
prominent in the mm03 subgroup (Fig. 2F). Although CXCL7 is expressed only in a subset of MM cells, subgroup cells with an elevated CXCL7 expression can be identified in all three patients
(Fig. 2G). We selected “B cell proliferation” and “osteoclast signaling” as target pathways and conducted signaling pathway enrichment analysis. The results showed that the mm03 subgroup had
the highest median activation of the “osteoclast signaling” pathway, with “B cell proliferation” ranking second (Fig. 2H–I). Moreover, the mm03 subgroup demonstrated the most significant
correlation with both pathways (Fig. 2J). CXCL7 IS ASSOCIATED WITH PATHOLOGICAL FRACTURES IN PATIENTS We collected bone marrow samples from 108 NDMM patients from 2016 to 2020 and assessed
CXCL7 expression using qPCR. All patients received an initial combination treatment regimen of proteasome inhibitors, immunomodulators, and dexamethasone. We determined the optimal cut-off
value for CXCL7 and compared the prognosis and clinicopathological disparities among patients with different expression levels of CXCL7 (Fig. 3A–B). The findings revealed that patients in
the high CXCL7 expression group had a higher likelihood of pathological fracture (P = 0.048) (Fig. 3C) and extramedullary invasion (_P_ = 0.010) (Fig. 3D). Moreover, elevated levels of CXCL7
were associated with reduced overall survival (OS) (P = 0.016) and progression-free survival (PFS) (P = 0.008) (Fig. 3E–F). However, no significant correlation was identified between CXCL7
and ISS stage or high-risk cytogenetics. The clinical baseline characteristics of the patients are presented in Table 1. We then investigated the association between CXCL7 expression levels
and the number of osteolytic lesions in patients. Osteolytic lesions were evaluated via PET-CT. Spearman correlation analysis revealed a significant positive correlation between CXCL7
expression and the number of osteolytic lesions (_P_ = 0.034) (Fig. 3G). To further validate the clinical relevance of CXCL7, we assessed its prognostic value in the Multiple Myeloma
Research Foundation (MMRF) database, the largest publicly available dataset of MM patients. A total of 784 NDMM patients with complete prognostic data and RNA-seq data were included in the
study. Survival analysis, based on the optimal cutoff value, revealed that higher CXCL7 expression was significantly associated with shorter OS time (P = 0.006) (Fig. 3H). CXCL7 PROMOTES
PROLIFERATION OF MM CELLS In CCLE database, CXCL7 expression was found to be low in the NCI-H929 cell line, moderate in the RPMI 8226 cell line, and high in the U266 cell line (Fig. 4A).
Lentiviruses carrying CXCL7-overexpression (CXCL7-OE) and Normal-Control (NC) were used to infect NCI-H929 and RPMI 8226 cell lines. Meanwhile, siRNA was employed for CXCL7 knockdown
(CXCL7-KD) in U266 and RPMI 8226 cell lines. CXCL7 RNA levels were measured by qPCR (Fig. 4B–E). Protein expression, both intracellular and secreted, was assessed using Western blotting
(Fig. 4F–I) and ELISA (Fig. 4J–M). The CCK-8 assay was used to evaluate cell proliferation. The results showed a significantly higher proliferation rate in the CXCL7-OE group for both H929
and RPMI 8226 cell lines (Fig. 4N–O). In contrast, the CXCL7-KD group exhibited slower proliferation in U266 and RPMI 8226 cells (Fig. 4P–Q). Subcutaneous xenograft tumor models were
established in B-NGD mice with CXCL7-OE and NC RPMI 8226 cell lines. Analysis revealed accelerated tumor growth in the CXCL7-OE group (Fig. 4R–T). Immunohistochemistry performed on excised
tumors demonstrated elevated levels of Ki-67 proliferation index in the CXCL7-OE group (Fig. 4U). CXCL7 FACILITATE INCIDENCE OF PATHOLOGICAL FRACTURES IN MOUSE Subsequently, a murine
xenograft model was established via intravenous infusion of MM cells to scrutinize the colonization of CXCL7-OE MM cells in the bone marrow by virtue of the homing effect and its
ramification on the occurrence of osteolytic lesions (Fig. 5A). This model mimics the in vivo behavior of plasma cells, allowing us to investigate the influence of CXCL7 on myeloma cell
activity in a more physiologically relevant context. At 7 weeks, three out of five mice in the CXCL7-OE group exhibited symptoms of long bone fractures. We performed regular venous blood
collection to measure serum free light chain levels and assess tumor burden, which showed a significantly higher tumor burden in the CXCL7-OE group (Fig. 5B). Micro-CT imaging of femoral
bones revealed more pronounced bone deterioration in the CXCL7-OE group (Fig. 5C). Specifically, bone mineral density (BMD) was significantly reduced in the CXCL7-OE group (_P_ = 0.007),
with a mean decrease of 105 mg/cc compared to the NC group. Additionally, the average trabecular thickness (Tb, Th) was significantly decreased in the CXCL7-OE group (P = 0.037), indicating
