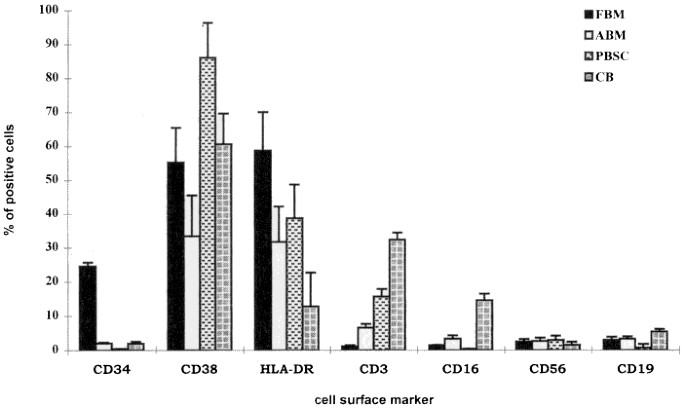
- Select a language for the TTS:
- UK English Female
- UK English Male
- US English Female
- US English Male
- Australian Female
- Australian Male
- Language selected: (auto detect) - EN
Play all audios:
ABSTRACT Hematopoietic stem cell transplantation has been increasingly used to replace a defective hematopoietic system and to treat various genetic defects as well as malignant diseases.
However, the limitations of conventional bone marrow transplantation have stimulated an intense interest in exploring the use of alternative sources of hematopoietic stem cells, including
peripheral blood mononuclear cells (PBMC) and cord blood (CB). A major investigative effort of our laboratory has been focused on evaluating fetal bone marrow (FBM) for transplantation. The
current study compares and characterizes the functional and phenotypic characteristics of FBM, CB, adult bone marrow (ABM), and PBMC by clonogenicity assays, immunogenicity, and the
quantification of progenitor cells. There was a striking difference in the proportion of CD34+ cells in FBM, ABM, PBMC, and CB (24.6%, 2.1%, 0.5%, and 2.0%, respectively). The clonogenic
potential, as measured by colony forming unit in culture (CFU-C) assay, was significantly higher in FBM when compared with ABM, PBMC, and CB (202.5, 73.5, 40.8, and 65.5 colonies/105 cells,
respectively). There was a significant decrease in proliferative responsiveness in mixed lymphocyte reaction (MLR) assay of FBM and CB compared with ABM and PBMC. These observations indicate
that each source of hematopoietic stem cells has different intrinsic properties closely correlated with ontogenetic age that is a vital determinant for phenotypic characteristics, lineage
commitments, immunogenicity, and proliferative potentials. SIMILAR CONTENT BEING VIEWED BY OTHERS IN VITRO EXPANSION OF FETAL LIVER HEMATOPOIETIC STEM CELLS Article Open access 04 June 2021
LINEAGE TRACKING TO REVEAL THE FATE OF HEMATOPOIETIC STEM CELLS INFLUENCED BY FLK2− MULTIPOTENT PROGENITORS AFTER TRANSPLANTATION Article Open access 13 January 2023 NON-CONDITIONED BONE
MARROW CHIMERIC MOUSE GENERATION USING CULTURE-BASED ENRICHMENT OF HEMATOPOIETIC STEM AND PROGENITOR CELLS Article Open access 11 June 2021 MAIN The reduced immunogenicity of fetal tissue
makes it a useful donor source for transplantation. In addition, fetal tissues produce abundant trophic substances and growth factors, which promote cell growth and regeneration of damaged
tissues and cells. The ability for rapid cellular growth, proliferation, differentiation, revascularization, and tissue repair has resulted in the successful use of fetal tissue
transplantation in the treatment of various diseases, including Parkinson's disease, neural tissue injury, diabetes, and myocardial infarction as well as various genetic defects(1–8).
