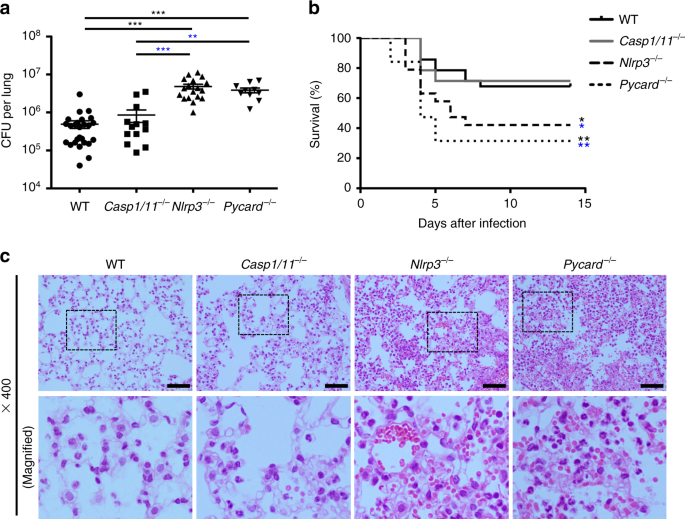
- Select a language for the TTS:
- UK English Female
- UK English Male
- US English Female
- US English Male
- Australian Female
- Australian Male
- Language selected: (auto detect) - EN
Play all audios:
ABSTRACT It is widely accepted that inflammasomes protect the host from microbial pathogens by inducing inflammatory responses through caspase-1 activation. Here, we show that the
inflammasome components ASC and NLRP3 are required for resistance to pneumococcal pneumonia, whereas caspase-1 and caspase-11 are dispensable. In the lung of _S_. _pneumoniae_-infected mice,
ASC and NLRP3, but not caspase-1/11, were required for optimal expression of several mucosal innate immune proteins. Among them, TFF2 and intelectin-1 appeared to be protective against
pneumococcal pneumonia. During infection, ASC and NLRP3 maintained the expression of the transcription factor SPDEF, which can facilitate the expression of the mucosal defense factor genes.
Moreover, activation of STAT6, a key regulator of _Spdef_ expression, depended on ASC and NLRP3. Overexpression of these inflammasome proteins sustained STAT6 phosphorylation induced by type
2 cytokines. Collectively, this study suggests that ASC and NLRP3 promote airway mucosal innate immunity by an inflammasome-independent mechanism involving the STAT6-SPDEF pathway. SIMILAR
CONTENT BEING VIEWED BY OTHERS MAFB REGULATES NLRP3 INFLAMMASOME ACTIVATION BY SUSTAINING P62 EXPRESSION IN MACROPHAGES Article Open access 16 October 2023 SARS-COV-2 N PROTEIN PROMOTES
NLRP3 INFLAMMASOME ACTIVATION TO INDUCE HYPERINFLAMMATION Article Open access 02 August 2021 INTERFERON SIGNALLING AND NON-CANONICAL INFLAMMASOME ACTIVATION PROMOTE HOST PROTECTION AGAINST
MULTIDRUG-RESISTANT _ACINETOBACTER BAUMANNII_ Article Open access 12 November 2024 INTRODUCTION The airway surface is continuously exposed to microbes and foreign particles that are inhaled
into the respiratory tract.1 The airway epithelium forms a protective mucosal barrier between the external and internal environments. Airway epithelial cells, including ciliated and
secretory cells, play a key role in the removal of inhaled microbes and particles through mucociliary clearance. Type II alveolar epithelial cells secrete surfactant proteins (SPs) that have
protective effects against bacterial pathogens. Hence, the airway epithelium is usually active to maintain an effective mucosal barrier, thereby protecting the host from microbial
colonization and infections. The Gram-positive bacterial pathogen _Streptococcus pneumoniae_ is a leading cause of pneumonia, meningitis, and septicemia and is responsible for significant
mortality and morbidity worldwide.2 The polysaccharide capsule and the cytolysin pneumolysin (PLY) are critical virulence factors of _S. pneumoniae_. PLY is a member of cholesterol-dependent
cytolysins and forms ring- or arc-shaped pores on cholesterol-containing membranes. PLY plays a critical role in the pathogenicity of _S. pneumoniae_ by disrupting tissue barriers,
suppressing ciliary beating on epithelial cells, and inhibiting bactericidal activity of neutrophils.3,4 Pattern-recognition receptors (PRRs), such as Toll-like receptors (TLRs) and Nod-like
receptors (NLRs), play a central role in innate immunity.5 PRRs sense specific pathogen-derived molecular structures and, in turn, provide signals that activate innate and acquired
immunity. NLR family, pyrin domain-containing 3 (NLRP3, also known as Cryopyrin) and absent in melanoma 2 (AIM2) are cytoplasmic PRRs, which sense potassium efflux and cytosolic DNA,
respectively.6,7 After recognition of specific agonizts, NLRP3 and AIM2 form inflammasomes. Inflammasomes are cytoplasmic multiprotein complexes that recruit and activate caspase-1, which in
turn processes pro-interleukin (IL)-1β and pro-IL-18 into their respective bioactive forms. Apoptosis-associated speck-like protein containing a CARD (ASC, also known as PYCARD) is an
inflammasome adapter protein that is required for the formation of the AIM2 and NLRP3 inflammasomes. After inflammasome activation, ASC also forms the ASC speck, a supramolecular aggregate
of ASC dimers, which serves as another platform for the activation of caspase-1. Pneumococcal components and products are sensed by several PRRs, leading to the induction of inflammatory
mediators, some of which contribute to host defense against _S. pneumoniae_.8 Previous studies have suggested that _S. pneumoniae_ induces the activation of caspase-1 and secretion of
bioactive IL-1β and IL-18 in a PLY-dependent manner and that the AIM2 and NLRP3 inflammasomes mediate the _S. pneumoniae_-induced activation of caspase-1 and secretion of these
cytokines.8,9,10,11,12 Except for one study which demonstrated that ASC and NLRP3 impair host defense against serotype 3 _S_. _pneumoniae_,13 most of previous works have shown that mice
deficient in either NLRP3 or ASC were more susceptible to pneumococcal pneumonia than wild-type (WT) mice, suggesting the importance of the NLRP3 inflammasome in host defense against _S.
pneumoniae_.9,10,12 Moreover, IL-1β and IL-18 have been suggested to play a protective role in pneumococcal pneumonia.14,15,16 In light of the previous observations, one could propose a
simple model, in which caspase-1 contributes to host defense in pneumococcal pneumonia through the processing of IL-1β and IL-18 following NLRP3 inflammasome activation. However, our recent
experiments demonstrated that the resistance of caspase-1/caspase-11-deficient mice to pneumococcal pneumonia was comparable to that of WT mice. Besides, although NLRP3 is required for
optimal host defense, substantial levels of IL-1β and IL-18 were detected in bronchoalveolar lavage fluid (BALF) of NLRP3-deficient mice infected with _S. pneumoniae_. Accordingly, NLRP3 and
ASC might protect the host from _S. pneumoniae_ by a yet unidentified mechanism, which is independent of caspase-1. In this study, we tried to elucidate the mechanism, by which NLRP3 and
ASC contribute to the control of pneumococcal pneumonia in an inflammasome-independent manner. RESULTS NLRP3 AND ASC, BUT NOT CASPASE-1/11, ARE REQUIRED FOR THE RESISTANCE AGAINST _S.