that CXCL7 overexpression accelerates bone loss and disrupts trabecular structure. Then, tissue samples from the femur, subcutaneous metastases, livers, lungs, and kidneys were collected for
histopathological examination via hematoxylin and eosin (HE) staining (Fig. 5D–E). Subsequently, we performed immunohistochemical analysis to detect BCMA and CD138, which allowed us to
assess the presence of monoclonal plasma cells in the bone marrow of mice. Distinct BCMA (+) and CD138 (+) plasma cell infiltrates were observed in the femurs of both the OE and NC groups
(Fig. 5F). The femur sections revealed that myeloma cells in the CXCL7-OE group showed enhanced colonization and homing abilities within the bone marrow. CXCL7 ACTIVATES THE JAK/STAT3
SIGNALING PATHWAY We conducted RNA-seq on CXCL7-OE and NC RPMI 8226 cell lines and identified differentially expressed genes (Fig. 6A). KEGG pathway analysis revealed significant enrichment
of the JAK/STAT signaling pathway in the CXCL7-OE cells (Fig. 6B–C). Among the differentially expressed genes, JAK1 and STAT3 were conspicuously upregulated. Prior studies have validated
that CXCR2 acts as the primary receptor of CXCL7, and the JAK/STAT3 is one of the pathways activated following the activation of CXCR2 [15, 16]. We postulate that CXCL7 activates the
JAK/STAT3 pathway via CXCR2, thereby influencing the proliferation of MM cells and further triggering the expression of downstream activating proteins associated with the osteoclast
signaling pathway (Fig. 6D). To explore the JAK/STAT3 signaling pathway, we carried out WB to evaluate the expression of JAK1, phosphorylated JAK1 protein (p-JAK1), STAT3, phosphorylated
STAT3 (p-STAT3) and CXCR2 in different MM cell lines. In both H929 and RPMI 8226 cell lines, the levels of p-JAK1 and p-STAT3 were significantly increased in CXCL7-OE group (Fig. 6E).
Conversely, in U266 and RPMI 8226 cell lines, these levels were reduced in CXCL7-KD group (Fig. 6F). Additionally, the expression level of CXCR2 was significantly upregulated in CXCL7-OE
H929 and RPMI8226 cells (Fig. 6E) and significantly downregulated in CXCL7-KD U266 and RPMI8226 cells (Fig. 6F). Co-immunoprecipitation (Co-IP) confirmed that CXCL7 interacts with CXCR2 in
both H929 and RPMI8226 cells (Fig. 6G). These results suggest that increased CXCL7 expression enhances its binding to CXCR2 and activates the JAK/STAT3 pathway. Immunohistochemical analysis
of the mouse xenograft tumor model further confirmed significant JAK/STAT3 pathway activation in CXCL7-OE group (Fig. 6H). Additionally, single-cell RNA sequencing of MM patient samples
revealed a strong co-expression of CXCL7 and JAK/STAT3 in the mm03 subgroup (Fig. 2K). JAK/STAT3 forms a crucial oncogenic pathway in MM, traditionally believed to be activated by IL-6.
However, in the absence of IL6, most MM cell lines still have a highly active JAK/STAT3 pathway. Our research found that a subset of MM cells secreting CXCL7 can activate the JAK/STAT3
pathway through autocrine effects. Also, we observed enhanced activation of JAK/STAT3 in other cell subgroups (Fig. 2K), providing evidence for its potential activation via multiple
mechanisms. CXCL7 UPREGULATES THE EXPRESSION LEVELS OF MMP13 AND C-MYC VIA JAK/STAT3 PATHWAY We next investigated the downstream genes activated by the JAK/STAT3 pathway. Transcriptome
sequencing of CXCL7-OE cells revealed significant upregulation of matrix metalloproteinase 13 (MMP13) and C-myc. Both MMP13 and C-myc are crucial effector proteins in response to JAK/STAT3
activation and are closely associated with osteoclast signaling and MM cell proliferation. Consequently, we proceeded with the validation of MMP13 and C-myc expression levels. The results
showed a significant increase in both MMP13 and C-myc in CXCL7-OE cells (Fig. 6E), whereas their expression was significantly reduced in CXCL7-KD cells (Fig. 6F). Meanwhile, we utilized TRAP
as a marker for osteoclasts and observed a significant increase in osteoclast numbers in the bone tissue of CXCL7-OE mice. Furthermore, we assessed the expression levels of MMP2, MMP9, and
MMP13, which serve as markers for proteins associated with the activation of the osteoclast signaling pathway. Our findings revealed a significant elevation in the expression of all three
MMPs in the bone tissue of the CXCL7-OE mice, with MMP13 showing the highest expression (Fig. 7A–B). To further substantiate that the upregulation of JAK/STAT3 is attributable to CXCR2, we
treated MM cells with SB225002, a CXCR2 inhibitor. This treatment led to a significant reduction in JAK/STAT3 activation (Fig. 7C–D) and cell proliferation (Fig. 7E–F). We further treated
CXCL7-OE cells with Ruxolitinib (JAK inhibitor) (Fig. 7G–H). The results demonstrated that both p-STAT3 expression and cell proliferative capacity were significantly suppressed (Fig. 7G–J).