Hematopoietic stem cell transplantation has not only been increasingly used for correction of abnormal hematopoiesis in patients with malignant and nonmalignant diseases but also has made
high dose chemotherapy possible for the treatment of many solid malignancies. Historically, the adult bone marrow has been the major source of hematopoietic stem cells. However, the shortage
of availability of donors for allogeneic bone marrow transplantation and the risk of malignant contamination in autologous bone marrow have stimulated a search for alternative sources of
stem cells. One of the prime sources has been PBMC, which offers several advantages including rapid hematopoietic and immunologic reconstitution and frequently lower risks of contamination
with tumor cells compared with bone marrow grafts(9,10). Similarly, CB is being used as an alternative source and offers the advantages of easy accessibility and a good hematopoietic
cellular yield, which is equivalent to that of ABM(11–14). Although there are variable levels of maternal cell contamination observed in CB that may be associated with GVHD, this has not
posed any major clinical problem(15,16). However, the relatively small number of primitive stem cells and large number of committed progenitors per donor in CB may affect its therapeutic
efficacy. On the other hand, transplantation of fetal tissue in animal models and in human experimental treatments with fetal liver, thymus, spleen, and bone marrow have shown distinct
advantages over adult tissues including lowered immunogenicity and higher percent of primitive cells. Fetus-derived stem cells potentially remove the problems of tissue typing and also have
a high capacity to differentiate into the complete repertoire of erythroid, myeloid, and lymphoid lineages. The present studies were performed to compare the phenotypic characteristics of
FBM, harvested from second trimester of lost pregnancies, with ABM, PBMC, and CB. In addition, the clonogenic potential and immunogenicity of each of these cell sources were evaluated to
determine their suitability for transplantation. METHODS _CELL SOURCES AND PROCESSING._ FBM (_n_ = 8) was individually harvested from fetuses of lost pregnancies in the second trimester (18
to 20 wk gestation). FBM cells were collected from femurs, tibias, vertebral columns, pelvic bones, and scapulae, which were harvested en bloc, dissected aseptically, and cut into small
pieces. After removing the cortex, the marrow-rich cancellous portion was minced with a homogenizer. In all cases, the tissues were collected after informed consent was obtained. Normal ABM
(_n_ = 8) was obtained from normal allogeneic bone marrow donors according to standard techniques developed earlier(17). The volume of each aspiration was limited to < 5 mL to minimize
the contamination of peripheral blood with the harvested marrow(18,19). ABM cells were procured by flushing marrow collection bags and filter screens used in harvesting marrow from normal
allogeneic donors. Recombinant human granulocyte-colony stimulating factor (rhG-CSF) mobilized PBMC (_n_ = 8) were collected from normal volunteer donors. CB (_n_ = 12) was obtained
immediately after delivery by draining samples from the umbilical cord vein into sterile collection tubes containing preservative-free heparin. PBMC and CB were diluted to 1:3 with PBS. The
low-density mononuclear cells were separated by Ficoll-Hypaque (Atlanta Biologicals, GA) density centrifugation and washed twice with PBS. Cell yield and viability were determined by trypan
blue dye exclusion assay. The logistics of human donors were handled according to Department of Health and Human Services/National Institutes of Health guidelines on protection of human
subjects, with the authorization of the Georgetown University Medical School IRB Committee. _PHENOTYPIC ANALYSIS._ Two-color immunofluorescent staining was used to analyze the phenotypic
characteristics of mononuclear cells. Mononuclear cells were resuspended in PBS containing 0.3% BSA, 0.25% human immune globulin, and 0.3% sodium azide at a concentration of 106/100 µL and
incubated for 15 min at 25°C. An aliquot of 5 × 105 cells was removed and stained with monoclonal antibodies directed against various human lymphocyte and/or myeloid antigens. Monoclonal
antibody preparations directed against the following surface markers were used: CD34, CD38, CD3, CD16, CD19, CD56, and HLA-DR (Becton Dickinson, San Jose, CA). Cell analysis was performed on
FACStarplus (Becton Dickinson). The data were analyzed with Reproman and Lysys software. _CLONOGENIC CULTURES._ Mononuclear cells from all sources were added to semisolid media containing
0.8% of methylcellulose (HCC 4230; StemCell Technologies, Inc., Vancouver, Canada) supplemented with erythropoietin (Epo, 2 u/mL) and granulocyte/macrophage colony stimulating factor
(GM-CSF, 20 ng/mL). The cells were plated at a concentration of 1 × 105/mL and for each group, two 1-mL cultures were set up in 35-mm Petri dishes. Plates were incubated at 37°C in a
humidified atmosphere of 5% CO2 for 2 weeks. The number of CFU-C was scored on d 14 with a colony consisting of 40 or more cells. _MIXED LYMPHOCYTE REACTIONS (MLR)._ MLRs were conducted by
culturing 1 × 105 mononuclear cells with equal numbers of irradiated autologous or allogeneic bone marrow or blood cells in 96 well round-bottomed microtiter plates. The stimulator cells
were irradiated with 1500 rads with a JL Shepard Cs-137 Irradiator. All cultures were performed in triplicate in 0.2 mL of RPMI 1640 supplemented with 10% human AB serum. Cultures were
maintained in a 37°C and 5% CO2 humidified incubator for 5 d. DNA synthesis was measured by pulsing with 1 µCi of 3H-thymidine during the final 18 h of the culture. Cultures were harvested
onto glass fiber filter paper using a PHD™ cell harvester (Cambridge Technology Inc., MA) and 3H-thymidine incorporation was determined by liquid scintillation counting. _STATISTICAL
ANALYSIS._ Each of the markers was tested independently using an analysis of variance (ANOVA). In order to achieve normality and equal variance assumptions for the ANOVA, the marker