PNEUMONIAE_ To examine the role of inflammasome proteins in pneumococcal pneumonia, WT mice and mice deficient in caspase-1, ASC, or NLRP3 were infected intranasally with _S. pneumoniae_. As
previously reported, ASC-deficient (_Pycard_−/−) and _Nlrp3_−/− mice showed higher bacterial burdens in the lung and lower survival rates than WT mice after _S. pneumoniae_ infection (Fig.
1a, b).9,10,12 However, there was no significant difference between WT and caspase-1/11-deficient (_Casp1/11_−/−) mice in survival and bacterial counts in the lung. Moreover, severe tissue
damage was observed in the lung of _Pycard_−/− or _Nlrp3_−/− mice after _S. pneumoniae_ infection, while the pathogen caused less lung tissue damage in WT or _Casp1/11_−/− mice (Fig. 1c).
Many red blood cells were seen in the alveolar spaces of _Pycard_−/− or _Nlrp3_−/− mice, but not or less in those of WT or _Casp1/11_−/− mice. These results suggest that ASC and NLRP3
protect the host from pneumococcal pneumonia in an inflammasome-independent manner. CYTOKINES AND NEUTROPHIL INFILTRATION DO NOT EXPLAIN ANTIPNEUMOCOCCAL DEFENSE MEDIATED BY NLRP3 To
elucidate the mechanism, by which ASC and NLRP3 mediate host defense against pneumococcal pneumonia, we examined the role of these inflammasome proteins in innate immune responses to _S.
pneumoniae_, such as cytokine production and neutrophil recruitment to the lung. As previously described, BALF levels of IL-1β and IL-18 were significantly lower in _Pycard_−/− mice than in
WT mice after _S. pneumoniae_ infection (Fig. 2a, b).9 However, as low, but measurable, levels of these cytokines were detected in BALF of _Pycard_−/− mice, it is premature to conclude that
IL-1 and IL-18 signals were abolished in _Pycard_−/− mice. Besides, IL-1α, which signals through the same receptor as IL-1β, was produced during _S. pneumoniae_ infection even in the absence
of ASC, NLRP3, or caspase-1/11 (Fig. 2c). Substantial levels of IL-1β and IL-18 were detected in BALF of _Nlrp3_−/− mice (Fig. 2a, b); the protective action of NLRP3 is unlikely to involve
the production of these cytokines. In _Casp1/11_−/− mice, although the production of IL-18 in response to _S. pneumoniae_ was diminished, that of IL-1β was normal (Fig. 2a, b). The levels of
IL-6, TNFα, IL-10, and IFN-γ in BALF were substantially increased after _S. pneumoniae_ infection in all groups tested (Fig. 2d–g). IL-17A was not significantly increased in BALF after
infection with _S. pneumoniae_ (Fig. 2h). In addition, the number of total cells, neutrophils, and macrophages in BALF were not significantly different among the four groups after _S.
pneumoniae_ infection (Fig. 2i, j; data not shown). Neutrophil-derived serine proteases (NSPs) have been proposed to kill _S. pneumoniae_. The activity of NSPs in BALF samples was increased
after infection with _S. pneumoniae_; there was no significant difference in the activity among WT, _Casp1/11_−/−, _Nlrp3_−/− and _Pycard_−/− mice (Fig. 2k). Hence, it is suggested that
NLRP3 and ASC are dispensable for the recruitment of neutrophils and release of NSPs. Furthermore, we tested whether ASC and NLRP3 in cells of hemopoietic origin is involved in host defense
against pneumococcal pneumonia using irradiated bone marrow chimeric mice. There was no significant difference in bacterial counts in the lung between WT recipient mice reconstituted with
bone marrow cells from WT mice and those from _Pycard_-/- mice or _Nlrp3_−/− mice (Fig. 2l; Fig. S1). From these results, we speculate that NLRP3 and ASC protect the host from _S.
pneumoniae_ through mechanisms other than inflammasome-dependent cytokine production by immune cells. ASC- AND NLRP3-DEPENDENT GENE EXPRESSION IN THE LUNG To know how ASC and NLRP3 protect
the host from _S. pneumoniae_, we attempted to identify genes regulated by ASC and NLRP3 and performed a microarray analysis using total RNAs extracted from whole lungs of WT and mutant mice
that had been infected with _S. pneumoniae_. The microarray analysis revealed 213 genes and non-coding RNAs (there are overlapping) that were downregulated by deficiency of ASC or NLRP3,
but not by that of caspase-1/11 (Fig. 3a; Table S1). Several of these genes encode mucosal innate immune proteins, such as regenerating islet-derived 3γ (REG3γ), trefoil factor 2 (TFF2), BPI
fold containing family A member 1 (BPIFA1), BPIFB1, intelectin-1, and mucin 5b (Fig. 3a).17,18,19,20,21,22,23,24,25 On the other hand, the expression of genes coding for other markers of
pulmonary epithelial cells (SCGB1A1, SP-A, SP-B, SP-C, SP-D, podoplanin, aquaporin-5, E-cadherin, and epithelial cell adhesion molecule) and housekeeping proteins (GAPDH, β-actin, and
tubulins) was not decreased in the absence of caspase-1, ASC, or NLRP3 (Table S2). Moreover, deficiency of NLRP3 or ASC did not reduce the expression of plasminogen activator inhibitor-1
(also known as SERPINE1), which has been reported to prevent severe lung injury after _S. pneumoniae_ infection (Table S2).26 We confirmed by quantitative RT-PCR that the expression of
_Reg3g_, _Tff2_, _Bpifa1_, and _Bpifb1_ was lower in the lung of _Pycard_−/− or _Nlrp3_−/− mice than in that of WT or _Casp1/11_−/− mice, (Fig. 3b–e). The expression of _Muc5b_ and _Itln1_
showed a tendency to be decreased in the absence of ASC or NLRP3 (Fig. 3f–g). Protein levels of TFF2, BPIFA1, intelectin-1, and mucin 5b were significantly lower in _Pycard_−/− and
_Nlrp3_−/− mice than in WT mice (Fig. 3h–k). Taken together, ASC and NLRP3 appear to regulate the expression of several pulmonary epithelial genes, whose products might be involved in innate
immunity and mucosal barrier function, in an inflammasome-independent manner. Notably, in WT mice, the expression of _Reg3g_, _Tff2_, and _Bpifa1_ was not increased by _S. pneumoniae_
infection; rather, that of _Tff2_ and _Bpifa1_ was decreased during infection (Fig. S2). In ASC-deficient mice, expression levels of these genes were significantly lower than those in WT and
caspase-1/11-deficient mice both before and during _S. pneumoniae_ infection (Fig. S2). On the other hand, loss of NLRP3 did not affect the expression of _Reg3g_, _Tff2_, and _Bpifa1_ in
the lung of uninfected mice, whereas the expression was more severely reduced in NLRP3-deficient mice than in WT and caspase-1/11-deficient mice during _S. pneumoniae_ infection (Fig. S2).
