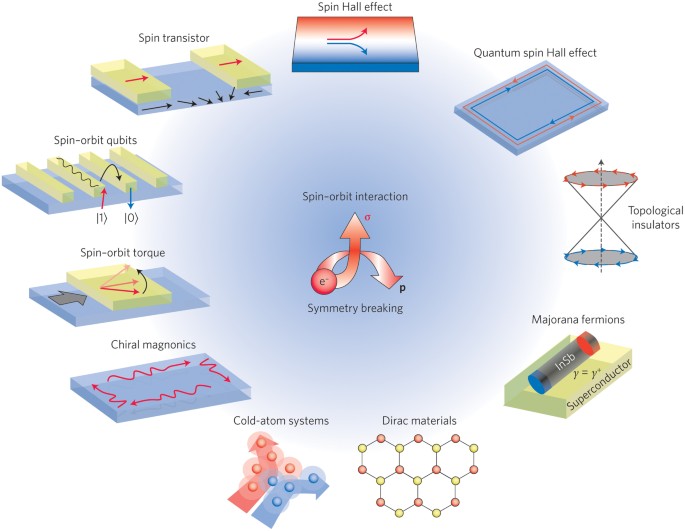
- Select a language for the TTS:
- UK English Female
- UK English Male
- US English Female
- US English Male
- Australian Female
- Australian Male
- Language selected: (auto detect) - EN
Play all audios:
ABSTRACT In 1984, Bychkov and Rashba introduced a simple form of spin–orbit coupling to explain the peculiarities of electron spin resonance in two-dimensional semiconductors. Over the past
30 years, Rashba spin–orbit coupling has inspired a vast number of predictions, discoveries and innovative concepts far beyond semiconductors. The past decade has been particularly creative,
with the realizations of manipulating spin orientation by moving electrons in space, controlling electron trajectories using spin as a steering wheel, and the discovery of new topological
classes of materials. This progress has reinvigorated the interest of physicists and materials scientists in the development of inversion asymmetric structures, ranging from layered
graphene-like materials to cold atoms. This Review discusses relevant recent and ongoing realizations of Rashba physics in condensed matter. Access through your institution Buy or subscribe
This is a preview of subscription content, access via your institution ACCESS OPTIONS Access through your institution Subscribe to this journal Receive 12 print issues and online access
$259.00 per year only $21.58 per issue Learn more Buy this article * Purchase on SpringerLink * Instant access to full article PDF Buy now Prices may be subject to local taxes which are
calculated during checkout ADDITIONAL ACCESS OPTIONS: * Log in * Learn about institutional subscriptions * Read our FAQs * Contact customer support SIMILAR CONTENT BEING VIEWED BY OTHERS
RASHBA-LIKE PHYSICS IN CONDENSED MATTER Article 24 August 2022 SYNTHETIC RASHBA SPIN–ORBIT SYSTEM USING A SILICON METAL-OXIDE SEMICONDUCTOR Article 03 June 2021 FIRST-PRINCIPLES
DETERMINATION OF SPIN–ORBIT COUPLING PARAMETERS IN TWO-DIMENSIONAL MATERIALS Article 25 March 2025 REFERENCES * Dresselhaus, G. Spin-orbit coupling effects in zinc blende structures. _Phys.
Rev._ 100, 580–586 (1955). Article CAS Google Scholar * Rashba, E. Properties of semiconductors with an extremum loop. 1. Cyclotron and combinational resonance in a magnetic field
perpendicular to the plane of the loop. _Sov. Phys. Solid State_ 2, 1109–1122 (1960). Google Scholar * Vas'ko, F. T. Spin splitting in the spectrum of two-dimensional electrons due to
the surface potential. _P. Zh. Eksp. Teor. Fiz._ 30, 574–577 (1979). CAS Google Scholar * Bychkov, Y. A. & Rasbha, E. I. Properties of a 2D electron gas with lifted spectral
degeneracy. _P. Zh. Eksp. Teor. Fiz._ 39, 66–69 (1984). CAS Google Scholar * Fabian, J., Matos-Abiague, A., Ertler, C., Stano, P. & Zutic, I. Semiconductor spintronics. _Acta Phys.