expression data were transformed using natural logs. Differences in the collection types (FBM, CM, PBMC, and ABM) were tested using Tukey's multiple comparison method(20) to control the
overall significance level at 0.05 for each marker. The colony formation data were tested in a similar manner, but the data were transformed using the square root, which is a standard
transformation instrument for assessment of data to achieve normally distributed values. The overall significance level was 0.01 for the multiple comparison method to control for using the
combined data. Where applicable, simple correlations were performed. RESULTS _PROPORTION OF HEMATOPOIETIC PROGENITORS._ The quantitative yield of mononuclear cells from each single fetus was
approximately 1-3 × 108 and was dependent on the age and the available number of fetal bones. The most striking and unique characteristics of FBM were the number of CD34+ cells in the
mononuclear cells after Ficoll separation. The percentage of CD34+ cells in FBM ranged from 14.8 to 30.7 (mean 24.6 ± 3.5) which was significantly higher when compared with the other three
sources, _i.e._ ABM, PBMC, and CB (Fig. 1). In comparison, the percentages of CD34+ cells in ABM and CB were equivalent, ranging from 1.3-3.4% and 1.2-2.9%, respectively. PBMC had the lowest
number of CD34+ cells (0.2-0.8%). Because the majority of the CD34+ cells are committed to either the hematopoietic or stromal cell lineages, we further compared the most common lineage
associated antigens, CD38 and HLA-DR, expression within the fraction of CD34+ cells. As shown in Table 1 and 2, FBM had the highest number of CD34+/38- and CD34+/HLA-DR- cells (2.11 ± 0.21%
and 3.12 ± 0.19%, respectively). The number of CD34+ cells was statistically analyzed by using ANOVA (Table 2). FBM had the highest log expression (3.13), CB and ABM were better than PBMC,
but were not different from each other (mean log expression: 1.09, 1.11, and 0.376, respectively). For the CD34+/DR- and CD34+/CD38- combination, FBM (1.10) remained the highest. PBMC and
ABM were better than CB, but they were not significantly different from each other. _PHENOTYPIC ANALYSIS OF LYMPHOID POPULATIONS._ In addition to the unique finding of CD34+ cells in FBM,
other phenotypic characteristics were also observed. The percentage of each marker expressed in different sources was shown in Figure 1. For each marker, the type of cell source made a
difference in log expression levels (_p_ < 0.001) for each marker except for CD56+ (_p_ = 0.025). Table 2 shows the mean log expression level for each collection type within each marker.
CD3 was significantly expressed in CB (3.46) and PBMC (2.79), compared with ABM (1.76) and FBM (0.12), but there was no statistical difference between CB and PBMC. Although CD19+ had the
highest expression in CB (1.68), a great percentage was also observed in ABM (0.91). Interestingly, the percentage of CD19+ cells in PBMC (-0.54) was lower than other sources. CD56+ cellular
concentrations in two samples from the ABM collection gave outlier values, causing higher standard deviations, thus potentially violating the normality assumption for the ANOVA. PBMC (1.04)
had the highest CD56 expression but was only significantly higher than CB (0.41). The percentages of CD56+ cells in FBM (0.88) and ABM (0.58) were not significantly different from each
other. CD16 was similarly expressed in both CB and PBMC but was significantly lower in ABM and FBM. _HEMATOPOIETIC PRECURSORS DEFINED BY CLONOGENIC ASSAYS._ Based on our initial finding that
the percentages of CD34+ cells in the four sources were quite different, we further compared the cloning efficiencies of bone marrow and blood cells. The cloning efficiency was defined as
the number of colonies per 1 × 105 cells after a 2-wk culture in semisolid media. Two types of colonies were formed from seeding of the collected cells, _i.e._ CFU-GM and BFU-E, in the
presence of GM-CSF and Epo. The number of CFU-C (CFU-GM + BFU-E)/105 cells from FBM was significantly higher than that from other sources (Fig. 2). The number of colonies from CB was less
than ABM but greater than PBMC. The number of CFU-GM was lower than BFU-E in all cell populations except in CB, where CFU-GM was greater than BFU-E. Table 3 shows the statistical analysis of
colony enumeration from different sources. It can be seen that although FBM showed, in general, higher numbers of colonies from various hematopoietic progenitors, individual differences
were seen between CFU-GM and BFU-E. Strong correlation was found between CD34+ cells and the number of colonies BFU-E and CFU-C. The correlation coefficients were 0.92 (_p_ = 0.01) and 0.93
(_p_ = 0.004), respectively. However, a weak correlation of 0.57 (_p_ = 0.37) was found between CD34+ cells and CFU-GM. The correlation between CD34+ cells and the number of colonies in the
individual groups [FBM, ABM, PBSC, and CB] were poor. Probably, the small sample size (_n_ = 8) in each group was not suitable for correlation analysis. _MIXED LYMPHOCYTE REACTION ASSAYS._
Mixed lymphocyte reactions in both autologous and allogeneic cell cultures are shown in Figure 3. FBM demonstrated reduced responses to both autologous and allogeneic simulators when
compared with adult cells. Interestingly, the proliferative responses were much lower when irradiated FBM was used as a stimulator to other sources. CB exhibited a profound immunoreactivity
when compared with FBM but it was significantly lower than ABM and PBMC. DISCUSSION Hematopoiesis is the process of formation and development of blood cells that is sustained throughout the
life span of the individual by multi-potential stem cells. The varying sites of human hematopoiesis, which takes place during ontogenesis (yolk sac, spleen, thymus, liver, and bone marrow),
provide new insights for the identification of potential sources of stem cells in both children and adults. Thus, CB, PBMC, and fetal liver have been used in hematopoietic stem cell
transplantation experimentally and clinically(21–26). However, there have been no reports using FBM obtained from spontaneously aborted fetuses as an alternative source of hematopoietic stem
cell for transplantation. The present studies provide additional support for the potential use of this source. Fetal tissue is preimmunocompetent and is characterized by a significantly