These results suggest that ASC maintains the expression of _Reg3g_, _Tff2_, and _Bpifa1_ under normal conditions and that ASC and NLRP3 have an inhibitory effect on the reduction in the
expression of the mucosal defense genes during _S. pneumoniae_ infection. We also found that _Reg3g_ expression in the distal jejunum was significantly lower in the absence of ASC than in
its presence (Fig. S3), supporting that ASC is involved in the expression of this gene. INVOLVEMENT OF TFF2 AND INTELECTIN-1 IN HOST DEFENSE AGAINST PNEUMOCOCCAL PNEUMONIA It has been
reported that REG3γ, BPIFA1, and intelectin-1 bind to certain bacteria to kill, inhibit the growth, or assist phagocytosis of them. REG3γ has been shown to minimally bind with _S.
pneumoniae_.17,20,22,25,27 We analyzed the binding ability of BPIFA1, BPIFB1, intelectin-1, and TFF2 to _S. pneumoniae_. Flag-tagged proteins of BPIFA1, BPIFB1, and TFF2 were not pulled-down
with _S. pneumoniae_ cells (Fig. 4a), suggesting that these proteins hardly bind to pneumococci. On the other hand, intelectin-1 showed binding to _S. pneumoniae_ (Fig. 4a), suggesting a
possible contribution of this protein to the protection against pneumococcal pneumonia. The epithelial repair factor TFF2 has recently been implicated in host defense against microbial
infections.19,28 We examined the effect of TFF2, intelectin-1, and BPIFA1 on pneumococcal pneumonia. _Pycard_−/− mice were transfected intranasally with expression vectors for TFF2,
intelectin-1, or BPIFA1 or an empty vector and then infected with _S. pneumoniae_. Flag-tagged TFF2 was detected in BALF from TFF2-transfected mice, but not in that from empty
vector-transfected mice (Fig. 4b). The levels of intelectin-1 were increased in the lung of _Pycard_−/− mice after transfection with intelectin-1 (Fig. 4c). Strikingly, bacterial counts in
the lung of the TFF2 and intelectin-1 vector groups were lower than those in the lung of the empty vector group (Fig. 4d, e). On the other hand, bacterial counts in the lung of the BPIFA1
vector group showed no difference comparing with those in the empty vector group (Fig. 4e). These results suggest that TFF2 and intelectin-1 are protective against pulmonary pneumococcal
infection. ASC AND NLRP3 ARE DISPENSABLE FOR THE ACTIVATION OF STAT3 AND MAPKS Previous studies have suggested STAT3 and MAPK pathways regulate the expression of _Reg3g_, _Bpifa1_, _Bpifb1_,
_Muc5b_, _Scgb3a1_, and _Tff2_.29,30,31 In addition, a study suggested ASC can mediate the activation of STAT3 and activator protein 1 (AP-1).32 Then, we tested whether ASC and NLRP3 are
involved in the activation of STAT3 and MAPK in the lung. Although phosphorylated STAT3 (phospho-STAT3) was detected in airway epithelial cells in both infected and uninfected mice, it was
not reduced in the absence of ASC or NLRP3 (Fig. S4A–C). Moreover, phospho-JNK and phospho-extracellular signal-regulated kinases 1/2 (ERK), upstream signaling kinases of AP-1, were not
significantly lower in the lung of _Pycard_−/− mice and _Nlrp3_−/− mice than in that of WT mice (Fig. S4A, B). Hence, activation of STAT3, JNK, and ERK is unlikely to depend on ASC and
NLRP3. The transcriptional regulator NKX2-1 (also known as TTF1) has been presented to have an inhibitory effect on the expression of _Reg3g_, _Tff2_, _Muc5b_, and _Itln1_.33 Expression
levels of _Nkx2-1_ in the lung were similar in all groups tested (Fig. S4D, E; Table S2), ruling out a role for ASC and NLRP3 in regulating NKX2-1 expression. ASC AND NLRP3 FACILITATE STAT6
ACTIVATION AND SPDEF EXPRESSION Sterile alpha motif pointed domain-containing ets transcription factor (SPDEF), in contrast to NKX2-1, has been shown to positively regulate the expression of
_Reg3g_, _Tff2_, _Muc5b_, and _Itln1_ directly or through differentiation of secretory cells (GSE44101).34,35,36 In HEK293 cells, forced expression of SPDEF increased the promoter activity
of the _Reg3g_ gene, confirming this transcription factor activates the expression of this gene (Fig. 5a). On the other hand, forced expression of ASC and a constitutively active mutant of
NLRP3 (NLRP3_R258W) did not increase the promoter activity of the _Reg3g_ gene, suggesting that the NLRP3-ASC pathway has no direct effect on the expression of this gene. We found that the
expression of _Spdef_ in the lung was significantly reduced during _S. pneumoniae_ infection in _Pycard_−/− and _Nlrp3_−/− mice, whereas the infection did not affect it in WT and
_Casp1/11_−/− mice (Fig. 5b). These results suggest that ASC and NLRP3 positively regulate the expression of SPDEF during _S. pneumoniae_ infection, thereby maintaining the expression of
SPDEF-regulated airway defense factors. _Spdef_ is a STAT6 target gene; for example, IL-13, a type 2 cytokine, has been shown to increase SPDEF expression in a STAT6-dependent manner.37
Accordingly, we tested whether STAT6 signaling is activated during _S. pneumoniae_ infection. Phospho-STAT6 was increased in the airway epithelium during pneumococcal pneumonia (Fig. 5c;
Fig. S5). Notably, the _S. pneumoniae_ induction of phospho-STAT6 was attenuated in _Pycard_−/− and _Nlrp3_−/− mice. Moreover, the expression of representative STAT6-responsive genes,
_Muc5ac_ and _Clca3_, was significantly lower in the lung of _Pycard_−/− mice than in that of WT mice. Pulmonary expression of _Muc5ac_ and _Clca3_ was also significantly lower in _Nlrp3_−/−
mice than in WT mice (Fig. 5d, e). It is therefore suggested that ASC and NLRP3 are involved in STAT6 activation during pneumococcal pneumonia. ASC AND NLRP3 SUSTAIN STAT6 PHOSPHORYLATION
Type 2 cytokines, including IL-4 and IL-13, induce STAT6 activation.38 The expression of _IL4_ was not detectable by real-time RT-PCR (data not shown). Although the expression of _IL13_ in
the lung of _S. pneumoniae_-infected mice tended to be reduced by deficiency of ASC or NLRP3, IL-13 protein levels in BALF were not significantly different among WT, _Casp1/11_−/−,
_Nlrp3_−/−, and _Pycard_−/− mice (Fig. 6a, b). IL-25, IL-33, and TSLP (thymic stromal lymphopoietin) have been demonstrated to regulate innate type 2 responses.38 The expression of these
genes in the lung of _Nlrp3_−/− and _Pycard_−/− mice was comparable to that in WT mice (Fig. 6c–e). We also measured the levels of IL-33 in BALF by ELISA, as IL-33 lacks a canonical
secretory signal sequence and is released upon necrotic cell death as an alarmin. The levels of IL-33 in BALF of uninfected and infected mice were under the detection limit of the ELISA kit;
nonetheless, _S. pneumoniae_ infection seemed to reduce BALF levels of IL-33 in the absence of ASC or NLRP3 (Fig. 6f). However, genetic ablation of IL-1R-like 1 (also known as ST2), a
receptor for IL-33,39 did not affect the expression of _Spdef_, _Muc5ac_, and _Tff2_ in the lung of _S. pneumoniae_-infected mice, though _Reg3g_ expression was slightly lower in IL-1R-like