Furthermore, treatment of MM cells with CXCR2 inhibitors and JAK inhibitors resulted in downregulation of MMP13 and C-myc expression (Fig. 7C–D & G–H). We further explored the
relationship between CXCL7 and the expression of CXCR2 and MMP13 using data from the MMRF database. Our analysis revealed a significant positive correlation between CXCL7 and both CXCR2 (P
< 0.001) and MMP13 (P < 0.001) (Fig. S1). ELEVATED EXPRESSION OF CXCL7 IN MM CELLS SIGNIFICANTLY FACILITATES THE GENERATION OF OSTEOCLASTS To further validate that high CXCL7
expression in MM cells promotes osteoclastogenesis, we co-cultured RPMI8226 CXCL7-OE/NC cell lines with bone marrow-derived macrophages (BMDMs) isolated from C57BL/6 mice. M-CSF and RANKL
were added to the BMDMs to induce osteoclast differentiation. After 6 days of co-culture, TRAP staining was performed to assess osteoclast formation. The results showed that BMDMs
co-cultured with CXCL7-OE cells generated a significantly higher number of multinucleated osteoclasts compared to the NC group, indicating that elevated CXCL7 expression enhances
osteoclastogenesis (Fig. 7K). Subsequently, immunofluorescence was employed to examine both MMP13 and TRAP (a marker for osteoclasts) within femoral bone marrow from mouse xenograft models
(Fig. 7L). The results revealed a marked co-localization of MMP13 around TRAP-positive multinucleated osteoclasts, suggesting a strong interaction between MMP13 and osteoclasts. In contrast,
no such co-expression was observed in the NC group, further supporting the role of MMP13 secreted by myeloma cells in facilitating osteoclast activity. DISCUSSION MM is a heterogeneous
tumor characterized by diverse subpopulations of myeloma cells with distinct biological behaviors [5, 17, 18]. Osteolytic damage and pathological fractures are hallmark clinical symptoms of
myeloma, leading to restricted mobility and loss of self-care ability in MM patients [4]. Our research identifies CXCL7 as a key gene contributing to pathological fractures in MM.
Subpopulations of MM cells with elevated CXCL7 expression show enhanced activation of the osteoclast signaling pathway. Notably, CXCL7 is a member of the chemokine family, which plays a
critical role in regulating immune cell function [19, 20]. Given that MM originates from lymphocytes, chemokines like CXCL7 are likely key regulators of myeloma cell behavior. Current
research on the role of CXCL7 in tumors is limited. Previous studies have shown that in glioma, tumor-induced macrophages secrete CXCL7 to enhance glioma stem cell functionality [21]. In
breast cancer, targeting CXCL7 inhibits tumor growth and metastasis [10], while in cholangiocarcinoma, CXCL7 promotes tumor proliferation and invasion via the AKT signaling pathway [22]. In
MM, CXCL7 has been identified as the predominant activator of MMP13. CXCL7 can be secreted by MSCs, thereby stimulating MM cells to produce a significant amount of MMP13, which constitutes a
crucial means for activating the osteoclast signaling pathway [23, 24]. Our research has disclosed that in MM, not only stromal cells but also a subset of myeloma cells secrete CXCL7 in an
autocrine manner. CXCL7 activates the JAK/STAT3 pathway within MM cells via the CXCR2 receptor and upregulates the expression of MMP13. Through multiple analyses, we confirmed that MM cells
with elevated CXCL7 expression secrete high levels of MMP13. A strong correlation was observed between the gene expression of CXCL7, CXCR2, and MMP13 in the MMRF database. However, no
significant correlation was found between CXCL7 and MYC gene expression. We hypothesize that this lack of correlation arises because MMP13 in myeloma cells is primarily induced by CXCL7,
whereas MYC, involved in various cancer pathways, is regulated by multiple mechanisms. As only a subset of myeloma cells secretes CXCL7, the potential association between CXCL7 and MYC
expression may be diluted in the broader plasma cell RNA-seq data. The JAK/STAT3 pathway is widely recognized as a crucial oncogenic pathway in MM. It was formerly hypothesized that it was
primarily activated via IL6 [25]. Nevertheless, the activation of the JAK/STAT3 pathway is ubiquitous in MM [26]; numerous MM cells still exhibit a significantly activated JAK/STAT3 pathway
in the absence of IL6, suggesting the involvement of alternative activation mechanisms. Our Single-cell RNA sequencing analysis revealed widespread activation of the JAK/STAT3 pathway across
various MM subgroups, with a notable co-localization of JAK/STAT3 and CXCL7 in one subgroup. This concurrently implies that there are additional pathways for the activation of STAT3. STAT3
is a key transcription factor involved in the initiation and progression of MM [27]. In our study, we observed that the upregulation of STAT3 significantly increased the protein levels of
MMP13 and C-myc. MMPs play a critical role in osteolytic damage in MM and promote tumor growth by enhancing angiogenesis and facilitating the migration and invasion of MM cells [28,29,30].