reduced capacity to evoke immunogenic responses(27,28). The depressed immunocompetence or immunologic immaturity of the fetus is ontogeny-related. It is a result of the reduced number or
lack of postthymic T-lymphocytes _in utero_, which, when mature, contribute to GVHD and/or from immaturity of antigen-presenting cells. In the course of fetal development, the precursors of
the hematopoietic stem cells arise in the yolk sac, migrate to the liver, and thereafter to the thymus and spleen. Eventually, they locate in bone marrow(2). In the second trimester of
pregnancy (18-22 wk), the bone marrow provides an enriched source of hematopoietic precursors. The number of the progenitor cells at this gestational age increases dramatically because of
the rapid development of the fetus. Recently, there has been an increasing interest in CB as a source of stem cells for transplantation. CB has several distinct advantages: it is easily
accessible and contains large number of cells including immature lymphocytes that are not capable of mediating mature T cell functions associated with GVHD(29). Furthermore, the number of
CD34+ cells in CB is similar or slightly higher than in ABM(30,31). However, because of contamination of CB with maternal blood cells, there is the potential risk of GVHD after CB
transplantation. Recently published registry data(11) from 45 European transplantation centers collected between 1988 and 1996 on CB transplantation (_n_ = 143) revealed that in 78
recipients of CB from related donors the estimated survival at 1 y was 63% in all pediatric patients and in some adults with hematopoietic diseases. GVHD of at least grade II in HLA-matched
recipients occurred at 9%, whereas in HLA-mismatched recipients it was estimated to be 50%, with a 29% survival at 1 y. It was concluded that cytomegalovirus contamination seemed to be the
most important predictor of GVHD. More studies are needed to improve the survival of unrelated recipients(32). The observation that the stem cells exist in the peripheral blood of adults has
led to the exploitation of PBMC as an alternative source for hematopoietic reconstitution. However, because a small number of stem cells circulate in the peripheral blood, the
administration of hematopoietic growth factors and/or chemotherapeutic reagents are often required for mobilizing progenitor cells into the blood stream before harvesting(33,34). The
reported frequencies of CD34+ cells in different sources of stem cells varies between different laboratories and are probably related to differences in methods of detection or analysis
criteria. The number of CD34+ cells in ABM and CB ranged from 0.5-4%(31,35), whereas in PBMC, CD34+ cells comprised < 0.5%. Our results showed a significant increase of CD34+ cells and a
higher clonogenic ability of FBM. Previous studies from other laboratories have shown that CD34+ cells can be divided into at least four subgroups according to CD38 and HLA-DR expression.
Among these subgroups, the CD34+/CD38- immunophenotype is considered to be a highly primitive subpopulation of CD34+ cells(36). It has been previously reported that CD34+ cells from ABM with
low or absent expression of HLA-DR antigen are enriched for primitive blast-cell-containing colonies, high proliferative potential-colony-forming cells, and long term culture initiating
cells(37). However, data derived from CB clearly showed that the HLA-DR+ subpopulation of CD34+ cells is more primitive than the CD34+/HLA-DR- population(38,39). Our studies showed that the
percentage of CD34+/HLA-DR- cells in the FBM was significantly higher than other sources (Table 1) but it was not related to the clonogenic potential. Although the percentage of single CD34+
cells in CB was lower than other sources, its general clonogenic ability (CFU-C) was still comparable to ABM. The only significant difference was that the total colonies from CB were
dominated by CFU-GM. This result indicates that CB demonstrates a trend toward a higher proportion of primitive committed progenitor cells. Moreover, most primitive cells in ABM were found
in the CD34+, CD38-, and HLA-DR- cell populations suggesting that ABM and FBM differ in the HLA-DR and CD38 expression levels. This further suggests that during human development, the
primitive progenitor cells differ in their behavior with respect to the ontogeny of hemopoietic system(40,41). MLR is induced by culturing mononuclear cells (which include T cells, B cells,
NK cells, dendritic cells, and monocytes) from one individual as a responder with mononuclear cells derived from another individual as a stimulator. Cells used as stimulators need to be
treated with irradiation or mitomycin C. Two populations of alloreactive T cells are stimulated during allogeneic MLR. One type of T cells is CD8+, which is major histocompatibility complex
(MHC) class I restricted, the other one is CD4+, which is MHC class II restricted. Meanwhile, B cells, dendritic cells, and monocytes also participate in this reaction by presenting antigens
to T cells and/or producing cytokines. It is difficult to say which type of cells dominates over the other because of the collective function of the responder cells in the reaction. Our
data showed a poor correlation between CD3+ cells and the MLR when the same cells were used as stimulators for different responders. A similar level of MLR was observed in PBSC and ABM,
although CD3+ cells in PBSC were three-fold higher than ABM. On the other hand, CB had the highest percentage of CD3+ cells, but its MLR was much lower than PBSC and ABM. The same phenomenon
was also observed when HLA-DR expression was used as a reference marker. It indicates that a single marker cannot predict the level of MLR. The ontogenetic influence to MLR that includes
functional maturity of the responder cells must be considered. In our comparative studies of MLR assays, the FBM revealed a significantly decreased responsiveness after stimulation with
autologous and allogeneic hematopoietic cells. We assume that this immunoincompetence was related to either the reduced number of CD3+ cells or immaturity of antigen presenting cells in FBM.