1-deficient mice than in WT mice (Fig. 6g–j). Judging from these results, it is unlikely that IL-33 links ASC and NLRP3 to STAT6 activation and the expression of the mucosal defense genes
during pneumococcal pneumonia. To examine the direct effect of NLRP3 and ASC on STAT6 activation, HEK293 cells transfected with STAT6 were stimulated with recombinant (r) IL-13 or IL-4,
which has been demonstrated to induce STAT6 phosphorylation in this experimental setting.40 Phosphorylated STAT6 was detected in STAT6-transfected HEK293 cells after treatment with rIL-13/4
(Fig. 7a–d; Fig. S6). STAT6 phosphorylation peaked at 1 or 2 h after rIL-13/4 treatment, while it gradually decreased at later time points, suggesting that STAT6 phosphorylation is
suppressed by a negative feedback mechanism during the treatment. Surprisingly, when HEK293 cells were co-transfected with ASC plus NLRP3_R258W, the decrease in STAT6 phosphorylation at the
later time points after rIL-13/4 treatment was less pronounced than when the cells were co-transfected with the control vector (Fig. 7a, c; Fig. S6). On the other hand, co-transfection with
ASC plus NLRP3_R258W did not sustain STAT6 phosphorylation if the cells were treated with rIL-13 or rIL-4 for 1 h and then washed to remove the cytokines (Fig. 7b, d; Fig. S6). From these
results, it is conceivable that NLRP3 and ASC sustain phosphorylation of STAT6 by inhibiting a negative feedback mechanism, which controls type 2 cytokine induction of STAT6 activation.
DISCUSSION It is well-established that caspase-1 is a central effector of inflammasomes. In fact, a number of studies have suggested that inflammasomes exert their physiological and
pathological effects by activating caspase-1, which leads to pyroptosis and production of IL-1β and IL-18.6,7 In this study, we found that NLRP3 and ASC, but not caspase-1/11, play a
critical role in host defense against pneumococcal pneumonia. We also found that during _S. pneumoniae_ infection, NLRP3 and ASC maintain the expression of several mucosal defense genes in
an inflammasome-independent manner. Thus, this study provides an insight into innate immune homeostasis in airway mucosa. A recent report has demonstrated that during _S. pneumoniae_
infection, NLRP3 protects the lung epithelial barrier by increasing the adherence of alveolar epithelial cells.41 The study also showed that this protective effect of NLRP3 is independent of
ASC, caspase-1, and IL-1/IL-18. On the other hand, we found that both NLRP3 and ASC are involved in the expression of mucosal defense factors upon pneumococcal pneumonia. Hence, the
previous study and our study discovered different inflammasome-independent roles for NLRP3 in host defense against _S. pneumoniae_, and it is conceivable that NLRP3 protects the host from
this pathogen by multiple mechanisms. A previous study has suggested that IL-18-deficient mice are more susceptible to pneumococcal pneumonia than WT mice.15 As most of IL-18 production was
caspase-1-dependent during _S. pneumoniae_ infection, we had expected that _Casp1/11_−/− mice are susceptible to this bacterial pathogen. However, unexpectedly, the resistance of
_Casp1/11_−/− mice to _S. pneumoniae_ was comparable to that of WT mice (Fig. 1). A possible explanation for this discrepancy is that IL-18 signaling was not completely abrogated in
caspase-1-deficient mice. Indeed, _S. pneumoniae_-induced IL-18 production was significantly impaired, but not completely abolished, in the absence of caspase-1/11 (Fig. 2b). Substantial
levels of IFN-γ were also produced in _Casp1/11_−/− mice (Fig. 2g). Therefore, it is conceivable that in _Casp1/11_−/− mice, the low levels of IL-18 were produced by a caspase-1-independent
mechanism. On the other hand, IL-18 signaling would be completely abrogated in IL-18-deficient mice, which might significantly affect the resistance to pneumococcal pneumonia. It should also
be noted that _Casp1/11_−/− mice, but not _Casp1_−/− mice, were used in this study. Therefore, the possibility that caspase-11 impairs host defense and expression of mucosal defense genes
cannot be ruled out and needs further investigations. TFF2 has been known to play critical roles in mucus gel formation and tissue repair. Although the precise mechanism by which TFF2
contributes to host defense against _S. pneumoniae_ remains unclear, this peptide perhaps promotes mucosal barrier function to control pneumococcal pneumonia. It has been reported that
TFF2-deficient mice are more susceptible to _Yersinia enterocolitica_ and the hookworm parasite _Nippostrongylus brasiliensis_,19,28 suggesting that TFF2 protects from different types of
pathogens. REG3γ and BPIFA1 have also been reported to play important roles in innate immune defense against Gram-positive and Gram-negative bacteria, respectively.25,27,42,43,44,45
Considering that these ASC- and NLRP3-dependent mucosal defense factors (TFF2, REG3γ, and BPIFA1) as a whole can provide protection against a broad range of microbial pathogens, it is worth
examining if NLRP3 and ASC also maintain the expression of mucosal defense genes during infection by other microbial pathogens and in other mucosal tissues. Although both ASC and NLRP3 were
required for the optimal expression of _Reg3g_, _Tff2_, and _Bpifa1_ during _S. pneumoniae_ infection, the former, but not the latter, was required for it in uninfected mice (Fig. S3). One
possible explanation for this is that ASC might maintain the expression of these genes dependently on NLRP3 upon pneumococcal pneumonia, but other receptors under normal conditions. This and
other possibilities will be investigated in future studies. _S. pneumoniae_ infection reduced the expression of mucosal defense genes, such as _Tff2_ and _Bpifa1_, in the lung (Fig. S3). It
has been suggested that IFN-γ inhibits mucus production, blocks goblet cell development, and promotes goblet cell apoptosis.46 As substantial levels of IFN-γ were produced in response to
_S. pneumoniae_ infection in all groups tested, it is possible that mucus-producing goblet cells were decreased by IFN-γ during pneumococcal pneumonia, resulting in the reduction in the
expression of mucosal defense genes. By contrast, SPDEF mediates differentiation of goblet cells and plays a critical role in the expression of mucosal defense genes, including _Reg3g_,
_Tff2_, _Muc5b_, and _Itln1_34,35,36 (GSE44101). Thus, we speculate that STAT6 activation, which is augmented by NLRP3 and ASC, maintains goblet cell differentiation and mucus production
through the expression of SPDEF, thereby counteracting the effect of IFN-γ. The mechanism by which STAT6 was activated in airway epithelium during _S. pneumoniae_ infection remains unclear.