Interestingly, previous studies have shown that IL6 can lead to the upregulation of MMP13, but CXCL7 is the most significant factor in upregulating MMP13 in MM [23, 24]. Our study explains
this phenomenon, that is, CXCL7 leads to the upregulation of MMP13 through the activation of the JAK/STAT3 pathway. Our research examined the role of CXCL7 in promoting osteolytic lesions in
myeloma using various methods. In animal studies, we measured femur BMD using Micro-CT. BMD was calculated as Bone Mineral Content (BMC) divided by Bone Volume (BV). As BV was computed
using a computerized method, fractured portions of the femur, which were disconnected from the rest of the bone, were excluded from the analysis. Given that the CXCL7-OE group exhibited more
pathological fractures, this exclusion likely minimized differences in BDM between OE and NC groups. In the NC group, implantation of myeloma cells into the femurs also resulted in a
decrease in BMD compared to normal mice. However, the OE group displayed a significantly greater reduction in BMD, with a decrease of over 100 mg/cc compared to the NC group, reflecting
substantial bone loss. Our findings highlight that a subset of myeloma cells secretes CXCL7 via an autocrine mechanism, playing a pivotal role in tumor progression and the development of
osteolytic lesions. Targeting CXCL7 emerges as a promising therapeutic strategy, as inhibiting this autocrine loop could help prevent bone deterioration. Despite CXCL7 being expressed in
only a fraction of myeloma cells, the high intra-tumor heterogeneity of myeloma suggests that targeting these particularly aggressive subpopulations could provide substantial clinical
benefit. Blocking CXCL7 may reduce MMP13 production, decrease osteoclast activation, and mitigate bone resorption, ultimately lowering fracture risk and improving patient outcomes. While
research on CXCL7 inhibitors for MM is still in its early stages and no targeted drugs have been developed, the potential to treat patients with severe bone lesions is considerable. The
development of CXCL7-targeted therapies could open new avenues for managing myeloma and its complications. A deeper understanding of CXCL7’s mechanisms, coupled with the development of
effective inhibitors, will be essential for advancing therapeutic options and improving patient care. METHODS AND MATERIALS WEIGHTED CORRELATION NETWORK ANALYSIS (WGCNA) First, we utilized
the gene expression profiles of patients in the GEO database. We chose the GSE24080 cohort and designated the TT2 patient group as the training set, while assigning the TT3 patient group as
the validation set. In the TT2 training set, we separately calculated the Median Absolute Deviation (MAD) for each gene. To exclude the genes with the smallest MAD, we excluded the top 50%
of genes. The R package “goodSamplesGenes” was utilized for the removal of outlier genes and samples, and WGCNA was further used to construct a scale-free co-expression network. Initially,
we performed both Pearson’s correlation matrices and the average linkage method for all pairwise genes. After selecting the power of 5, the adjacency was transformed into a topological
overlap matrix (TOM) to measure the network connectivity of a gene by defining it as the sum of its adjacency with all other genes for network gene ration, and the corresponding
dissimilarity (1-TOM) was calculated. To classify genes with similar expression profiles into gene modules, we conducted average linkage hierarchical clustering according to the TOM-based
dissimilarity measure with a minimum gene group size of 30 for the genes dendrogram. Additionally, we merged modules with a distance of less than 0.25. SINGLE SAMPLE GENE SET ENRICHMENT
ANALYSIS (GSEA) Single sample GSEA (ssGSEA) analysis was performed to determine the degree of activation for each pathway, which was recorded as the Enrichment Score (ES). The score ranged
from 0 to 1, indicating minimal to maximal activation. The activation scores for the “B cell tumor proliferation” pathway and the “osteoclast signaling activation” pathway were calculated
using ssGSEA. The gene sets for these pathways were obtained from the MsiGDB database. THE EXPRESSION LEVEL OF CXCL7 AND CLINICOPATHOLOGICAL FEATURES OF SAMPLES A total of 108 clinical
samples of newly diagnosed MM patients were included in this study, which was collected from patients who visited Zhongshan Hospital of Fudan University from 2016 to 2020. In order to
further observe the relationship between CXCL7 and the prognosis and drug treatment response of patients, only patients who had not undergone autologous hematopoietic stem cell
transplantation were included. All patients received an initial treatment of a three-drug combination of protease inhibitor, lenalidomide, and dexamethasone. The bone marrow samples of
patients were collected at the time of diagnosis. All bone marrow fluid samples were enriched with nucleated cells and sorted with CD138 magnetic beads. The expression level of CXCL7 is
detected by the qPCR. SINGLE CELL MRNA SEQUENCING A diverse array of bone marrow samples was collected from Zhongshan Hospital affiliated with Fudan University, following approval from the
Institutional Review Board of Zhongshan Hospital. Bone marrow mononuclear cells (BMMCs) were isolated and employed for single-cell RNA sequencing (scRNAseq). Single-cell libraries were
generated from unsorted BMMCs using a 10× Genomics Chromium Single Cell 5′ Library Kit and Chromium instrument, followed by sequencing on an Illumina sequencer. The obtained FASTQ data was
aligned to the reference genome (GRCh38) using Cell Ranger (v5.0.1), subsequently undergoing read filtering, barcode counting, and unique molecular identifier (UMI) counting. Seurat v4.0.1