Further, FBM as a stimulator caused a decreased proliferation in all sources of hematopoietic cells. Thus, these data support the hypothesis that FBM may not be recognized as foreign to the
same extent as ABM. The reduced immunologic responses may reduce the incidence and severity of GVHD and potentially obviate the problem of tissue type matching. Further, the increased
HLA-DR+ cells in FBM might suggest greater propensity for GVHD. However, it is known that both HLA class I and II are involved in graft _versus_ host reactions but not class II alone(32).
Together with the decreased MLR reactivity of fetal cells, FBM cells might express reduced HLA class I and/or immature class II antigens. CB is also composed of phenotypically and
functionally immature lymphocytes that are not capable of mediating mature T cell functions associated with GVHD(24). Preliminary functional studies using CB lymphocytes confirm that they
are alloreactive but respond less vigorously in mixed lymphocytes culture than corresponding adult lymphocytes(29,41). As seen in this study, CB revealed relatively reduced immunoreactivity
and immunogenicity when compared with adult hematopoietic cells, but both of these properties were much higher in CB than in FBM. The high frequency of CD3+ cells in CB conflicts with its
low immunogenicity, probably because the naive T lymphocytes contribute to this population. The results of the present study are in agreement with the current knowledge on the ontogenetic
differences between various sources of hematopoietic stem cells. Namely, each source of hematopoietic stem cells has different intrinsic properties that are closely correlated with
ontogenetic age; and thus, the phenotypic characteristics of human stem cells, the lineage commitment, the level of reconstitution, and engraftment are a function of ontogenetic
age(24,42,43). Our earlier _in vivo_(44–46) as well as _in vitro_(47) studies of FBM transplantation in allogeneic and xenogeneic systems revealed the high efficacy of fetal tissue derived
from miscarriage for transplantation, which is in agreement with data reported by others(48–51). The results of the present study not only support the potential utility of FBM, derived from
spontaneous abortion, for hematopoietic stem cell transplantation, but also suggest that fetal stem cells may provide an important source of cells for gene therapy. A question may be raised
whether a given fetal donor provides a sufficient number of stem cells for effective transplantation. The low immunoreactivity of the fetal tissue suggests that stem cells from more than one
donor could be pooled together. The feasibility of this approach was suggested by our earlier studies in which mixtures of stem cells were transplanted _in utero_, into nonhuman primate
fetuses(44–46). In conclusion, the data in the present study reveal significant ontogenetic differences between sources of stem cells and suggest that fetal bone marrow derived from viable
miscarriages may be a useful source of transplantable cells. More _in vivo_ studies on the engrafting and immune responses in relevant animal model systems are needed to provide predictive
parameters, such as optimal number of stem cells for successful transplantation in the human. ABBREVIATIONS * BFU-E: burst-forming unit - erythrocyte * CFU-C: colony forming unit in culture
* CFU-GM: colony-forming unit - granulocyte macrophage * FBM: fetal bone marrow * ABM: adult bone marrow * CB: cord blood * GVHD: graft _versus_ host disease * PBSC: peripheral blood stem