Low levels of IL-13 mRNA and protein were detected in _S. pneumoniae_-infected mice (Fig. 6), implying that unlike allergens, this bacterium only weakly stimulates type 2 cytokine
production, leading to modest STAT6 activation. However, further experiments are required to elucidate the mechanism linking _S. pneumoniae_ infection to STAT6 activation. In conclusion, we
found that during pneumococcal pneumonia, ASC and NLRP3 maintain the expression of multiple mucosal defense genes in the airway mucosa, possibly by sustaining STAT6 activation in an
inflammasome-independent manner. Our results demonstrate a novel caspase-1-independent role for inflammasome proteins, which may help to control microbial infections. MATERIALS AND METHODS
REAGENTS Anti-caspase-1 Ab (sc-514) and anti-ASC Ab (sc-22514-R) were purchased from Santa Cruz Biotechnology (Santa Cruz, CA); anti-NLRP3 mAb (Cryo-2) from Enzo Life Sciences (Farmingdale,
NY); anti-β-actin mAb from Sigma-Aldrich (St. Louis, MO); anti-phosho-JNK Ab (4668), anti-phosho-ERK Ab (9101), and anti-phosho-STAT3 Ab (9145) from Cell Signaling Technology (Danvers, MA);
anti-phosho-STAT6 Ab (ab28829) from Abcam (Cambridge, MA); anti-Flag Ab from Rockland Immunochemicals (Gilbertsville, PA); anti-mouse CD16/32 mAb (101320), PE-labeled anti-mouse F4/80 mAb
(123109), and FITC-labeled anti-Gr-1 mAb (108405) from Biolegend (San Diego, CA); ELISA kits for mouse IL-1β, IL-6, IL-10, IL-13, IL-17A, IL-33, and TNFα from eBioscience; ELISA kits for
mouse TFF2, BPIFA1, intelectin-1, and mucin 5b from Cloud-Clone Corp. (Katy, TX); IL-18 ELISA kit from Medical & Biological Laboratories (Nagoya, Japan); anti-IFN-γ Abs and recombinant
IFN-γ from Thermo Scientific (Waltham, MA); recombinant hIL-4 and recombinant hIL-13 from PeproTech (Rocky Hill, NJ). MICE _Pycard_−/− mice generated by Prof. Shun-ichiro Taniguchi (Shinshu
University), _Casp1_−/− _Casp11_129mt/129mt mice generated by Dr. Keisuke Kuida (Millennium Pharmaceuticals), _Nlrp3__−/−_ mice generated by Prof. Jürg Tschopp (University of Lausanne), and
_Il1rl1__−/−_ mice generated by Prof. Shizuo Akira (Osaka University) were kindly gifted. The mutant and WT mice were on a C57BL/6 background. Mice were maintained in specific-pathogen-free
conditions and used at 8–10 weeks of age. All of the experimental procedures performed on mice were approved by the Animal Ethics and Research Committee of Kyoto University Graduate School
of Medicine, Kyoto, Japan (Med Kyo 13011), and by the Animal Ethics and Research Committee of Southwest University, Chongqing, China (Nos. 11–1025). BACTERIAL STRAIN _S. pneumoniae_ D39
(serotype 2) was purchased from the National Collection of Type Cultures (NCTC 7466; Central Public Health Laboratory, London, UK). Pneumococci were grown overnight on blood agar plates at
37 °C and 5% CO2. Colonies were inoculated into Todd-Hewitt broth (BD Biosciences, San Jose, CA) supplemented with 0.5% yeast extract, grown until mid-logarithmic phase (optical density at
600 nm [OD600] = 0.5), and centrifuged at 6000 × _g_ for 15 min. The bacterial pellet was suspended in phosphate-buffered saline (PBS) and stocked at −80 °C. The concentration was determined
by colony counting on blood agar plates. IN VIVO INFECTION EXPERIMENTS Mice were anesthetized with pentobarbitone (Nacalai tesque, Kyoto, Japan) and infected intranasally with _S.
pneumoniae_ in 30 μl PBS. Lungs collected from the infected mice were homogenized in PBS, serially diluted, and plated onto blood agar. CFUs were counted after overnight culture. BALF
samples were collected in 1 ml PBS. The collected BALF samples were first centrifuged at 200 × _g_ for 3 min at 4 °C, and the supernatants were transferred to new tubes for cytokine
detection. For intranasal transfection, 10 μg of each plasmid dissolved in 20 μl TE was mixed with 10 μl of Transfectin Lipid Reagent (Bio-Rad Laboratories, Hercules, CA), incubated for 15
min at room temperature, and inoculated into mice as above. FLOW CYTOMETRIC ANALYSIS The pellets of BALF were washed and the total cell number was counted. Totally, 106 cells from each
sample were incubated with anti-mouse CD16/32 mAb for 30 min at 4 °C. Then the cells were stained with PE-labeled anti-mouse F4/80 mAb and FITC-labeled anti-Gr-1 mAb for 30 min at 4 °C and
analyzed on a FACScalibur flow cytometer (BD Biosciences). The number of F4/80+ macrophages and F4/80− Gr-1+ neutrophils were calculated. ASSAY OF NEUTROPHIL SERINE PROTEASE ACTIVITY BALF
was diluted 10 times with NSP assay buffer (50 mM Tris-HCl pH7.4, 150 mM NaCl) and incubated with 1 mM N-Methoxysuccinyl-Ala-Ala-Pro-Val p-nitroanilide (MeOSuc-AAPV-pNA; Sigma-Aldrich) for
24 h at 37 °C, and the OD at 405 nm was measured with a plate reader. HISTOPATHOLOGY The lungs were perfused with 10 ml PBS, fixed with 10% formalin, and paraffin-embedded. Slides were
stained with hematoxylin/eosin or with anti-phospho-STAT3 Ab or anti-phospho-STAT6 Ab. MICROARRAY Total RNAs were extracted from whole lungs using TRIzol Reagent (Thermo Scientific) followed
by cleanup using NucleoSpin RNA Clean-up (MACHEREY-NAGEL, Düren, Germany). Microarray analysis was performed by using SurePrint G3 Mouse GE 8 × 60 K Microarray (Agilent, Santa Clara, CA) at
the Takara Bio Corporation (Shiga, Japan). Cy3-labeled cRNAs were synthesized using Low Input Quick Amp Labeling Kit One-Color (Agilent). Hybridization and washing were done by using Gene
Expression Hybridization Kit (Agilent) and Gene Expression Wash Buffers Pack (Agilent), respectively. Slides were scanned on an Agilent DNA Microarray Scanner G2565CA, and data were obtained
using Agilent Feature Extraction software. Microarray data have been submitted to GEO (NCBI) under the GSE accession number GSE56653. QUANTITATIVE RT-PCR Total RNAs were isolated from lungs
and small intestines as described above. The RNAs were reverse-transcribed using SuperScript VILO cDNA Synthesis Kit (Thermo Scientific). Quantitative real-time RT-PCR was carried out using