Slovaca_ 57, 565–907 (2007). Article CAS Google Scholar * Chappert, C., Fert, A. & Van Dau, F. N. The emergence of spin electronics in data storage. _Nature Mater._ 6, 813–823 (2007).
Article CAS Google Scholar * Jungwirth, T., Wunderlich, J. & Olejník, K. Spin Hall effect devices. _Nature Mater._ 11, 382–390 (2012). Article CAS Google Scholar * Dyakonov, M. I.
& Perel, V. I. Possibility of orienting electron spins with current. _ZhETF Pis. Red._ 13, 657–660 (1971). Google Scholar * Hirsch, J. Spin Hall effect. _Phys. Rev. Lett._ 83,
1834–1837 (1999). Article CAS Google Scholar * Murakami, S., Nagaosa, N. & Zhang, S.-C. Dissipationless quantum spin current at room temperature. _Science_ 301, 1348–1351 (2003).
Article CAS Google Scholar * Sinova, J. et al. Universal intrinsic spin Hall effect. _Phys. Rev. Lett._ 92, 126603 (2004). Article CAS Google Scholar * Xiao, D., Chang, M.-C. &
Niu, Q. Berry phase effects on electronic properties. _Rev. Mod. Phys._ 82, 1959–2007 (2010). Article CAS Google Scholar * Kato, Y. K., Mährlein, S., Gossard, A. C. & Awschalom, D. D.
Observation of the spin Hall effect in semiconductors. _Science_ 306, 1910–1913 (2004). Article CAS Google Scholar * Wunderlich, J., Kaestner, B., Sinova, J. & Jungwirth, T.
Experimental observation of the spin-Hall effect in a two dimensional spin-orbit coupled semiconductor system. _Phys. Rev. Lett._ 94, 047204 (2005). Article CAS Google Scholar *
Wunderlich, J. et al. Spin Hall effect transistor. _Science_ 330, 1801–1804 (2010). Article CAS Google Scholar * Valenzuela, S. O. & Tinkham, M. Direct electronic measurement of the
spin Hall effect. _Nature_ 442, 176–179 (2006). Article CAS Google Scholar * Hoffmann, A. Spin Hall effects in metals. _IEEE Trans. Magn._ 49, 5172–5193 (2013). Article CAS Google
Scholar * Sinova, J., Valenzuela, S. O., Wunderlich, J., Back, C. H. & Jungwirth, T. Spin Hall effects. _Rev. Mod. Phys._
http://journals.aps.org/rmp/accepted/58077E11Q8f15e01e0aa5510b2767e3da25148b87 (2015). * Rauch, H. et al. Verification of coherent spinor rotation of fermions. _Phys. Lett. A_ 54, 425–427
(1975). Article Google Scholar * König, M. et al. Direct observation of the Aharonov–Casher Phase. _Phys. Rev. Lett._ 96, 076804 (2006). Article CAS Google Scholar * Bergsten, T.,
Kobayashi, T., Sekine, Y. & Nitta, J. Experimental demonstration of the time reversal Aharonov–Casher effect. _Phys. Rev. Lett._ 97, 196803 (2006). Article CAS Google Scholar *
Aharonov, Y. & Casher, A. Topological quantum effects for neutral particles. _Phys. Rev. Lett._ 53, 319–321 (1984). Article CAS Google Scholar * Nitta, J., Akazaki, T., Takayanagi, H.