cell REFERENCES * Leonard ZI 1995 Developmental biology of hematopoiesis. _Blood_ 86: 2876–2891 Article Google Scholar * Metcalf D, More MAS 1971 Embryonic aspects of hematopoiesis. In:
Neuberger A, Tatum EL (eds) _Frontiers of Biology-Hematopoietic Cells_. North Holland Publishing Co, Amsterdam, pp 172–271 Google Scholar * Michejda M 1988 Utilization of fetal tissue in
transplantation. _Fetal Ther_ 21: 129–134 Google Scholar * Michejda M 1990 CNS repair. In: Harrison M, Golbus M, Filly R (eds) _Unborn Patient, 2nd Ed_. W. Saunders, Philadelphia, pp
565–580 Google Scholar * Breeze RE, Wells TH Jr, Freed CR 1995 Implantation of fetal tissue for the management of Parkinson's disease: a technical note. _Neurosurgery_ 36: 1044–1048
Article CAS PubMed Google Scholar * Leor J, Patterson M, Quinones MJ, Kedes LH, Kloner RA 1996 Transplantation of fetal myocardial tissue into the infarcted myocardium of rat: a
potential method for repair of infarcted myocardium?. _Circulation_ 94( suppl 9): II332–336 CAS PubMed Google Scholar * Beattie GM, Otonkoski T, Lopez AD, Hayek A 1997 Functional
beta-cell mass after transplantation of human fetal pancreatic cells: differentiation or proliferation?. _Diabetes_ 46: 244–248 Article CAS PubMed Google Scholar * Anderson DK, Howland
DR, Reier PJ 1995 Fetal neural grafts and repair of the injured spinal cord. _Brain Pathol_ 5: 451–457 Article CAS PubMed Google Scholar * Talmadge JE, Reed E, Ino K, Kessinger A,
Kuszynski C, Heimann D, Varney M, Jackson J, Vose JM, Bierman PJ 1997 Rapid immunologic reconstitution following transplantation with mobilized peripheral blood stem cells as compared to
bone marrow. _Bone Marrow Transplant_ 19: 161–172 Article CAS PubMed Google Scholar * Koehne G, Zeller W, Stockschlaeder M, Zander AR 1997 Phenotype of lymphocyte subsets after
autologous peripheral blood stem cell transplantation. _Bone Marrow Transplant_ 19: 149–156 Article CAS PubMed Google Scholar * Gluckman E, Rocha V, Boyer-Chammard A, Locatelli F, Arcese
W, Pasquini R, Ortega J, Souillet G, Ferreira E, Laporte JP, Fernandez M, Chastang C 1997 Outcome of cord-blood transplantation from related and unrelated donors. _N Engl J Med_ 337:
373–381 Article CAS PubMed Google Scholar * Gluckman E, Broxmeyer HE, Auerbach A, Douglas GW, Devergie A, Esperou H, Thierry D, Socie G, Lehn P, Cooper S, English D, Kurzberg J, Bard J,
Boye EA 1989 Hematopoietic reconstitution in a patient with Fanconi's anemia by means of umbilical cord blood from HLA-identical sibling. _N Engl J Med_ 321: 1174–1178 Article CAS
PubMed Google Scholar * Broxmeyer HE, Douglas GW, Hangoc G, Cooper S, Bard J, English D, Arny M, Thomas L, Boys EA 1989 Human umbilical cord blood as a potential source of transplantable
hematopoietic stem/progenitor cells. _Proc Natl Acad Sci USA_ 86: 3828–3832 Article CAS PubMed PubMed Central Google Scholar * Thoma SJ, Lamping CP, Ziegler BL 1994 Phenotype analysis
of hematopoietic CD34+ cell populations derived from human umbilical cord blood using flow cytometry and cDNA-polymerase chain reaction. _Blood_ 83: 2103–2014 Article CAS PubMed Google
Scholar * Briz M, Regidor C, Monteagudo D, Somolinos N, Garaulet C, Fores R, Posada M, Fernandez MN 1998 Detection of maternal DNA in umbilical cord blood by polymerase chain reaction
amplification of minisatellite sequences. _Bone Marrow Transplant_ 21: 1097–1099 Article CAS PubMed Google Scholar * Cairo MS, Wagner JE 1997 Placental and/or umbilical cord blood: an
alternative source of hematopoietic stem cells for transplantation. _Blood_ 90: 4665–4678 Article CAS PubMed Google Scholar * Thomas ED, Storb R 1970 Technique for human marrow grafting.