primers listed in Table S3 (Primer No. 1–34), EXPRESS SYBR GreenER qPCR Supermix with Premixed ROX (Thermo Scientific), and the Applied Biosystems PRISM 7000 (Thermo Scientific), according
to the manufacturer’s instructions. Relative mRNA level was calculated by the ΔCT method with _Actb_ mRNA for normalization. WESTERN BLOTTING ANALYSIS Proteins were extracted from whole
lungs using TRIzol Reagent. The protein samples (1 μg per sample) were subjected to sodium dodecyl sulphate (SDS)-polyacrylamide gel electrophoresis and subsequently transferred to
polyvinylidene difluoride membranes (Merck Millipore, Billerica, MA). The membranes were immunoblotted with antibodies indicated in each figure. PLASMIDS The full-length SPDEF cDNA was
amplified by PCR using primers Nos. 43 and 44 (Table S3) and then inserted into pFLAG-CMV2 vector (Sigma-Aldrich). The pFLAG expression vectors for TFF2, BPIFA1, BPIFB1, and intelectin-1
were constructed by using primers Nos. 35–42 (Table S3) and the pFLAG-CMV1 vector (Sigma-Aldrich). The expression vectors for ASC and NLRP3_R258W were constructed in our previous study.47
Luciferase reporter vector for the _Reg3g_ gene promoter was constructed by using primers Nos. 45 and 46 (Table S3) and the pGL3-Basic vector (Promega, Madison, WI). The reporter vector
pGL3-_Reg3g_ promoter contain −1501 to +310 of the _Reg3g_ gene. The pRL-SV40 vector was purchased from Promega. pCMV-STAT6-IRES-Neo was a gift from Axel Nohturfft (Addgene plasmid # 35482).
PROTEIN BINDING ASSAY HEK293 cells obtained from the American Type Culture Collection were transiently transfected with 1 μg of each expression vector using Transfectin Lipid Reagent
(Bio-Rad Laboratories, Hercules, CA). Culture medium was replaced with Opti-MEM (Thermo Scientific) supplemented with 1% fetal calf serum 1 day after transfection, and the cells were
incubated for an additional 48 h. Culture supernatants were then collected and used for protein binding assay. Pneumococci were diluted in assay buffer (Tris buffered saline containing 2 mM
CaCl2) and incubated at 4 °C for 2 h after the addition of 1/5 volume of the HEK293 culture supernatants. Bacterial cells were washed twice with assay buffer, and _S. pneumoniae_-bound
proteins were eluted in SDS-sample buffer. The samples were subjected to Western blot analysis with an anti-Flag Ab. LUCIFERASE ASSAY HEK293 cells grown on 24-well plates were co-transfected
with pRL-SV40 (50 ng) and either of pGL3-Basic, pGL3-_Tff2_ promoter or pGL3-_Reg3g_ promoter (500 ng). The cells were also transfected with pFLAG-CMV2 (300 ng), pFLAG-SPDEF (300 ng), or
pFLAG-ASC (150 ng) plus pFLAG-NLRP3_R258W (150 ng). The cells were washed and lysed in 100 μl of Passive Lysis Buffer (Promega) 48 h after transfection. Firefly luciferase activity and
Renilla luciferase activity in the lysates were measured using the Dual-Luciferase Reporter Assay System (Promega) and Wallac ARVO SX Multilabel Counter (Perkin Elmer, Waltham, MA). pGL3
Firefly luciferase activity was normalized to pRL Renilla luciferase activity. STATISTICAL ANALYSIS For comparisons between two groups, Mann–Whitney _U_ test was used. Multigroup comparisons
were made by Kruskal–Wallis test followed by Dunn’s test. Survival differences were analyzed by the log-rank test. For all statistical analyses, GraphPad Prism 6 software was used.
REFERENCES * Hallstrand, T. S. et al. Airway epithelial regulation of pulmonary immune homeostasis and inflammation. _Clin. Immunol._ 151, 1–15 (2014). Article CAS Google Scholar *
Tuomanen, E. I., Austrian, R. & Masure, H. R. Pathogenesis of pneumococcal infection. _N. Engl. J. Med._ 332, 1280–1284 (1995). Article CAS Google Scholar * Kadioglu, A., Weiser, J.
N., Paton, J. C. & Andrew, P. W. The role of _Streptococcus pneumoniae_ virulence factors in host respiratory colonization and disease. _Nat. Rev. Microbiol._ 6, 288–301 (2008). Article
CAS Google Scholar * Marriott, H. M., Mitchell, T. J. & Dockrell, D. H. Pneumolysin: a double-edged sword during the host-pathogen interaction. _Curr. Mol. Med._ 8, 497–509 (2008).
Article CAS Google Scholar * Kumar, H., Kawai, T. & Akira, S. Pathogen recognition by the innate immune system. _Int. Rev. Immunol._ 30, 16–34 (2011). Article CAS Google Scholar *
Tsuchiya, K. & Hara, H. The inflammasome and its regulation. _Crit. Rev. Immunol._ 34, 41–80 (2014). Article CAS Google Scholar * Latz, E. The inflammasomes: mechanisms of activation
and function. _Curr. Opin. Immunol._ 22, 28–33 (2010). Article CAS Google Scholar * Koppe, U., Suttorp, N. & Opitz, B. Recognition of _Streptococcus pneumoniae_ by the innate immune
system. _Cell Microbiol._ 14, 460–466 (2012). Article CAS Google Scholar * Fang, R. et al. Critical roles of ASC inflammasomes in caspase-1 activation and host innate resistance to
_Streptococcus pneumoniae_ infection. _J. Immunol._ 187, 4890–4899 (2011). Article CAS Google Scholar * McNeela, E. A. et al. Pneumolysin activates the NLRP3 inflammasome and promotes
proinflammatory cytokines independently of TLR4. _PLoS Pathog._ 6, e1001191 (2010). Article Google Scholar * Shoma, S. et al. Critical involvement of pneumolysin in production of
interleukin-1alpha and caspase-1-dependent cytokines in infection with _Streptococcus pneumoniae_ in vitro: a novel function of pneumolysin in caspase-1 activation. _Infect. Immun._ 76,
1547–1557 (2008). Article CAS Google Scholar * Witzenrath, M. et al. The NLRP3 inflammasome is differentially activated by pneumolysin variants and contributes to host defense in
pneumococcal pneumonia. _J. Immunol._ 187, 434–440 (2011). Article CAS Google Scholar * Van, M. L. et al. ASC and NLRP3 impair host defense during lethal pneumonia caused by serotype 3
_Streptococcus pneumoniae_ in mice. _Eur. J. Immunol._ 48, 66–79 (2018). Article Google Scholar * Kafka, D. et al. Contribution of IL-1 to resistance to _Streptococcus pneumoniae_
infection. _Int. Immunol._ 20, 1139–1146 (2008). Article CAS Google Scholar * Lauw, F. N. et al. IL-18 improves the early antimicrobial host response to pneumococcal pneumonia. _J.
