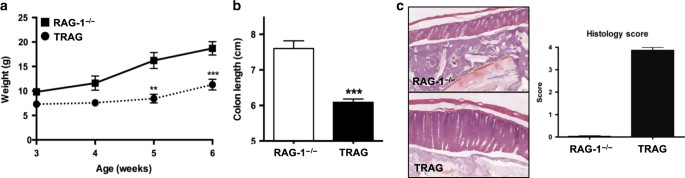
- Select a language for the TTS:
- UK English Female
- UK English Male
- US English Female
- US English Male
- Australian Female
- Australian Male
- Language selected: (auto detect) - EN
Play all audios:
ABSTRACT Innate immunity contributes to the pathogenesis of inflammatory bowel disease (IBD). However, the mechanisms of IBD mediated by innate immunity are incompletely understood and there
are limited models of spontaneous innate immune colitis to address this question. Here we describe a new robust model of colitis occurring in the absence of adaptive immunity.
RAG1-deficient mice expressing TNFAIP3 in intestinal epithelial cells (TRAG mice) spontaneously developed 100% penetrant, early-onset colitis that was limited to the colon and dependent on
intestinal microbes but was not transmissible to co-housed littermates. TRAG colitis was associated with increased mucosal numbers of innate lymphoid cells (ILCs) and depletion of ILC
prevented colitis in TRAG mice. ILC depletion also therapeutically reversed established colitis in TRAG mice. The colitis in TRAG mice was not prevented by interbreeding to mice lacking
group 3 ILC nor by depletion of TNF. Treatment with the JAK inhibitor ruxolitinib ameliorated colitis in TRAG mice. This new model of colitis, with its predictable onset and colon-specific
inflammation, will have direct utility in developing a more complete understanding of innate immune mechanisms that can contribute to colitis and in pre-clinical studies for effects of
therapeutic agents on innate immune-mediated IBD. You have full access to this article via your institution. Download PDF SIMILAR CONTENT BEING VIEWED BY OTHERS CTLA-4 EXPRESSING INNATE
LYMPHOID CELLS MODULATE MUCOSAL HOMEOSTASIS IN A MICROBIOTA DEPENDENT MANNER Article Open access 04 November 2024 HARNESSING MURINE MODELS OF CROHN’S DISEASE ILEITIS TO ADVANCE CONCEPTS OF
PATHOPHYSIOLOGY AND TREATMENT Article 27 July 2021 RATIONALLY DESIGNED BACTERIAL CONSORTIA TO TREAT CHRONIC IMMUNE-MEDIATED COLITIS AND RESTORE INTESTINAL HOMEOSTASIS Article Open access 28
May 2021 INTRODUCTION Ulcerative colitis and Crohn’s disease are chronic debilitating inflammatory disorders of the gastrointestinal tract. The etiology of these inflammatory bowel diseases
(IBDs) is not known but the pathology includes excessive activation of the immune system leading to damage of the intestinal mucosa and loss of normal function. The innate immune system has
a key role in this inflammation, as it is the first to react to microbial challenges, directs the adaptive immune response, and generates the final cellular effectors of immune-mediated
inflammation. In most models of spontaneous genetically driven IBD, adaptive immune cells, in particular T cells, are essential for colitis.1 However, the discovery of a parallel network of
innate lymphoid cells (ILCs) enriched at mucosal sites and capable of producing cytokines comparable to T-helper cells, suggests that the innate immune system alone may be capable of
initiating and sustaining colitis independently from adaptive immune cells.2,3,4 A better understanding of how innate immune-mediated inflammation contributes to IBD is needed in order to
identify promising therapies to treat these diseases. Here we describe a new robust model of spontaneous innate immune mediated colitis that will facilitate a better understanding of innate
immune contributions to IBD. TNFAIP3, also known as A20, is a cytosolic ubiquitin-editing enzyme that inhibits innate immune receptor signaling and tumor necrosis factor (TNF)-induced cell
death.5,6,7,8 TNFAIP3-associated genetic variants have been implicated in human IBD and other inflammatory diseases.8, 9 Mice lacking TNFAIP3 develop inflammation, as they are unable to
properly inhibit microbial activation of innate receptors.5, 10 This is compounded by the fact that TNFAIP3−/− mice also cannot control TNF-induced inflammation and are susceptible to
TNF-induced cell death.7 Cell-type-specific roles for TNFAIP3 in intestinal inflammation have been demonstrated by lineage-specific deletions showing that TNFAIP3 mainly suppresses
inflammation in myeloid cells, while it mainly suppresses cell death in intestinal epithelial cells (IECs).11 Expression of TNFAIP3 in IEC improves barrier function and protects against DSS
colitis and IEC death.12, 13 However, expression of TNFAIP3 in IEC results in invasion of the colonic inner mucus layer by bacteria and increased susceptibility to colitis in IL10−/− mice.14
Thus, the role of TNFAIP3 in intestinal inflammation is both cell and context dependent. Here we describe a new model of IBD wherein immunodeficient RAG1−/− mice that express TNFAIP3 in IEC
spontaneously develop 100% penetrant early-onset colitis. This new model will allow us to better understand the role of innate immunity in colitis and test the effects of established and
emerging therapies for IBD. In this study, we show that ILC depletion can prevent and can also reverse established colitis, suggesting that ILC targeted therapies may be able to maintain and
induce IBD remission, respectively. ILCs act through production of cytokines that can induce responses in target cells through JAK-STAT signals. JAK Inhibitors have shown promise in
clinical trials for IBD and genetic variants associated with JAK2 increase risk for IBD.15, 16 In vitro studies have shown both increased and decreased innate immune inflammation in response
to JAK inhibitors.17,18,19,20 Thus, in vivo models of innate immune inflammation are necessary to help resolve these inconsistencies. In this study we find that ruxolitinib, a JAK1/JAK2
inhibitor, suppresses innate immune-mediated intestinal inflammation. This new robust model of innate immune colitis will provide important insight into innate mechanisms of IBD and allow
testing of therapies for their effects on innate immune inflammation in vivo. RESULTS SPONTANEOUS INNATE IMMUNE-MEDIATED COLITIS We previously demonstrated that villin-TNFAIP3 x IL10−/− mice
develop early-onset 100% penetrant colitis, which was not observed in villin-TNFAIP3 or IL10−/− mice (all on a C57Bl/6 background).14 To investigate the role of regulatory T cells in this