software was utilized for cell clustering and dimension reduction. Single-cell data from different patients were integrated and analyzed via the UMAP downscaling approach. For pathway
activation analysis in different cell subsets, we employed the irGSEA package along with AUCell and UCell methods to score pathway activation levels based on gene sets obtained from the
MSigDB database. CELL CULTURE U266 and NCI-H929 cell lines are purchased from the Institute of Basic Medical Sciences, Chinese Academy of Medical Sciences, and RPMI-8226 cell line is
obtained from the cell bank of the Chinese Academy of Sciences for use in this investigation. Cells are grown in RPMI 1640 media (Gibco, USA) with 10% fetal bovine serum (FBS) (Gibco, USA)
under 37 °C, saturated humidity, and 5% CO2 conditions. ESTABLISHMENT OF LENTIVIRAL STABILIZED CELL LINES The CXCL7 overexpression and control lentiviruses were obtained from Genechem
Biotechnology Co., Ltd. (Shanghai, China). The vector components are arranged in the following sequence: Ubi-MCS-3FLAG-CBh-gcGFP-IRES-puromycin. H929 and RPMI8226 cells were subjected to
infection at a multiplicity of infection (MOI) of 100 for 12 h. Seventy-two hours post-transduction, the culture medium was amended with 2 μg/ml puromycin dihydrochloride (GeneChem, China)
to facilitate the selection of stably transfected cells. The successful overexpression of CXCL7 was validated using Western blot analysis and quantitative PCR. SMALL INTERFERING RNA
TRANSFECTION Small interfering RNA (siRNA) targeting CXCL7 (Genomeditech, China), with the sequence provided in Supplementary Table 1, was used for transfection. U266 and RPMI8226 cells in
the logarithmic growth phase were seeded into a six-well cell culture plate. The transfection complex was prepared by mixing 125 μL of RPMI 1640 medium (Gibco, USA), 3.75 μL of Lipo3000
reagent (Thermo Fisher Scientific, USA), and 10 μL of siRNA. After allowing the transfection complex to stand for 20 min, it was added to the six-well plate and gently mixed with the cell
suspension. The plate was then incubated at the appropriate conditions for 24-48 h before changing to a fresh medium. ENZYME-LINKED IMMUNOSORBENT ASSAY (ELISA ASSAY) The concentration of
human CXCL7 cytokine in the culture medium was measured using an ELISA kit (Abcam, England). All procedures were performed according to the manufacturer’s instructions. QUANTITATIVE RT-PCR
ANALYSIS Quantitative RT-PCR analyses were performed using the EZ Bioscience RNA Extraction Kit to extract RNA from control or treated cells, followed by cDNA synthesis with the Takara cDNA
Synthesis Kit and real-time PCR using the Takara TB Green Premix Ex Taq (Tli RNaseH Plus) quantitative PCR kit. All primer sequences used in this study are shown in Supplementary Table 2.
WESTERN BLOT ANALYSIS To lyse the cells, use 1X RIPA buffer containing Tris buffer (50 mM, pH 7.4), NaCl (150 mM), Triton X-100 (1%), PMSF (1 mM), SDS (0.1%), and protease inhibitor mixture
from Beyotime Biotechnology. Cell lysates were mixed with Loading Buffer, separated by electrophoresis in a 10-15% SDS-PAGE gel, and transferred to a PVDF membrane from Millipore. Membranes
were blocked with QuickBlock Blocking Buffer for Western Blot from Biotechnology. Membranes were washed three times with PBST and incubated with enzyme-linked secondary antibodies for 1 h at
room temperature. Following PBST cleaning, luminescence was recorded with Lumin4000 from GE, USA, and ECL reagent from BioSharp. All antibodies used are listed in Supplementary Table 3.
SUBCUTANEOUS TRANSPLANTATION TUMOR CXCL7-OE and NC RPMI8226 cells were harvested during logarithmic growth, washed with PBS, and resuspended in the appropriate volume of PBS to achieve a
density of f 5×10^6/100 μL. The cells were then subcutaneously injected into B-NDG mice within 2.5 h. Five randomly assigned 5-week-old female mice were divided into NC group and CXCL7-OE
group. After alcohol disinfection, the mice were inoculated with 100 μL of cell suspension on the outside of the right upper limb and returned to their cages for further observation. The
mice were monitored regularly for tumor growth, and tumor volumes were measured twice weekly using calipers. Mice were euthanized at the experimental endpoint, and tumors were excised for
further analysis, including histopathological and molecular investigations. XENOGRAFT TUMOR MODEL IN MICE THROUGH INTRAVENOUS INJECTION CXCL7-OE and NC RPMI8226 cells, harvested during
logarithmic growth, were washed with PBS and resuspended in PBS at a concentration of 10^7 cells per 100 µL. The cell suspension was injected into the tail vein of 5-week-old female B-NDG
mice within 2.5 h of preparation. Mice were randomly assigned into two groups: the NC group and the CXCL7-OE group, with five mice per group. Prior to injection, the tail veins were
sterilized with cotton balls soaked in a 75% alcohol solution to promote vein dilation. A total volume of 100 µL of the cell suspension was injected in the direction of blood flow. Tumor
growth and development were monitored regularly throughout the experiment. At the experimental endpoint, mice were euthanized, and tissues, including lungs, liver, and bone, were harvested
for histological and molecular analyses. IMMUNOHISTOCHEMISTRY (IHC) ANALYSIS Mouse tumors were sampled and then embedded in paraffin and sectioned by a microtome. Immunohistochemistry was
performed according to the steps provided by the manufacturer of the immunohistochemistry kit (Sevier, China), in which the primary antibodies used are also listed in Supplementary Table 3.