_Blood_ 36: 507–515 Article CAS PubMed Google Scholar * Batinic D, Marusic M, Pavletic Z, Bogdanic V, Uzarevic B, Nemet D, Labar B 1990 Relationship between differing volumes of bone
marrow aspirates and their cellular composition. _Bone Marrow Transplant_ 6: 103–107 CAS PubMed Google Scholar * Holdrinet RSG, van Egmond J, Wessels JMC, Haanen C 1980 A method for
quantification of peripheral blood admixture in bone marrow aspirates. _Exp Hematol_ 8: 103–107 CAS PubMed Google Scholar * Scheffe H 1959 The Analysis of Variance. John Wiley & Sons,
Inc, New York pp 73–77 * Conrad PD, Emerson SG 1998 Ex vivo expansion of hematopoietic cells from umbilical cord blood for clinical transplantation. _J Leukoc Biol_ 64: 147–155 Article CAS
PubMed Google Scholar * Gian VG, Moreb JS, Abdel-Mageed A, Weeks FM, Scornick JC, Winggard JR 1998 Successful salvage using mismatched umbilical cord blood transplant in an adult with
recurrent acute myelogenous leukemia failing autologous peripheral blood progenitor cell transplant: a case history and review. _Bone Marrow Transplant_ 21: 1197–1200 Article CAS PubMed
Google Scholar * Gale RP 1992 Fetal liver transplants. _Bone Marrow Transplant_ 21: 1197–1200 Google Scholar * Landsdorp PM, Dragowska W, Mayani H 1993 Ontogeny-related changes in
proliferative potential of human hematopoietic cells. _J Exp Med_ 178: 787–791 Article Google Scholar * Kurtzberg J, Laughlin M, Graham ML, Smith C, Oslon JF, Halperin EC, Ciocci G,
Carrier C, Stevens CE, Rubinstein P 1996 Placental blood as a source of hematopoietic stem cells for transplantation into unrelated recipients. _N Engl J Med_ 335: 157–166 Article CAS
PubMed Google Scholar * Kessinger A, Armitage JO, Landmark JD, Smith DM, Weisenburger DD 1988 Autologous peripheral hematopoietic stem cell transplantation restores hematopoietic function
following marrow ablative therapy. _Blood_ 71: 723–727 Article CAS PubMed Google Scholar * Cleveland WW, Fogel BJ, Brown WT, Kay HEM 1968 Foetal thymic transplant in a case of
Digeorge's syndrome. _Lancet_ 2: 1211–1214 Article CAS PubMed Google Scholar * Steele RW, Limas C, Thurman GB, Schuellin M, Bauer H, Bellanti JA 1972 Familial thymic aplasia:
attempt reconstitution with fetal thymus in a Millipore diffusion chamber. _N Engl J Med_ 287: 787–791 Article CAS PubMed Google Scholar * Harris DT, Schumacher MJ, Locasio J, Besencon
FJ, Olson GB, De Luca D, Shenker L, Bard J, Boyse EA 1992 Phenotypic and functional immaturity of human umbilical cord blood T lymphocytes. _Proc Natl Acad Sci USA_ 89: 10006–10010 Article
CAS PubMed PubMed Central Google Scholar * Ema H, Suda T, Miura Y, Nakauchi H 1990 Colony formation of clone-sorted human hematopoietic progenitors. _Blood_ 75: 1941–1946 Article CAS
PubMed Google Scholar * Saeland S, Duvert V, Caux C, Pandrau D, Favre C, Vallé A, Durand I, Charbord P, deVries J, Banchereau J 1992 Distribution of surface-membrane molecules on bone
marrow and cord blood CD34+ hematopoietic cells. _Exp Hematol_ 20: 24–33 CAS PubMed Google Scholar * Sasazuki T, Juji T, Morihima Y, Kinukawa N, Kashiwabara H, Inoko H, Yoshida T, Kimura
A, Akaza T, Kamikawaji N, Kodera Y, Takaku F 1998 Effect of matching of cells from an unrelated donor. _N Engl J Med_ 339: 1177–1185 Article CAS PubMed Google Scholar * Kessinger A,
Armitage JO 1991 The evolving role of autologous peripheral stem cell transplantation following high-dose chemotherapy for malignancies. _Blood_ 77: 211–213 Article CAS PubMed Google
Scholar * Bensinger WI, Weaver CH, Appelbaum FR, Rowley S, Demirer T, Sanders J, Storb R, Buckner CD 1991 Transplantation of allogeneic peripheral blood stem cells mobilized by recombinant
human granulocyte colony-stimulating factor. _Blood_ 85: 1655–1658 Article Google Scholar * Simmons PJ, Torok-Storb B 1991 CD34 expression by stromal precursors in normal human adult bone
marrow. _Blood_ 78: 2848–2853 Article CAS PubMed Google Scholar * Srour EF, Brand JE, Briddell RA, Leemhuis T, van Besien K, Hoffman R 1991 Human CD34+ HLA-DR- bone marrow cells contain
progenitor cells capable of self-renewal, multi lineage differentiation, and long term in vitro hematopoiesis. _Blood Cells_ 17: 287–295 CAS PubMed Google Scholar * Denis E, Bender J, Lee
W, Schilling M, Smith S, Unerzagt K, Law P, Burgess J 1994 Harvesting, characterization, and culture of CD34+ cells from human bone marrow, peripheral blood, and cord blood. _Blood Cells_
20: 411–423 Google Scholar * Hao QL, Shah AJ, Thiemann FT, Smogorzewska EM, Crooks GM 1995 A functional comparison of CD34+CD38- cells in cord blood and bone marrow. _Blood_ 86: 3745–3753
Article CAS PubMed Google Scholar * Traycoff CM, Abboud MR, Laver J, Brandt JE, Hoffman R, Law P, Ishizawa L, Srour EF 1994 Evaluation of the in vitro behavior of phenotypically defined
populations of umbilical cord blood hematopoietic progenitor cells. _Exp Hematol_ 22: 215–222 CAS PubMed Google Scholar * Terstappen LW, Huang S 1994 Analysis of bone marrow stem cell.
_Blood Cells_ 20: 45–63 CAS PubMed Google Scholar * Huang S, Terstappen LW 1994 Lymphoid and myeloid differentiation of single human CD34+, HLA-DR+, CD38- hematopoietic stem cells.