model, we crossed villin-TNFAIP3 mice to immunodeficient RAG1−/− mice with the intention of studying the adoptive T cell transfer model of colitis in these mice. However, villin-TNFAIP3 x
RAG1−/− mice (TRAG mice) spontaneously developed 100% penetrant colitis by the time they were 5–6 weeks of age. This was evident by reduced body weight, shorter colon length and histology
showing extensive colitis in TRAG mice (Fig. 1). This inflammation was not observed in villin-TNFAIP3 or in RAG1−/− mice co-housed as littermates with TRAG mice. Mouse breeding and studies
shown in Fig. 1 were undertaken at the University of Chicago animal care facility but all subsequent work has been performed in the animal care facilities at the University of Notre Dame and
this phenotype has persisted across both institutions. Thus, epithelial expression of TNFAIP3 combined with immunodeficiency results in spontaneous, early onset, 100% penetrant, innate
immune-mediated colitis. INNATE IMMUNE CELLS ARE INCREASED IN TRAG COLITIS To evaluate the immune cells contributing to colitis in TRAG mice, we collected lamina propria leukocytes (LPLs)
from co-housed 4- and 8-week-old TRAG and RAG1−/− littermates. The mucosa of TRAG mice contained increased numbers of CD45+ leukocytes including neutrophils, inflammatory monocytes (Ly6Chi)
and ILCs (Fig. 2). The increase in mucosal innate cells was evident as early as 4 weeks of age, prior to overt clinical signs of colitis in TRAG mice. Thus, colitis in TRAG mice is
characterized by increased numbers of innate immune cells, including ILC, in the colonic mucosa. GENE AND CYTOKINE EXPRESSION IN TRAG MICE In order to understand the nature of colitis in
this model, we analyzed gene expression in the colon, comparing TRAG vs. RAG1−/− mice after the establishment of colitis in TRAG mice. Principle component analysis revealed differential gene
expression in TRAG vs. RAG1−/− mice. RNASeq profiles revealed differential expression of 6663 genes in TRAG vs. RAG1−/− colonic tissue. These included 174 genes with greater than log2fold
change increase and 102 genes with more than log2fold decreased expression in TRAG vs. RAG1−/− colons. Pathway analysis was consistent with interferon-γ (IFNγ), interleukin (IL)-22 and IL17
gene expression signatures, as well as activation of JAK-STAT signaling in TRAG colons, suggestive of cytokine-mediated inflammation (Fig. 3). In addition, increased expression of chemokines
and a signature suggestive of neutrophil and myeloid cell recruitment/migration/chemotaxis was evident in TRAG colons. This is consistent with the increased numbers of neutrophils and
inflammatory myeloid cells we observed in the mucosa of TRAG mice. The increased expression of IFNγ and IL-22, along with genes induced by these cytokines, was confirmed by quantitative PCR
(Fig. 3). To assess cytokine secretion from mucosal immune cells, we cultured LPLs in media and measured cytokine production using a flow cytometric multi-plex bead based assay. Supernatants
of LPLs from TRAG mucosa produced increased amounts of IFNγ, TNF, IL-22, IL-5, IL-1β, and IL-9, compared with those from RAG1−/− mice (Fig. 3). In the absence of adaptive immune cells, the
cytokine profile of the colitic mucosa of TRAG mice is consistent with activation or increased numbers of ILC (IFNγ, TNF, IL-5, IL-9, and IL-22) or other cytokine producing cells such as
inflammatory macrophages (IL-1β). ILC DEPLETION PREVENTS COLITIS IN TRAG MICE The results showing increased numbers of ILC and a cytokine profile consistent with ILC activation in the
intestinal mucosa of TRAG mice suggested that these cells might be contributing to colitis in this model. To test this, we immunodepleted ILC from TRAG mice beginning at 4 weeks of age using
anti-Thy1 antibody (or pre-immune serum as control) injected intraperitoneally (i.p.) every 3 days for at total of 15 days, as previously described.4 TRAG mice treated with control antibody
exhibited clinical and histological signs of colitis comparable to untreated TRAG mice (Fig. 4). Conversely, mice treated with antibody to deplete ILC showed signs of clinical improvement
and markedly reduced histological signs of inflammation (Fig. 4). In addition, LPLs from TRAG mice treated with anti-Thy1 antibody displayed reduced numbers of mucosal ILC, neutrophils and
inflammatory myeloid cells (Fig. 4). Thus, depletion of ILC prevents innate immune mediated colitis in TRAG mice. ILC DEPLETION REVERSES ESTABLISHED COLITIS IN TRAG MICE One goal of IBD
therapy is to induce remission in patients with disease. This can be modeled in mice by allowing colitis to develop before therapeutically treating disease. ILC depletion in young TRAG mice
prevents colitis indicating that ILC are involved in the initiation of innate immune colitis. Whether ILC has a role in maintaining established inflammation in colitis is not known. The
colitis in TRAG mice is accompanied by persistently elevated numbers of ILC, suggesting that ILC may have a role in maintaining this innate immune colitis (Fig. 2). To test this we
therapeutically treated mice with ILC-depleting antibody after the establishment of colitis. Eight-week-old TRAG mice were treated with antibody or control serum for 21 days and assessed for
colitis. Clinical scores suggested that the colitis in TRAG mice was reversed by depletion of ILC over time (Fig. 5). This was supported by LPL preparations showing reduced numbers of
neutrophils and inflammatory myeloid cells in the mucosa of ILC-depleted TRAG mice, compared with control TRAG mice (Fig. 5). Histology also confirmed the reduction of colitis induced by
depleting ILC from mice after the onset of colitis. Thus, ILC depletion can reverse established innate immune colitis to induce remission of disease. TRAG COLITIS IS DISTINCT FROM OTHER
INNATE MODELS OF IBD Innate immune colitis in T-bet−/− × RAG2−/− mice (TRUC) mice is induced by gut microbes and is transmissible to co-housed RAG2−/− or wild-type (WT) mice. We did not
observe any transmission of colitis to co-housed RAG1−/−, v-TNFAIP3, or WT littermates of TRAG mice. Three possible explanations of this are that microbes are not involved in TRAG colitis,