CO-IMMUNOPRECIPITATION (CO-IP) To isolate the protein supernatant, cell lysates were subjected to centrifugation for 10–15 min. The resulting supernatant was incubated with the specific
antibody overnight at 4 °C. Following this, the immune complexes were captured by magnetic beads through a 1-hour incubation. To eliminate nonspecific binding, the beads underwent three
washes with IP buffer and one wash with distilled water. The immunoprecipitated proteins were then analyzed via WB. CO-CULTURE OF MM CELLS AND MOUSE BONE MARROW-DERIVED MACROPHAGES (BMDMS)
The co-culture system of MM cells and mouse BMDMs was established using a Transwell co-culture system. Logarithmically growing CXCL7-OE/NC RPMI8226 cells were seeded into the insert wells of
the Transwell at a density of 1×10^5 cells per well, while BMDMs were plated in the lower chamber at a density of 5 × 10^5 cells per well. To extract BMDMs, 8-week-old C57BL/6 mice were
euthanized by cervical dislocation following deep anesthesia. Both femoral bones were exposed and carefully cut open, and the bone marrow was collected using sterile scissors and forceps.
The extracted bone marrow was placed into a dish containing sterile PBS, and the marrow was gently squeezed out using a syringe piston. The resulting cell suspension was filtered through a
70 μm cell sieve to remove debris and bone fragments. The filtered suspension was transferred into a culture flask with a complete medium, where it was allowed to settle for 4 h to remove
non-adherent cells. The supernatant was then transferred into a new flask containing medium supplemented with M-CSF (2 ng/μL) and RANKL (20 ng/μL). After 6 days, osteoclast differentiation
and morphology in the lower chamber were assessed using TRAP staining. STATISTICAL ANALYSIS Continuous variables were analyzed using Student’s t-test for normally distributed data or the
Mann-Whitney U test for non-normally distributed data. PFS and OS probabilities were estimated using the Kaplan-Meier method, and differences in statistical significance were evaluated using
the two-sided log-rank test. Categorical data were assessed using the Chi-square test. Statistical analyses were performed using R, version 4.3.3 (http://cran.r-project.org). A p-value less
than 0.05 was considered statistically significant unless otherwise specified. All confidence intervals (CIs) were calculated at a 95% level. *_P_ < 0.05, **_P_ < 0.01 and ***_P_ <
0.001 were noted. REPORTING SUMMARY Further information on research design is available in the Nature Research Reporting Summary linked to this article. DATA AVAILABILITY The single-cell
RNA-seq processed gene expression data reported in this paper have been deposited into the CNGB Sequence Archive (CNSA) [31] of the China National GeneBank DataBase (CNGBdb) [32] with
accession number CNP0005613(CSE0000445), and raw sequencing data have been deposited in the OMIX, China National Center for Bioinformation, Chinese Academy of Sciences (accession no.
OMIX007530) [33]. To request access to raw sequencing data, please apply at the Human Genetic Resources Service System of the Ministry of Science and Technology (https://apply.hgrg.net/) in
accordance with the Regulations on the Management of Human Genetic Resources of China. The multiple myeloma RNA-seq data GSE24080 were downloaded from the GEO database. REFERENCES * Rajkumar
SV. Treatment of multiple myeloma. Nat Rev Clin Oncol. 2011;8:479–91. Article CAS PubMed PubMed Central Google Scholar * Chng WJ, Dispenzieri A, Chim CS, Fonseca R, Goldschmidt H,
Lentzsch S, et al. IMWG consensus on risk stratification in multiple myeloma. Leukemia. 2014;28:269–77. Article CAS PubMed Google Scholar * van de Donk N, Pawlyn C, Yong KL. Multiple
myeloma. Lancet. 2021;397:410–27. Article PubMed Google Scholar * Tanimoto K, Hiasa M, Tenshin H, Teramachi J, Oda A, Harada T, et al. Mechanical unloading aggravates bone destruction and
tumor expansion in myeloma. Haematologica. 2022;107:744–9. Article CAS PubMed Google Scholar * Terpos E, Christoulas D, Gavriatopoulou M, Dimopoulos MA. Mechanisms of bone destruction
in multiple myeloma. Eur J Cancer Care (Engl). 2017;26. * Mempel TR, Lill JK, Altenburger LM. How chemokines organize the tumour microenvironment. Nat Rev Cancer. 2024;24:28–50. Article CAS
PubMed Google Scholar * Ozga AJ, Chow MT, Luster AD. Chemokines and the immune response to cancer. Immunity. 2021;54:859–74. Article CAS PubMed PubMed Central Google Scholar * Zhou
Z, Xia G, Xiang Z, Liu M, Wei Z, Yan J, et al. A C-X-C Chemokine Receptor Type 2-Dominated Cross-talk between Tumor Cells and Macrophages Drives Gastric Cancer Metastasis. Clin Cancer Res.