Immunol._ 168, 372–378 (2002). Article CAS Google Scholar * Zwijnenburg, P. J., van der Poll, T., Florquin, S., Roord, J. J. & Van Furth, A. M. IL-1 receptor type 1 gene-deficient
mice demonstrate an impaired host defense against pneumococcal meningitis. _J. Immunol._ 170, 4724–4730 (2003). Article CAS Google Scholar * Cash, H. L., Whitham, C. V., Behrendt, C. L.
& Hooper, L. V. Symbiotic bacteria direct expression of an intestinal bactericidal lectin. _Science_ 313, 1126–1130 (2006). Article CAS Google Scholar * Roy, M. G. et al. Muc5b is
required for airway defence. _Nature_ 505, 412–416 (2014). Article CAS Google Scholar * Shah, A. A. et al. Increased susceptibility to _Yersinia enterocolitica_ Infection of Tff2
deficient mice. _Cell. Physiol. Biochem._ 30, 853–862 (2012). Article CAS Google Scholar * Shin, O. S. et al. LPLUNC1 modulates innate immune responses to Vibrio cholerae. _J. Infect.
Dis._ 204, 1349–1357 (2011). Article CAS Google Scholar * Taupin, D. & Podolsky, D. K. Trefoil factors: initiators of mucosal healing. _Nat. Rev. Mol. Cell Biol._ 4, 721–732 (2003).
Article CAS Google Scholar * Tsuji, S. et al. Capture of heat-killed _Mycobacterium bovis_ bacillus Calmette-Guerin by intelectin-1 deposited on cell surfaces. _Glycobiology_ 19, 518–526
(2009). Article CAS Google Scholar * Van Itallie, C. M. & Anderson, J. M. Claudin interactions in and out of the tight junction. _Tissue Barriers_ 1, e25247 (2013). Article Google
Scholar * Zariwala, M. A., Knowles, M. R. & Omran, H. Genetic defects in ciliary structure and function. _Annu. Rev. Physiol._ 69, 423–450 (2007). Article CAS Google Scholar * Di, Y.
P. Functional roles of SPLUNC1 in the innate immune response against Gram-negative bacteria. _Biochem. Soc. Trans._ 39, 1051–1055 (2011). Article CAS Google Scholar * Lim, J. H. et al.
Tumor suppressor CYLD regulates acute lung injury in lethal _Streptococcus pneumoniae_ infections. _Immunity_ 27, 349–360 (2007). Article CAS Google Scholar * Choi, S. M. et al. Innate
Stat3-mediated induction of the antimicrobial protein Reg3gamma is required for host defense against MRSA pneumonia. _J. Exp. Med._ 210, 551–561 (2013). Article CAS Google Scholar *
Wills-Karp, M. et al. Trefoil factor 2 rapidly induces interleukin 33 to promote type 2 immunity during allergic asthma and hookworm infection. _J. Exp. Med._ 209, 607–622 (2012). Article
CAS Google Scholar * Xu, Y., Ikegami, M., Wang, Y., Matsuzaki, Y. & Whitsett, J. A. Gene expression and biological processes influenced by deletion of Stat3 in pulmonary type II
epithelial cells. _BMC Genomics_ 8, 455 (2007). Article Google Scholar * Tu S. P., et al. p53 inhibition of AP1-dependent TFF2 expression induces apoptosis and inhibits cell migration in
gastric cancer cells. _Am J Physiol Gastrointest Liver Physiol_. 297, 385–396 (2009). Article CAS Google Scholar * Thaikoottathil, J. & Chu, H. W. MAPK/AP-1 activation mediates TLR2
agonist-induced SPLUNC1 expression in human lung epithelial cells. _Mol. Immunol._ 49, 415–422 (2011). Article CAS Google Scholar * Hasegawa, M. et al. Mechanism and repertoire of
ASC-mediated gene expression. _J. Immunol._ 182, 7655–7662 (2009). Article CAS Google Scholar * Maeda, Y. et al. Airway epithelial transcription factor NK2 homeobox 1 inhibits mucous cell
metaplasia and Th2 inflammation. _Am. J. Respir. Crit. Care. Med._ 184, 421–429 (2011). Article CAS Google Scholar * Chen, G. et al. SPDEF is required for mouse pulmonary goblet cell
differentiation and regulates a network of genes associated with mucus production. _J. Clin. Invest._ 119, 2914–2924 (2009). Article CAS Google Scholar * Horst, D. et al. Requirement of
the epithelium-specific Ets transcription factor Spdef for mucous gland cell function in the gastric antrum. _J. Biol. Chem._ 285, 35047–35055 (2010). Article CAS Google Scholar * Marko,
C. K. et al. Spdef null mice lack conjunctival goblet cells and provide a model of dry eye. _Am. J. Pathol._ 183, 35–48 (2013). Article CAS Google Scholar * Park, K. S. et al. SPDEF
regulates goblet cell hyperplasia in the airway epithelium. _J. Clin. Invest._ 117, 978–988 (2007). Article CAS Google Scholar * Erle, D. J. & Sheppard, D. The cell biology of asthma.
_J. Cell Biol._ 205, 621–631 (2014). Article CAS Google Scholar * Schmitz, J. et al. IL-33, an interleukin-1-like cytokine that signals via the IL-1 receptor-related protein ST2 and
induces T helper type 2-associated cytokines. _Immunity_ 23, 479–490 (2005). Article CAS Google Scholar * Hebenstreit, D., Luft, P., Schmiedlechner, A., Duschl, A. & Horejs-Hoeck, J.