or that the microbes involved in TRAG colitis are not transmissible, or that microbial transmission occurs but does not transfer colitis as seen in TRUC mice. To test whether microbes drive
colitis in TRAG mice, we administered antibiotics to TRAG mice beginning at weaning for three weeks. Antibiotic treatment ameliorated colitis in TRAG mice, indicating that microbes induce
colitis in this model (Fig. 6). Thus, although colitis is induced by microbes in both the TRUC and TRAG models, TRAG colitis is distinct from TRUC colitis in that it is not transmissible to
co-housed mice. Colitis can be induced in RAG−/− mice infected with _Helicobacter hepaticus_. This innate immune colitis model is accompanied by hepatitis. We did not observe hepatitis in
any TRAG mice nor did we observe colitis in any RAG−/− mice in our studies (Fig. 6). Acute and transient innate colitis can also be induced by administration of anti-CD40 antibody to RAG−/−
mice and this is accompanied by systemic inflammation, hepatopathology and splenomegaly. The colitis in TRAG mice was chronic, restricted to the colonic mucosa and accompanied by no signs of
inflammation in the terminal ileum, spleen or liver indicating that this model is distinct from the anti-CD40 innate model of IBD (Fig. 6). Existing models of innate colitis are mediated by
group 3 ILC and are thus ameliorated by interbreeding with mice lacking the transcription factor RoRγt. To test for the role of group 3 ILC in our model we crossed TRAG mice to Rorc−/− mice
and assessed for colitis. TRAG × Rorc−/− mice exhibited colitis with comparable incidence as TRAG littermates (Fig. 6). Histologically, there was some evidence of attenuation of colitis
severity in TRAG x Rorc−/− mice compared to TRAG littermates, indicating that group 3 ILC3 may contribute to colitis severity in TRAG mice, which is consistent with the increased secretion
of IL-22 by TRAG LPLs (Figs. 3, 6). This indicates that the colitis in TRAG mice, unlike other innate models of colitis, can occur independently of RoRγt and is therefore not dependent on
group 3 ILC (or ILC derived from plasticity of group 3 ILC). Colitis in TRUC mice can be ameliorated with anti-TNF antibody treatment. To assess the role of TNF in the TRAG colitis model, we
administered anti-TNF antibody to TRAG mice beginning at weaning for 3 weeks. TRAG mice treated with anti-TNF antibody exhibited colitis comparable to TRAG mice (Fig. 6). Thus, unlike the
TRUC model of colitis, TRAG colitis is not ameliorated by anti-TNF therapy. Together these findings indicate that the innate immune colitis observed in TRAG mice is distinct from other
innate immune models of IBD. JAK INHIBITION PREVENTS INNATE IMMUNE MEDIATED COLITIS ILC respond to and produce cytokines that signal through receptors acting via the JAK/STAT pathway. Our
gene array results were consistent with increased JAK/STAT signal induced gene expression. We therefore assessed STAT activation in Thy1.2+ LPLs isolated from TRAG and RAG1−/− mice. Flow
cytometric staining indicated increased intensity of phospho-STAT1 in the Thy1.2+ LPLs isolated from the mucosa of TRAG mice compared to RAG1−/− littermates, implicating JAK/STAT signaling
in the colitis of TRAG mice (Fig. 7). JAK inhibitors have shown promise in clinical trials for IBD.15 We therefore tested whether JAK inhibition could prevent colitis in TRAG mice.
Four-week-old TRAG mice were administered the JAK2/1 inhibitor ruxolitinib (or dimethyl sulfoxide (DMSO) vehicle control) by gavage twice daily from three to seven weeks of age.
Ruxolitinib-treated mice displayed improved clinical scores for IBD and this was confirmed at necropsy by gross examination of the colon (Fig. 7). LPL preparations displayed reduced innate
immune cell invasion in the mucosa of TRAG mice treated with ruxolitinib. Histology also indicated that treatment with ruxolitinib treatment trended toward reduction of the severity of
colitis in TRAG mice (Fig. 7). The effects of ruxolitinib on colitis were muted, because we observed less colitis than typically seen at this age in the control mice that were treated with
DMSO (vehicle). We suspect that DMSO alone reduced some of the intestinal inflammation in TRAG mice, as DMSO is known to have anti-inflammatory properties in vivo.21 Thus, the innate immune
mediated colitis in this model involves increased activation of STAT signaling in Thy1.2+ cells and can be ameliorated by pharmaceutical inhibition of JAK2/1. DISCUSSION This study is the
first to describe the spontaneous innate immune mediated colitis that occurs in TRAG mice. This model will be invaluable for improving our understanding of innate immune inflammation in
colitis as it is robust, occurring with 100% penetrance with an early and predictable age of onset. One advantage of a predictable and early-onset colitis is that it allows for clinical
studies that model induction and maintenance of remission that are less tractable in other spontaneous colitis models wherein colitis onset is more stochastic and can occur at much later
ages in mice. In the present study, we used this model to demonstrate that depletion of ILC can reverse established colitis and also prevent the establishment of colitis, suggesting that ILC
depletion may be able to both induce and maintain remission of innate immune colitis, respectively. The ability to reverse established colitis by depletion of ILC supports prior studies
showing that therapeutic treatment of _H. hepaticus_ innate colitis with Thy1.2 antibody reversed intestinal inflammation and colitis associated cancer.22 The cytokine IL-22 was identified
as pathogenic in that model and we observed elevated IL-22 secretion from LPLs of TRAG mice. In the _H. hepaticus_ model, pathogenic IL-22 originated from Nkp46− ILC, suggesting that ntural
killer (NK) cells were not driving the chronic stages of colitis in that model.22 One possible source of IL-22 in our model could be group 3 ILC. These cells might be contributing to the
colitis in TRAG mice, but our finding that colitis occurs in TRAG x Rorc−/− mice indicates that group 3 ILC are not required for the development of colitis in TRAG mice. Depletion of Thy1.2+
cells markedly reduced colitis in TRAG mice, suggesting that group 1 or group 2 ILC contribute to the colitis in this model. Group 1 ILC (including ILC1 and NK cells) produce significant