2019;25:3317–28. Article CAS PubMed PubMed Central Google Scholar * Lim WK, Chai X, Ghosh S, Ray D, Wang M, Rasheed SAK, et al. Galpha-13 induces CXC motif chemokine ligand 5 expression
in prostate cancer cells by transactivating NF-kappaB. J Biol Chem. 2019;294:18192–206. Article CAS PubMed PubMed Central Google Scholar * Wang YH, Shen CY, Lin SC, Kuo WH, Kuo YT, Hsu
YL, et al. Monocytes secrete CXCL7 to promote breast cancer progression. Cell Death Dis. 2021;12:1090. Article CAS PubMed PubMed Central Google Scholar * Fang W, You J, Xu Q, Jiang Y,
Hu H, Chen F, et al. Plasma Exosomal CXCL7 is a Potential Biomarker for Lung Adenocarcinoma. Clin Lab. 2022;68. * Kinouchi T, Uemura M, Wang C, Ishizuya Y, Yamamoto Y, Hayashi T, et al.
Expression level of CXCL7 in peripheral blood cells is a potential biomarker for the diagnosis of renal cell carcinoma. Cancer Sci. 2017;108:2495–502. Article CAS PubMed PubMed Central
Google Scholar * Barlogie B, Tricot G, Rasmussen E, Anaissie E, van Rhee F, Zangari M, et al. Total therapy 2 without thalidomide in comparison with total therapy 1: role of intensified
induction and posttransplantation consolidation therapies. Blood. 2006;107:2633–8. Article CAS PubMed Google Scholar * van Rhee F, Szymonifka J, Anaissie E, Nair B, Waheed S, Alsayed Y,
et al. Total Therapy 3 for multiple myeloma: prognostic implications of cumulative dosing and premature discontinuation of VTD maintenance components, bortezomib, thalidomide, and
dexamethasone, relevant to all phases of therapy. Blood. 2010;116:1220–7. Article PubMed PubMed Central Google Scholar * Yang X, Chen X, Zhang S, Fan W, Zhong C, Liu T, et al. Collagen
1-mediated CXCL1 secretion in tumor cells activates fibroblasts to promote radioresistance of esophageal cancer. Cell Rep. 2023;42:113270. Article CAS PubMed Google Scholar * Du J, Lin
Z, Fu XH, Gu XR, Lu G, Hou J. Research progress of the chemokine/chemokine receptor axes in the oncobiology of multiple myeloma (MM). Cell Commun Signal. 2024;22:177. Article PubMed PubMed
Central Google Scholar * Chen M, Wan Y, Li X, Xiang J, Chen X, Jiang J, et al. Dynamic single-cell RNA-seq analysis reveals distinct tumor program associated with microenvironmental
remodeling and drug sensitivity in multiple myeloma. Cell Biosci. 2023;13:19. Article CAS PubMed PubMed Central Google Scholar * Matsumoto T, Abe M. Bone destruction in multiple
myeloma. Ann N. Y Acad Sci. 2006;1068:319–26. Article CAS PubMed Google Scholar * Bikfalvi A, Billottet C. The CC and CXC chemokines: major regulators of tumor progression and the tumor
microenvironment. Am J Physiol Cell Physiol. 2020;318:C542–C54. Article PubMed PubMed Central Google Scholar * Marcuzzi E, Angioni R, Molon B, Cali B Chemokines and Chemokine Receptors:
Orchestrating Tumor Metastasization. Int J Mol Sci. 2018;20. * Yan J, Zhao Q, Wang J, Tian X, Wang J, Xia X, et al. FGL2-wired macrophages secrete CXCL7 to regulate the stem-like
functionality of glioma cells. Cancer Lett. 2021;506:83–94. Article CAS PubMed PubMed Central Google Scholar * Guo Q, Jian Z, Jia B, Chang L. CXCL7 promotes proliferation and invasion
of cholangiocarcinoma cells. Oncol Rep. 2017;37:1114–22. Article CAS PubMed Google Scholar * Fu J, Li S, Feng R, Ma H, Sabeh F, Roodman GD, et al. Multiple myeloma-derived MMP-13
mediates osteoclast fusogenesis and osteolytic disease. J Clin Invest. 2016;126:1759–72. Article PubMed PubMed Central Google Scholar * Lo CH, Shay G, McGuire JJ, Li T, Shain KH, Choi
JY, et al. Host-Derived Matrix Metalloproteinase-13 Activity Promotes Multiple Myeloma-Induced Osteolysis and Reduces Overall Survival. Cancer Res. 2021;81:2415–28. Article CAS PubMed
PubMed Central Google Scholar * Johnson DE, O’Keefe RA, Grandis JR. Targeting the IL-6/JAK/STAT3 signalling axis in cancer. Nat Rev Clin Oncol. 2018;15:234–48. Article CAS PubMed PubMed
Central Google Scholar * Bharti AC, Shishodia S, Reuben JM, Weber D, Alexanian R, Raj-Vadhan S, et al. Nuclear factor-kappaB and STAT3 are constitutively active in CD138+ cells derived
from multiple myeloma patients, and suppression of these transcription factors leads to apoptosis. Blood. 2004;103:3175–84. Article CAS PubMed Google Scholar * Yu H, Lee H, Herrmann A,
Buettner R, Jove R. Revisiting STAT3 signalling in cancer: new and unexpected biological functions. Nat Rev Cancer. 2014;14:736–46. Article CAS PubMed Google Scholar * Marquez-Curtis LA,
Dobrowsky A, Montano J, Turner AR, Ratajczak J, Ratajczak MZ, et al. Matrix metalloproteinase and tissue inhibitors of metalloproteinase secretion by haematopoietic and stromal precursors
and their production in normal and leukaemic long-term marrow cultures. Br J Haematol. 2001;115:595–604. Article CAS PubMed Google Scholar * Vandooren J, Van den Steen PE, Opdenakker G.