SOCS-1 and SOCS-3 inhibit IL-4 and IL-13 induced activation of Eotaxin-3/CCL26 gene expression in HEK293 cells. _Mol. Immunol._ 42, 295–303 (2005). Article CAS Google Scholar *
Kostadinova, E. et al. NLRP3 protects alveolar barrier integrity by an inflammasome-independent increase of epithelial cell adherence. _Sci. Rep._ 6, 30943 (2016). Article CAS Google
Scholar * Brandl, K., Plitas, G., Schnabl, B., DeMatteo, R. P. & Pamer, E. G. MyD88-mediated signals induce the bactericidal lectin RegIII gamma and protect mice against intestinal
Listeria monocytogenes infection. _J. Exp. Med._ 204, 1891–1900 (2007). Article CAS Google Scholar * Liu, Y. et al. SPLUNC1/BPIFA1 contributes to pulmonary host defense against
_Klebsiella pneumoniae_ respiratory infection. _Am. J. Pathol._ 182, 1519–1531 (2013). Article CAS Google Scholar * Liu, Y. et al. Increased susceptibility to pulmonary Pseudomonas
infection in Splunc1 knockout mice. _J. Immunol._ 191, 4259–4268 (2013). Article CAS Google Scholar * Vaishnava, S. et al. The antibacterial lectin RegIIIgamma promotes the spatial
segregation of microbiota and host in the intestine. _Science_ 334, 255–258 (2011). Article CAS Google Scholar * Mitchell, C., Provost, K., Niu, N., Homer, R. & Cohn, L. IFN-gamma
acts on the airway epithelium to inhibit local and systemic pathology in allergic airway disease. _J. Immunol._ 187, 3815–3820 (2011). Article CAS Google Scholar * Hara, H. et al.
Phosphorylation of the adaptor ASC acts as a molecular switch that controls the formation of speck-like aggregates and inflammasome activity. _Nat. Immunol._ 14, 1247–1255 (2013). Article
CAS Google Scholar Download references ACKNOWLEDGEMENTS We thank Prof. Jürg Tschopp (University of Lausanne) and the Institute for Arthritis Research for providing _Nlrp3_−/− mice; Prof.
Shun-ichiro Taniguchi (Shinshu University) for _Pycard_−/− mice; Dr. Keisuke Kuida (Millennium Pharmaceuticals) for _Casp1/11_−/− mice; Prof. Shizuo Akira (Osaka University) for _Il1rl1_−/−
mice; the center for anatomical and pathological researches of Kyoto University for histological support; Ms. Shoko Hosojima and Ms. Nana Iwami for technical assistance. This work was
supported by the Japan Society for the Promotion of Science (JSPS KAKENHI Grant Numbers 26460523, 18K07106, 15K15078, 26110002, and 24590522), the Ministry of Health, Labor and Welfare of
Japan, the National Key Research and Development Program of China (2018YFD0500500) and National Natural Science Foundation of China (31400762). AUTHOR INFORMATION Author notes * Ryosuke
Uchiyama Present address: School of Pharmacy and Pharmaceutical Sciences, Mukogawa Women’s University, Nishinomiya, 663–8179, Japan * Shunsuke Sakai Present address: T Lymphocyte Biology
Unit, Laboratory of Parasitic Diseases, National Institute of Allergy and Infectious Diseases, National Institutes of Health, Bethesda, MD, 20892, USA * Hideki Hara Present address:
Department of Pathology and Comprehensive Cancer Center, University of Michigan Medical School, Ann Arbor, MI, 48109, USA * These authors contributed equally to this study: R. Fang, R.
Uchiyama, S. Sakai AUTHORS AND AFFILIATIONS * College of Animal Science and Technology, Southwest University, Chongqing, 400715, China Rendong Fang * Department of Microbiology, Kyoto
University Graduate School of Medicine, Kyoto, 606-8501, Japan Rendong Fang, Shunsuke Sakai, Hideki Hara, Masao Mitsuyama, Ikuo Kawamura & Kohsuke Tsuchiya * Department of Microbiology,
Hyogo College of Medicine, Nishinomiya, 663-8501, Japan Ryosuke Uchiyama & Hiroko Tsutsui * Division of Immunology and Molecular Biology, Cancer Research Institute, Kanazawa University,
Kakuma-Machi, Kanazawa, 920-1192, Japan Takashi Suda & Kohsuke Tsuchiya * Hakubi Center, Kyoto University, Kyoto, 606-8501, Japan Masao Mitsuyama * Institute for Frontier Science
Initiative (InFiniti), Kanazawa University, Kanazawa, 920-1192, Japan Kohsuke Tsuchiya Authors * Rendong Fang View author publications You can also search for this author inPubMed Google
Scholar * Ryosuke Uchiyama View author publications You can also search for this author inPubMed Google Scholar * Shunsuke Sakai View author publications You can also search for this author
inPubMed Google Scholar * Hideki Hara View author publications You can also search for this author inPubMed Google Scholar * Hiroko Tsutsui View author publications You can also search for
this author inPubMed Google Scholar * Takashi Suda View author publications You can also search for this author inPubMed Google Scholar * Masao Mitsuyama View author publications You can
also search for this author inPubMed Google Scholar * Ikuo Kawamura View author publications You can also search for this author inPubMed Google Scholar * Kohsuke Tsuchiya View author
publications You can also search for this author inPubMed Google Scholar CONTRIBUTIONS R.F. performed most of the experiments. R.U. performed the experiments with ST2-deficient mice. S.S.
helped with the microarray analysis. H.H. helped with the bone marrow chimera experiments. H.T. provided materials and reviewed the paper. M.M., I.K., and T.S. discussed the experimental
results and reviewed the paper. K.T. supervised the study, designed the experiments, wrote the paper, and performed experiments. CORRESPONDING AUTHOR Correspondence to Kohsuke Tsuchiya.
ETHICS DECLARATIONS COMPETING INTERESTS The authors declare no competing interests. ADDITIONAL INFORMATION PUBLISHER’S NOTE: Springer Nature remains neutral with regard to jurisdictional
claims in published maps and institutional affiliations. SUPPLEMENTARY INFORMATION SUPPLEMENTARY INFORMATION RIGHTS AND PERMISSIONS OPEN ACCESS This article is licensed under a Creative
Commons Attribution 4.0 International License, which permits use, sharing, adaptation, distribution and reproduction in any medium or format, as long as you give appropriate credit to the
original author(s) and the source, provide a link to the Creative Commons license, and indicate if changes were made. The images or other third party material in this article are included in
the article’s Creative Commons license, unless indicated otherwise in a credit line to the material. If material is not included in the article’s Creative Commons license and your intended
use is not permitted by statutory regulation or exceeds the permitted use, you will need to obtain permission directly from the copyright holder. To view a copy of this license, visit
http://creativecommons.org/licenses/by/4.0/. Reprints and permissions ABOUT THIS ARTICLE CITE THIS ARTICLE Fang, R., Uchiyama, R., Sakai, S. _et al._ ASC and NLRP3 maintain innate immune
homeostasis in the airway through an inflammasome-independent mechanism. _Mucosal Immunol_ 12, 1092–1103 (2019). https://doi.org/10.1038/s41385-019-0181-1 Download citation * Received: 04
July 2018 * Revised: 29 May 2019 * Accepted: 06 June 2019 * Published: 05 July 2019 * Issue Date: September 2019 * DOI: https://doi.org/10.1038/s41385-019-0181-1 SHARE THIS ARTICLE Anyone
you share the following link with will be able to read this content: Get shareable link Sorry, a shareable link is not currently available for this article. Copy to clipboard Provided by the
Springer Nature SharedIt content-sharing initiative