quantities of IFNγ and TNF, which were both found to be increased in the supernatants of cultured LPLs from TRAG mice. Group 1 ILC have been implicated in mouse models of IBD and in human
IBD and may be pathogenic in TRAG colitis.23, 24 Group 2 ILC make Th2-type cytokines and we observed increased levels of both IL-5 and IL-9 in the supernatants of TRAG LPLs. Although Th2
cytokines can drive colitis in mouse models and are implicated in human ulcerative colitis,25,26,27 the function of group 2 ILC in the intestine is considered primarily protective, defending
against helminthes and mediating epithelial cell repair through production of amphiregulin.28, 29 Indeed, we found that levels of amphiregulin were markedly increased in the transcriptome
of TRAG mice, suggesting that group 2 ILC may have been actively working to repair damaged intestinal epithelium in the TRAG colitis model. Thus although we cannot exclude a role for group 2
ILC in this model, it is unlikely that they are acting as inducers of colitis in TRAG mice. Lastly, it is possible that the pathology of TRAG colitis involves more than one subset of ILC
acting together to disrupt intestinal homeostasis. This is suggested by our finding that deletion of RORγt diminished but did not prevent colitis in TRAG mice. Further studies with mice
lacking group 1, group 2, or combinations of groups of ILC will be necessary to resolve this question. This is also the first study to demonstrate the efficacy of a JAK inhibitor in
suppression of innate immune inflammation in the absence of adaptive immunity in vivo. JAK inhibitors are currently being developed or in clinical trials for IBD.15 These inhibitors work by
blocking JAK-STAT signaling, which is a predominant mode of cell signaling by cytokine receptors. One concern about their use is that both pro- and anti-inflammatory cytokines signal through
JAK-STAT pathways.30 Anti-inflammatory cytokines like IL10, and proinflammatory cytokines like IFNγ exert their effects through JAK-STAT mediated suppression or induction of innate immune
inflammation. Therefore it is not clear whether JAK inhibitors will suppress or enhance innate immune mediated inflammation. In vitro studies have been equivocal, with some studies showing
suppression, whereas others show activation, of innate immune inflammation.17,18,19,20 Some of these discrepancies may be due to biphasic dose responses to JAK inhibition.30 In vivo studies
will help to resolve some of these questions and, in the present study, we have shown that one JAK inhibitor, ruxolitinib, has beneficial effects in an innate immune-mediated colitis model.
Thus, this JAK inhibitor suppressed, rather than activated, innate immune inflammation in vivo. De Wilde et al.31 demonstrated that the JAK inhibitor tofacitinib prevented enthesitis caused
by myeloid specific ablation of TNFAIP3 in vivo in an immune competent mouse model, indicating that JAK inhibition is a viable approach to treatment of inflammation initiated by innate
immune cells. The cellular targets of JAK Inhibitors in our model have yet to be determined. We observed increased activation of JAK/STAT signaling in Thy1.2+ cells and amelioration of
colitis in mice depleted of Thy1.2+ cells, suggesting that these could be one target of JAK inhibitors in prevention of inflammation. However, many other cell types, including myeloid cells
and IECs are activated by cytokines that act through JAK/STAT signaling and inhibition of responses to cytokines in these cell types may also be contributing to reduction of inflammation by
ruxolitinib in TRAG mice. It is possible that multiple cytokines acting in a concerted manner bring about the inflammation in TRAG mice and in human IBD. For this reason, the use of JAK
inhibitors, which act on multiple cytokine pathways simultaneously, hold significant promise as IBD therapies. The robust and predictable nature of the colitis in TRAG mice should allow us
to investigate whether ruxolitinib or other JAK inhibitors can also act therapeutically to induce remission in an innate model of colitis. The TRAG colitis is distinct from previously
described models of innate immune-mediated colitis. Mice lacking the transcription factor T-bet (Tbx21−/−) develop spontaneous colitis when crossed to RAG2−/− mice (TRUC mice) on a Balb/C
background.32 The colitis in TRUC mice is transmissible to co-housed immunocompetent littermates, characterized by high levels of TNF and suppressed by anti-TNF therapy.32 Although we found
that microbes drive colitis in TRAG mice, and that LPLs from TRAG mice secreted excess TNF we did not observe transmission of disease or amelioration with anti-TNF therapy. In addition, TRUC
colitis is markedly attenuated on the C57Bl/6 background, whereas TRAG colitis is robust and early onset in this background.33 Lastly, TRUC colitis is not characterized by a high IFNγ
cytokine profile, whereas TRAG colitis includes a high IFNγ profile.32 In addition to spontaneous innate models of inflammation there are induced innate models of colitis. These include
anti-CD40 treatment of RAG1−/− mice, which is accompanied by systemic inflammation and wasting disease, or _H. hepaticus_ infection of immunodeficient mice (i.e., 129SvEvRAG−/−, SCID, or
nude mice), accompanied by hepatitis and typhlitis, which are not seen in TRAG mice.34,35,36,37 We find that TRAG colitis is suppressed by ILC depletion and this is also the case in TRUC
mice, and in induced models of innate colitis.38, 39, 4 Most importantly, we find that TRAG colitis is not prevented by deletion of RoRγt, suggesting that, unlike other innate colitis
models, the colitis in TRAG mice is not dependent on group 3 ILC or cells derived from plasticity of group 3 ILC. Thus, although TRUC colitis or induced models of colitis may share some
immunopathology with TRAG colitis, the colitis in TRAG mice is distinct and likely to provide unique insights into the regulation of intestinal inflammation. As our model is distinct but
shares some features of other innate immune models of IBD it will be of interest to compare these models. For example, regulatory T cells can both prevent and cure colitis in the _H.