_Blood_ 83: 1515–1526 Article CAS PubMed Google Scholar * Clement LT, Vink PE, Bradley GE 1990 Novel immunoregulatory functions of phenotypically distinct subpopulations of CD4+ cells in
the human neonate. _J Immunol_ 145: 102–108 CAS PubMed Google Scholar * Lansdorp PM 1995 Developmental changes in the function of hematopoietic stem cells. _Exp Hematol_ 23: 187–191 CAS
PubMed Google Scholar * Michejda M, Peters SM, Bellanti JA 1992 Xenotransplantation, bone marrow transplantation and stem cell reconstitution. _Transplantation_ 54: 759–762 Article CAS
PubMed Google Scholar * Michejda M, Bacher J, Kuwabara T, Hodgen GD 1981 In utero allogeneic bone marrow transplantation in primates: roentgenographic and histologic observation.
_Transplantation_ 32: 96–100 Article CAS PubMed Google Scholar * Michejda M, Quo V 1996 Quo vadis? Fetal tissue transplantation. _J Hematother_ 5: 185–188 Article CAS PubMed Google
Scholar * Michejda M, Verma UN, Mazumder M, Wu AG 1996 Comparative study of hematopoietic precursors from fetal and adult bone marrow. _Fetal Diagn ther_ 11: 52–61 Article Google Scholar
* Low WC, Eastlund T, Verfaille C 1994 Human fetal tissue from spontaneous abortion as potential sources of donor tissue for cell transplantation therapies. _Transplant Proc_ 26: 3400–3406
PubMed Google Scholar * Tocci A, Menichella G, Peretta G, Mancuso S 1994 Fetal tissue collection from spontaneous abortions: a report from a single center. _Fetal Diagn Ther_ 6: 264–268
Google Scholar * Touraine JL, Royo C, Rocarolo MG 1989 Unmatched stem cells transplantation as a possible alternative to bone marrow transplantation. _Transplant Proc_ 21: 3112–3113 CAS
PubMed Google Scholar * Touraine JL 1993 Transplantation of fetal liver stem cells into patients and into human fetuses with induction of immunologic tolerance. _Transplant Proc_ 25:
1012–1013 CAS PubMed Google Scholar Download references AUTHOR INFORMATION AUTHORS AND AFFILIATIONS * International Center for Interdisciplinary Studies of Immunology, Georgetown
University School of Medicine, Washington, 20007, D.C. Ai Guo Wu, Maria Michejda & Joseph A Bellanti * Department of Pediatrics and Microbiology/Immunology, Georgetown University School
of Medicine, Washington, 20007, D.C. Ai Guo Wu, Maria Michejda & Joseph A Bellanti * Bone Marrow Transplantation Program, Georgetown University School of Medicine, Washington, 20007,
D.C. Ai Guo Wu, Amitabha Mazumder & Kenneth R Meehan * Departments of Laboratory Medicine, Georgetown University School of Medicine, Washington, 20007, D.C. Frederick A Menendez *
Obstetrics/Gynecology, Georgetown University School of Medicine, Washington, 20007, D.C. Jean-Gilles Tchabo * Biostatistics Unit, Lombardi Cancer Center, Georgetown University School of
Medicine, Washington, 20007, D.C. Rebecca Slack * Department of Obstetrics/Gynecology, Hutzel Hospital, Wayne State University, Detroit, 48202, Michigan Mark P Johnson Authors * Ai Guo Wu
View author publications You can also search for this author inPubMed Google Scholar * Maria Michejda View author publications You can also search for this author inPubMed Google Scholar *
Amitabha Mazumder View author publications You can also search for this author inPubMed Google Scholar * Kenneth R Meehan View author publications You can also search for this author
inPubMed Google Scholar * Frederick A Menendez View author publications You can also search for this author inPubMed Google Scholar * Jean-Gilles Tchabo View author publications You can also
search for this author inPubMed Google Scholar * Rebecca Slack View author publications You can also search for this author inPubMed Google Scholar * Mark P Johnson View author publications
You can also search for this author inPubMed Google Scholar * Joseph A Bellanti View author publications You can also search for this author inPubMed Google Scholar RIGHTS AND PERMISSIONS
Reprints and permissions ABOUT THIS ARTICLE CITE THIS ARTICLE Wu, A., Michejda, M., Mazumder, A. _et al._ Analysis and Characterization of Hematopoietic Progenitor Cells from Fetal Bone
Marrow, Adult Bone Marrow, Peripheral Blood, and Cord Blood. _Pediatr Res_ 46, 163–169 (1999). https://doi.org/10.1203/00006450-199908000-00006 Download citation * Received: 27 July 1998 *
Accepted: 26 March 1999 * Issue Date: 01 August 1999 * DOI: https://doi.org/10.1203/00006450-199908000-00006 SHARE THIS ARTICLE Anyone you share the following link with will be able to read
this content: Get shareable link Sorry, a shareable link is not currently available for this article. Copy to clipboard Provided by the Springer Nature SharedIt content-sharing initiative