Biochemistry and molecular biology of gelatinase B or matrix metalloproteinase-9 (MMP-9): the next decade. Crit Rev Biochem Mol Biol. 2013;48:222–72. Article CAS PubMed Google Scholar *
Cox TR. The matrix in cancer. Nat Rev Cancer. 2021;21:217–38. Article CAS PubMed Google Scholar * Guo X, Chen F, Gao F, Li L, Liu K, You L, et al. CNSA: a data repository for archiving
omics data. Database (Oxford). 2020;2020. * Chen FZ, You LJ, Yang F, Wang LN, Guo XQ, Gao F, et al. CNGBdb: China National GeneBank DataBase. Yi Chuan. 2020;42:799–809. PubMed Google
Scholar * Members C-N, Partners. Database Resources of the National Genomics Data Center, China National Center for Bioinformation in 2024. Nucleic Acids Res. 2024;52:D18–D32. Article
Google Scholar Download references FUNDING Science and Technology Innovation Plan of Shanghai Science and Technology Commission (21YF1406300). Natural Science Foundation of Shanghai
Municipality (22ZR1411400). Youth Foundation of Zhongshan hospital Fudan University (2021ZSQNN61). AUTHOR INFORMATION Author notes * These authors contributed equally: Yue Wang, Tianwei Lan.
AUTHORS AND AFFILIATIONS * Department of Hematology, Zhongshan Hospital, Fudan University, Shanghai, China Yue Wang, Tianwei Lan, Chi Zhou & Peng Liu * Department of Pathology,
Zhongshan Hospital, Fudan University, Shanghai, China Qiongyan Zhang Authors * Yue Wang View author publications You can also search for this author inPubMed Google Scholar * Tianwei Lan
View author publications You can also search for this author inPubMed Google Scholar * Qiongyan Zhang View author publications You can also search for this author inPubMed Google Scholar *
Chi Zhou View author publications You can also search for this author inPubMed Google Scholar * Peng Liu View author publications You can also search for this author inPubMed Google Scholar
CONTRIBUTIONS The conceptualization of this study was accomplished by YW, TL, and PL. YW and TL were responsible for developing the methodology. CZ played the role of the assistant. Data
collection was jointly carried out by YW, TL, and CZ. The determination of the pathological sections and immunohistochemical results was accomplished by QZ. The original draft of the writing
was produced by YW and TL. Finally, the review and editing of the writing were meticulously handled by PL. CORRESPONDING AUTHOR Correspondence to Peng Liu. ETHICS DECLARATIONS COMPETING
INTERESTS The authors declare no competing interests. ETHICS APPROVAL AND CONSENT TO PARTICIPATE The study was approved by the Ethics Committee of Fudan University Zhong Hospital
(Observation Research Cohort of Plasma Cell Diseases in Zhongshan Hospital, Registration Number: B2017-031R)) and complied with the principles of the Helsinki Accord. Bone marrow samples
were collected from all subjects, with patient consent, and stored in the tissue bank at Fudan University Zhongshan Hospital. The animal experiments were approved by the Animal Ethics
Committee of Zhongshan Hospital Fudan University. ADDITIONAL INFORMATION PUBLISHER’S NOTE Springer Nature remains neutral with regard to jurisdictional claims in published maps and
institutional affiliations. Edited by Anastasis Stephanou SUPPLEMENTARY INFORMATION SUPPLEMENTARY TABLE 1 SUPPLEMENTARY TABLE 2 SUPPLEMENTARY TABLE 3 SUPPLEMENTARY FIGURE S1 ORIGINAL DATA
FILE REPORTING SUMMARY RIGHTS AND PERMISSIONS OPEN ACCESS This article is licensed under a Creative Commons Attribution 4.0 International License, which permits use, sharing, adaptation,
distribution and reproduction in any medium or format, as long as you give appropriate credit to the original author(s) and the source, provide a link to the Creative Commons licence, and
indicate if changes were made. The images or other third party material in this article are included in the article’s Creative Commons licence, unless indicated otherwise in a credit line to
the material. If material is not included in the article’s Creative Commons licence and your intended use is not permitted by statutory regulation or exceeds the permitted use, you will
need to obtain permission directly from the copyright holder. To view a copy of this licence, visit http://creativecommons.org/licenses/by/4.0/. Reprints and permissions ABOUT THIS ARTICLE
CITE THIS ARTICLE Wang, Y., Lan, T., Zhang, Q. _et al._ Myeloma cell-derived CXCL7 facilitates proliferation of tumor cells and occurrence of osteolytic lesions through JAK/STAT3 pathway.
_Cell Death Dis_ 16, 74 (2025). https://doi.org/10.1038/s41419-025-07413-6 Download citation * Received: 05 October 2024 * Revised: 13 January 2025 * Accepted: 30 January 2025 * Published:
06 February 2025 * DOI: https://doi.org/10.1038/s41419-025-07413-6 SHARE THIS ARTICLE Anyone you share the following link with will be able to read this content: Get shareable link Sorry, a
shareable link is not currently available for this article. Copy to clipboard Provided by the Springer Nature SharedIt content-sharing initiative