hepaticus_ model of innate IBD, which is driven by IL-23 and group 3 ILCs.4, 35, 40, 41 Whether regulatory T cells have this effect on ILC3-independent colitis in TRAG mice, will provide new
insight into possible regulation of inflammation by regulatory T cells. TNFAIP3−/− × RAG1−/− mice develop spontaneous colitis owing to a critical role for TNFAIP3 in suppressing myeloid
cell responses to microbial stimulation.5, 7, 10 This colitis is distinct from TRAG colitis, as TNFAIP3−/− × RAG1−/− mice display widespread systemic inflammation including inflammation in
the liver, lung, skin, spleen and kidneys, which is not seen in TRAG colitis.7 Given the critical anti-inflammatory role of TNFAIP3 it is interesting that transgenic expression of TNFAIP3 in
IEC predisposes to colitis. This likely relates to cell and context dependent roles for TNFAIP3, similar to the cell and context dependent roles of nuclear factor (NF)-κB, a transcription
factor that is negatively regulated by TNFAIP3.5, 7 Activation of NF-κB in innate immune cells is required for inflammation, but loss of NF-κB activation in IEC leads to spontaneous
intestinal inflammation owing to a critical role of NF-κB in protection of IEC from cytokine-induced death.42, 43 Similarly, TNFAIP3, which inhibits NF-κB activation, may have cell context
dependent roles in colitis. TNFAIP3 protects against DSS-induced IEC death,12 but in TRAG colitis ulceration and denudation of the epithelium is occasionally evident, suggestive of IEC death
in this model. Thus TNFAIP3 expression in IEC may increase colitis susceptibility through mechanisms similar to those observed in conditions where NF-κB activation in IEC is blocked, namely
increased epithelial cell susceptibility to cytokine-induced death.42, 43 One explanation for colitis in TRAG mice may be that expression of TNFAIP3 in IEC changes the biogeography of the
intestinal microbiome, thus predisposing to colitis in immunodeficient hosts.14 In normal unchallenged mice, the colonic epithelium is covered by an inner layer of mucus that is relatively
devoid of microbes.44 In villin-TNFAIP3 mice this inner mucus layer is invaded by microbes.14 Despite this, villin-TNFAIP3 mice never spontaneously develop colitis but instead display
increased numbers of mucosal Tregs, which may prevent spontaneous inflammation.14 Indeed, villin-TNFAIP3 x IL10−/− mice exhibit invasion of the inner mucus layer by microbes and develop 100%
penetrant early-onset colitis on the C57Bl/6 background that is not observed in IL10−/− or villin-TNFAIP3 mice.14 Thus, the combination of microbial invasion of the inner mucus layer and
immunodeficiency (IL10 deficiency) results in colitis. It is likely that a similar process of altered microbial biogeography and immunodeficiency results in colitis in TRAG mice. As we have
observed that microbes drive colitis in TRAG mice, it will be of interest to determine how microbes interact with the intestinal mucosa to induce ILC, inflammatory cytokines, and activation
of JAK/STAT signals to promote inflammation. MATERIALS AND METHODS MICE Mice were bred and housed in Freimann Life Sciences Center at the University of Notre Dame. Previously, these mice
were generated, bred and housed at the University of Chicago animal care facility. All experiments were approved by Institutional Animal Care and Use Committees of both institutions. All
mice were bred and maintained on the C57BL/6 background. The villin-TNFAIP3 strain was generated previously using BAC-recombineering of the villin locus and characterized as described.13
RAG1−/− mice (C57Bl/6) were purchased from Jackson Laboratories and interbred to villin-TNFAIP3 mice to generate villin-TNFAIP3 × RAG1−/− (TRAG) mice or RAG1−/− littermate controls. To
generate TRAG × Rorc−/− mice, Rorc+/− mice were purchased from Jackson labs, interbred with RAG1−/− mice, and then crossed to villin-TNFAIP3 mice to generate TRAG × Rorc−/− mice or TRAG
Rorc+/− littermate controls. ASSESSMENT OF COLITIS Mice were monitored either bi-weekly or at each treatment point and disease activity scores were assigned. Disease activity scores were
averaged based on three categories, weight loss, blood in stool, and stool consistency. For weight, no weight loss was scored as 1, weight loss of 1–5% from baseline as 2, 5–10% as 3, 10–15%
as 4, and > 15% as 5. Hemoccult test strips and direct visualization were used to assess blood in stool with no blood scored as 1, blood-positive stool scored as 3, and overt rectal
bleeding scored as 5. Stool consistency was monitored as normal consistency scored as 1, pasty or semiformed stool scored as 3, and liquid stool or diarrhea scored as 5. At necropsy the
colon and cecum were excised and measured for length. Tissues including colon, small intestine, and liver were collected and formalin-fixed and paraffin-embedded tissues were sectioned (4–5
μm) and stained by hematoxylin and eosin for pathological scoring or immunohistochemistry. Histological scores for colitis were assigned by a board certified gastrointestinal pathologist
(C.R.W.) blinded to genotypes and treatments using the criteria: 0 = histologically normal; 1 = intraepithelial neutrophils (mild activity) also associated with karyorectic debris and
proliferation; 2 = crypt abscesses; 3 = > 50 % crypt abscesses; 4 = epithelial erosions or ulcers. LPL EXTRACTION AND FLOW CYTOMETRY Colons along with cecum (without cecal lymphoid patch)
were excised, thoroughly cleared of fecal content and residual fat, and cut longitudinally and into 2 cm sections. The sections were washed with several rounds of PBS to remove the
remaining feces. To eliminate IECs, tissue sections were shaken for ~2 h at 37 °C in RPMI with 1 mM of EDTA. Shaking for an additional ~2 h with collagenase (Sigma) and DNase (Sigma) in RPMI
to isolate the LPLs. Viable nucleated cells were counted and used for flow cytometry analysis. FLOW CYTOMETRY LPL single-cell suspensions, or other leukocytes where indicated, were
incubated with anti-CD16/32 (clone: 93) (eBioscience, San Diego, CA) to reduce nonspecific binding. Cells were incubated on ice for 30 mins in the dark, with a combination of fluorescent
antibodies (eBioscience). A cocktail consisting of anti-CD127 PE (clone: A7R34), anti-CD45.2 PE-eFluor 610 (104), anti-CD3 eFluor 450 (17A2), anti-CD19 eFluor 450 (eBio1D3), anti-NK1.1
eFluor 450 (PK136), anti-Gr-1 eFluor 450 (RB6-8C5), anti-Ter119 eFluor 450 (TER-119), and anti-CD11b eFluor 450 (M1/70), or anti-CD45.2 PerCP-Cy5.5 (104), anti-CD11b PE (M1/70), anti-Gr-1
eFluor 450 (RB6-8C5), anti-Siglec F eFluor 660 (1RNM44N), anti-Ly6C PE-Cy7 (HK1.4). Stained cells were incubated with a fixable viability dye (eBioscience), washed, and subsequently fixed
with 2% paraformaldehyde. Samples were acquired using a BD LSRFortessa X-20 (BD Biosciences, San Jose, CA) and analyzed on FlowJo v10 software (Tree Star, Inc., Ashland, OR). RNA ISOLATION
AND ANALYSIS Immediately after colon extraction, a small section of the distal colon ~2 mm was snap frozen, homogenized with Trizol (Life Technologies), isolated with chloroform,
isopropanol, and ethanol, and suspended in RNase-free water as per manufacturer’s protocol. For total transcriptome analysis, RNA samples were analyzed with the Illumina NextSeq500 with
libraries constructed using the Illumina TruSeq Standard mRNA kit. Overall, 98.5% of the reads passed the quality filters and trimming, which incorporated quality filtering (q20) and
allowing a minimum read length of 30 bases. Cleaned reads were than mapped to Mouse genome (Mus musculus ver. GRCm38.75) using Tophat ver. 2.1.0. Differential Expression analysis was
conducted using Deseq2. Sequence expressions were normalized and Wald’s _χ_2-tests were conducted on each gene to compare TRAG vs. RAG1−/− gene total colonic tissue gene expression.
Ingenuity Pathway Analysis software was utilized for genes expression profiles and pathways analysis. For reverse transcription-PCR (RT-PCR), RNA was isolated from the distal colon using
Trizol (Life Technologies) and cDNA was generated using High Capacity cDNA Reverse Transcription Kits (Applied Biosystems) following manufacturer’s instructions. Quantitative PCR was
performed with SYBR Green (Qiagen) in duplicate with two control housekeeping genes, 18S, and actin. The primer pairs (Integrated DNA Technologies) used for gene amplification were as
follows: IFN-□ fwr: 5′-CCATCAGCAACAACATAAGC-3′, rev: 5′-AGCTCATTGAATGCTTGGCG-3′; IL-22 fwr: 5′-CGCTGCCCGTCAACACCCGG-3′, rev: 5′-CTGATCCTTAGCACTGACTCCTCG-3′; IL-17f fwr:
5′-AACCAGGGCATTTCTGTCCC-3′, rev: 5′-TTTCTTGCTGAATGGCGACG-3′. Amplicon intensity was captured by Applied Biosystems StepOne Plus RT-PCR machine. CT values were normalized using the
housekeeping genes and to compare gene expression in TRAG vs. RAG1−/− colon tissue. CYTOKINE SECRETION FROM LPLS Freshly isolated LPLs (106) isolated from intestinal mucosa were incubated
overnight (18 h) in complete RPMI with 10% fetal calf serum without additional stimulation. Supernatants were assessed for cytokine levels with the Biolegend LEGENDplex cytokine bead array
following manufacturers instructions (Biolegend, San Diego CA). Cytokines assayed in this array included: IL-23, IL-5, IFNγ, TNF, IL-12 (p70), IL-4, IL-1β, GM-CSF, IL-9, IL-17F, IL-17A,
IL-22, and IL-13. ANTIBODY-BASED DEPLETIONS IN VIVO INNATE LYMPHOID CELLS Anti-Thy1.2 (IgG2b) antibody was generated from rat hybridoma cells (ATCC 30-H12). The hybridoma cells were grown in
RPMI-1640 (ATCC) with 10% fetal bovine serum and passaged at 30–40% confluence every other day. To extract the antibody, cells were grown to high density and switched to serum-free media
for 2 days before the supernatant containing the antibody was collected, filtered, and concentrated using Vivaspin 5000 Protein Concentrator Spin Columns (GE Healthcare Life Sciences).
HiTrap Protein G HP column (GE Healthcare Life Sciences) was then utilized to isolate the IgG antibody. The concentration of anti-Thy1.2 was measured using a rat IgG2 Elisa kit
(eBiosciences). Anti-Thy1.2 antibody was injected (1 mg/mouse; i.p.) every 3 days, with controls receiving pre-immune rat serum. Two different treatment strategies with Thy1.2 were
conducted. The first focused on colitis prevention, mice received the treatment at 4 weeks of age, received 5 doses, and were killed at 6–7 weeks of age. The second treatment strategy was
geared toward colitis treatment, mice at 8 weeks of age with already established inflammation were given eight treatments and killed at 11–12 weeks of age. Mice were monitored and disease
activity scores were administered at each treatment point. TUMOR NECROSIS FACTOR Four-week-old mice were treated with anti-TNF antibody (TN3-19.12) or control serum at a dose of 15ug/g every
7 days for 4 weeks, as described.32 ANTIBIOTIC TREATMENTS Weanlings were maintained on a cocktail of antibiotics dissolved in drinking water ad libitum until 6 weeks of age. The antibiotic
cocktail was comprised of vancomycin (500 mg/L), neomycin sulfate (1 g/L), metronidazole (1 g/L), and ampicillin (1 g/L), and was dissolved in grape Kool-Aid to facilitate consumption.
Control mice received Kool-Aid without antibiotics. INHIBITION OF JAK IN VIVO Mice received Ruxolitinib (AdoQ INB018424) via gavage at 60 mg/kg in DMSO, or vehicle control, twice daily from
3 to 7 weeks of age. Mice were assessed for clinical signs of colitis and assessed at euthanasia as described. STATISTICS Statistical analysis was performed using IBM SPSS and GraphPad Prism
software using analysis of variance (ANOVA) with post hoc tests. For clinical scores data was analyzed by two-way ANOVA with Sidak multiple comparisons test. Mann–Whitney tests or Student’s
_T_-tests were performed for paired data, where indicated. Significance was inferred at _p_ < 0.05. REFERENCES * Strober, W., Fuss, I. J. & Blumberg, R. S. The immunology of mucosal
models of inflammation. _Annu. Rev. Immunol._ 20, 495–549 (2002). Article CAS Google Scholar * Artis, D. & Spits, H. The biology of innate lymphoid cells. _Nature_ 517, 293–301
(2015). Article CAS Google Scholar * Geremia, A. et al. IL-23-responsive innate lymphoid cells are increased in inflammatory bowel disease. _J. Exp. Med._ 208, 1127–33 (2011). Article
CAS Google Scholar * Buonocore, S. et al. Innate lymphoid cells drive interleukin-23-dependent innate intestinal pathology. _Nature_ 464, 1371–5 (2010). Article CAS Google Scholar *
Boone, D. L. et al. The ubiquitin-modifying enzyme A20 is required for termination of Toll-like receptor responses. _Nat. Immunol._ 5, 1052–60 (2004). Article CAS Google Scholar * Wertz,
I. E. et al. De-ubiquitination and ubiquitin ligase domains of A20 downregulate NF-kappaB signalling. _Nature_ 430, 694–9 (2004). Article CAS Google Scholar * Lee, E. G. et al. Failure to
regulate TNF-induced NF-kappaB and cell death responses in A20-deficient mice. _Science_ 289, 2350–4 (2000). Article CAS Google Scholar * Ma, A. & Malynn, B. A. A20: linking a
complex regulator of ubiquitylation to immunity and human disease. _Nat. Rev. Immunol._ 12, 774–85 (2012). Article CAS Google Scholar * Jostins, L. et al. Host-microbe interactions have
shaped the genetic architecture of inflammatory bowel disease. _Nature_ 491, 119–24 (2012). Article CAS Google Scholar * Turer, E. E. et al. Homeostatic MyD88-dependent signals cause
lethal inflamMation in the absence of A20. _J. Exp. Med._ 205, 451–64 (2008). Article CAS Google Scholar * Vereecke, L. et al. A20 controls intestinal homeostasis through cell-specific
activities. _Nat. Commun._ 5, 5103 (2014). Article CAS Google Scholar * Rhee, L. et al. Expression of TNFAIP3 in intestinal epithelial cells protects from DSS- but not TNBS-induced
colitis. _Am. J. Physiol. Gastrointest. Liver Physiol._ 303, G220–227 (2012). Article CAS Google Scholar * Kolodziej, L. E. et al. TNFAIP3 maintains intestinal barrier function and
supports epithelial cell tight junctions. _PLoS ONE_ 6, e26352 (2011). Article CAS Google Scholar * Murphy, S. F. et al. Intestinal epithelial expression of TNFAIP3 results in microbial
invasion of the inner mucus layer and induces colitis in IL10-deficient mice. _Am. J. Physiol. Gastrointest. Liver Physiol._ 307, G871–882 (2014). Article CAS Google Scholar * Neurath, M.
F. Current and emerging therapeutic targets for IBD. _Nat. Rev. Gastroenterol. Hepatol._ 14, 269–78 (2017). Article CAS Google Scholar * Barrett, J. C. et al. Genome-wide association
defines more than 30 distinct susceptibility loci for Crohn’s disease. _Nat. Genet._ 40, 955–62 (2008). Article CAS Google Scholar * Ghoreschi, K. et al. Modulation of innate and adaptive
immune responses by tofacitinib (CP-690,550). _J. Immunol._ 186, 4234–43 (2011). Article CAS Google Scholar * Yarilina, A., Xu, K., Chan, C. & Ivashkiv, L. B. Regulation of
inflammatory responses in tumor necrosis factor-activated and rheumatoid arthritis synovial macrophages by JAK inhibitors. _Arthritis Rheum._ 64, 3856–66 (2012). Article CAS Google Scholar
* Pattison, M. J., Mackenzie, K. F. & Arthur, J. S. Inhibition of JAKs in macrophages increases lipopolysaccharide-induced cytokine production by blocking IL-10-mediated feedback. _J.
Immunol._ 189, 2784–92 (2012). Article CAS Google Scholar * Wang, H. et al. The role of JAK-3 in regulating TLR-mediated inflammatory cytokine production in innate immune cells. _J.
Immunol._ 191, 1164–74 (2013). Article CAS Google Scholar * Santos, N. C., Figueira-Coelho, J., Martins-Silva, J. & Saldanha, C. Multidisciplinary utilization of dimethyl sulfoxide:
pharmacological, cellular, and molecular aspects. _Biochem. Pharmacol._ 65, 1035–41 (2003). Article CAS Google Scholar * Kirchberger, S. et al. Innate lymphoid cells sustain colon cancer
through production of interleukin-22 in a mouse model. _J. Exp. Med._ 210, 917–31 (2013). Article CAS Google Scholar * Bernink, J. H. et al. Human type 1 innate lymphoid cells accumulate
in inflamed mucosal tissues. _Nat. Immunol._ 14, 221–9 (2013). Article CAS Google Scholar * Fuchs, A. et al. Intraepithelial type 1 innate lymphoid cells are a unique subset of IL-12- and
IL-15-responsive IFN-gamma-producing cells. _Immunity_ 38, 769–81 (2013). Article CAS Google Scholar * Fuss, I. J. et al. Disparate CD4 + lamina propria (LP) lymphokine secretion
profiles in inflammatory bowel disease. Crohn’s disease LP cells manifest increased secretion of IFN-gamma, whereas ulcerative colitis LP cells manifest increased secretion of IL-5. _J.
Immunol._ 157, 1261–70 (1996). CAS PubMed Google Scholar * Strober, W. & Fuss, I. J. Proinflammatory cytokines in the pathogenesis of inflammatory bowel diseases. _Gastroenterology_
140, 1756–67 (2011). Article CAS Google Scholar * Rosen, M. J. et al. Mucosal expression of type 2 and type 17 immune response genes distinguishes ulcerative colitis from colon-only
Crohn’s disease in treatment-naive pediatric patients. _Gastroenterology_ 152, 1345–57 e1347 (2017). Article CAS Google Scholar * Monticelli, L. A. et al. IL-33 promotes an innate immune
pathway of intestinal tissue protection dependent on amphiregulin-EGFR interactions. _Proc. Natl Acad. Sci. USA_ 112, 10762–7 (2015). Article CAS Google Scholar * von Moltke, J., Ji, M.,
Liang, H. E. & Locksley, R. M. Tuft-cell-derived IL-25 regulates an intestinal ILC2-epithelial response circuit. _Nature_ 529, 221–5 (2016). Article Google Scholar * Hedl, M., Proctor,
D. D. & Abraham, C. JAK2 disease-risk variants are gain of function and JAK signaling threshold determines innate receptor-induced proinflammatory cytokine secretion in macrophages. _J
Immunol_ 197, 3695–704 (2016). Article CAS Google Scholar * De Wilde, K. et al. A20 inhibition of STAT1 expression in myeloid cells: a novel endogenous regulatory mechanism preventing
development of enthesitis. _Ann. Rheum. Dis._ 76, 585–92 (2017). Article Google Scholar * Garrett, W. S. et al. Communicable ulcerative colitis induced by T-bet deficiency in the innate
immune system. _Cell_ 131, 33–45 (2007). Article CAS Google Scholar * Ermann, J. et al. Severity of innate immune-mediated colitis is controlled by the cytokine deficiency-induced colitis
susceptibility-1 (Cdcs1) locus. _Proc. Natl Acad. Sci. USA_ 108, 7137–41 (2011). Article CAS Google Scholar * Li, X. et al. SCID/NCr mice naturally infected with _Helicobacter hepaticus_
develop progressive hepatitis, proliferative typhlitis, and colitis. _Infect. Immun._ 66, 5477–84 (1998). CAS PubMed PubMed Central Google Scholar * Maloy, K. J. et al. CD4 + CD25 +
T(R) cells suppress innate immune pathology through cytokine-dependent mechanisms. _J. Exp. Med._ 197, 111–9 (2003). Article CAS Google Scholar * Ward, J. M. et al. Inflammatory large
bowel disease in immunodeficient mice naturally infected with _Helicobacter hepaticus_. _Lab. Anim. Sci._ 46, 15–20 (1996). CAS PubMed Google Scholar * Uhlig, H. H. et al. Differential
activity of IL-12 and IL-23 in mucosal and systemic innate immune pathology. _Immunity_ 25, 309–18 (2006). Article CAS Google Scholar * Ermann, J., Staton, T., Glickman, J. N., de Waal
Malefyt, R. & Glimcher, L. H. Nod/Ripk2 signaling in dendritic cells activates IL-17A-secreting innate lymphoid cells and drives colitis in T-bet-/-.Rag2-/- (TRUC) mice. _Proc. Natl
Acad. Sci. USA_ 111, E2559–2566 (2014). Article CAS Google Scholar * Powell, N. et al. The transcription factor T-bet regulates intestinal inflammation mediated by interleukin-7 receptor
+ innate lymphoid cells. _Immunity_ 37, 674–84 (2012). Article CAS Google Scholar * Kullberg, M. C. et al. IL-23 plays a key role in _Helicobacter hepaticus_-induced T cell-dependent
colitis. _J. Exp. Med._ 203, 2485–94 (2006). Article CAS Google Scholar * Maloy, K. J., Antonelli, L. R., Lefevre, M. & Powrie, F. Cure of innate intestinal immune pathology by CD4 +
CD25 + regulatory T cells. _Immunol. Lett._ 97, 189–92 (2005). Article CAS Google Scholar * Zaph, C. et al. Epithelial-cell-intrinsic IKK-beta expression regulates intestinal immune
homeostasis. _Nature_ 446, 552–6 (2007). Article CAS Google Scholar * Nenci, A. et al. Epithelial NEMO links innate immunity to chronic intestinal inflammation. _Nature_ 446, 557–61
(2007). Article CAS Google Scholar * Hansson, G. C. & Johansson, M. E. The inner of the two Muc2 mucin-dependent mucus layers in colon is devoid of bacteria. _Gut Microbes_ 1, 51–54
(2010). Article Google Scholar Download references ACKNOWLEDGEMENTS This work was supported by NIH support from NIAID (AI083375-01), NIDDK (DK42086), and the Crohn’s and Colitis Foundation
of America 0-34493-1362 AUTHOR INFORMATION Author notes * These authors contributed equally: A. M. Overstreet, D. L. LaTorre, L. Abernathy-Close. AUTHORS AND AFFILIATIONS * Department of
Microbiology and Immunology, Indiana University School of Medicine, Indianapolis, IN, USA A. M. Overstreet, L. Abernathy-Close, A. M. Boger & D. L. Boone * Department of Biological
Sciences, University of Notre Dame, South Bend, IN, USA D. L. LaTorre, K. R. Adlaka, A. M. Iverson & D. L. Boone * Department of Medicine, University of Chicago, Chicago, IL, USA S. F.
Murphy, L. Rhee & D. S. Bakke * Department of Pathology, University of Chicago, Chicago, IL, USA C. R. Weber Authors * A. M. Overstreet View author publications You can also search for
this author inPubMed Google Scholar * D. L. LaTorre View author publications You can also search for this author inPubMed Google Scholar * L. Abernathy-Close View author publications You can
also search for this author inPubMed Google Scholar * S. F. Murphy View author publications You can also search for this author inPubMed Google Scholar * L. Rhee View author publications
You can also search for this author inPubMed Google Scholar * A. M. Boger View author publications You can also search for this author inPubMed Google Scholar * K. R. Adlaka View author
publications You can also search for this author inPubMed Google Scholar * A. M. Iverson View author publications You can also search for this author inPubMed Google Scholar * D. S. Bakke
View author publications You can also search for this author inPubMed Google Scholar * C. R. Weber View author publications You can also search for this author inPubMed Google Scholar * D.
L. Boone View author publications You can also search for this author inPubMed Google Scholar CONTRIBUTIONS A.M.O., D.L.T., and L.A.C. designed and performed experiments, analyzed data, and
contributed to writing the manuscript. S.F.M., L.R., A.M.O., A.M.B., K.R.A., A.M.I., and D.S.B. performed experiments and reviewed the manuscript. C.R.W. scored and analyzed data. D.L.B.
conceived the project, designed experiments, and wrote the manuscript. CORRESPONDING AUTHOR Correspondence to D. L. Boone. ETHICS DECLARATIONS CONFLICT OF INTEREST The authors declare no
competing interest. ADDITIONAL INFORMATION PUBLISHER'S NOTE: Springer Nature remains neutral with regard to jurisdictional claims in published maps and institutional affiliations.
RIGHTS AND PERMISSIONS Reprints and permissions ABOUT THIS ARTICLE CITE THIS ARTICLE Overstreet, A.M., LaTorre, D.L., Abernathy-Close, L. _et al._ The JAK inhibitor ruxolitinib reduces
inflammation in an ILC3-independent model of innate immune colitis. _Mucosal Immunol_ 11, 1454–1465 (2018). https://doi.org/10.1038/s41385-018-0051-2 Download citation * Received: 28 July
2017 * Revised: 23 May 2018 * Accepted: 31 May 2018 * Published: 09 July 2018 * Issue Date: September 2018 * DOI: https://doi.org/10.1038/s41385-018-0051-2 SHARE THIS ARTICLE Anyone you
share the following link with will be able to read this content: Get shareable link Sorry, a shareable link is not currently available for this article. Copy to clipboard Provided by the
Springer Nature SharedIt content-sharing initiative