& Enoki, T. Gate control of spin-orbit interaction in an inverted InGaAs/InAlAs heterostructure. _Phys. Rev. Lett._ 78, 1335–1338 (1997). Article CAS Google Scholar * Wunderlich, J.
et al. Spin-injection Hall effect in a planar photovoltaic cell. _Nature Phys._ 5, 675–681 (2009). Article CAS Google Scholar * Ivchenko, E. L. & Pikus, G. E. New photogalvanic effect
in gyrotropic crystals. _JETP Lett._ 27, 604–608 (1978). Google Scholar * Ganichev, S. D. Spin-galvanic effect and spin orientation by current in non-magnetic semiconductors. _Int. J. Mod.
Phys. B_ 22, 1–26 (2008). Article CAS Google Scholar * Rojas-Sánchez, J. C. et al. Spin-to-charge conversion using Rashba coupling at the interface between non-magnetic materials.
_Nature Commun._ 4, 2944 (2013). Article CAS Google Scholar * Edelstein, V. M. Spin polarization of conduction electrons induced by electric current in two-dimensional asymmetric electron
systems. _Solid State Commun._ 73, 233–235 (1990). Article Google Scholar * Kato, Y. K., Myers, R., Gossard, A. & Awschalom, D. D. Current-induced spin polarization in strained
semiconductors. _Phys. Rev. Lett._ 93, 176601 (2004). Article CAS Google Scholar * Ganichev, S. D. et al. Electric current-induced spin orientation in quantum well structures. _J. Magn.
Magn. Mater._ 300, 127–131 (2006). Article CAS Google Scholar * Schultz, M. et al. Rashba spin splitting in a gated HgTe quantum well. _Semicond. Sci. Technol._ 11, 1168–1172 (1996).
Article CAS Google Scholar * Datta, S. & Das, B. Electronic analog of the electro-optic modulator. _Appl. Phys. Lett._ 56, 665–667 (1990). Article CAS Google Scholar * Koo, H. C.
et al. Control of spin precession in a spin-injected field effect transistor. _Science_ 325, 1515–1518 (2009). Article CAS Google Scholar * Ohe, J., Yamamoto, M., Ohtsuki, T. & Nitta,
J. Mesoscopic Stern–Gerlach spin filter by nonuniform spin-orbit interaction. _Phys. Rev. B_ 72, 041308 (2005). Article CAS Google Scholar * Kohda, M. et al. Spin–orbit induced
electronic spin separation in semiconductor nanostructures. _Nature Commun._ 3, 1082 (2012). Article CAS Google Scholar * Herbert, S. T., Muhammad, M. & Johnson, M. All-electric
quantum point contact spin-polarizer. _Nature Nanotech._ 4, 759–764 (2009). Article CAS Google Scholar * Frolov, S. M. et al. Ballistic spin resonance. _Nature_ 458, 868–871 (2009).
Article CAS Google Scholar * Nowack, K. C., Koppens, F. H. L., Nazarov, Y. V. & Vandersypen, L. M. K. Coherent control of a single electron spin with electric fields. _Science_ 318,
1430–1433 (2007). Article CAS Google Scholar * Nadj-Perge, S., Frolov, S. M., Bakkers, E. P. A. M. & Kouwenhoven, L. P. Spin-orbit qubit in a semiconductor nanowire. _Nature_ 468,
1084–1087 (2010). Article CAS Google Scholar * Van den Berg, J. et al. Fast spin-orbit qubit in an indium antimonide nanowire. _Phys. Rev. Lett._ 110, 066806 (2013). Article CAS Google
Scholar * Petersson, K. D. et al. Circuit quantum electrodynamics with a spin qubit. _Nature_ 490, 380–383 (2012). Article CAS Google Scholar * Dyakonov, M. & Perel, V. Spin
relaxation of conduction electrons in noncentrosymmetric semiconductors. _Sov. Phys. Solid State_ 13, 3023–3026 (1972). Google Scholar * Averkiev, N. S. & Golub, L. E. Giant spin
relaxation anisotropy in zinc-blende heterostructures. _Phys. Rev. B_ 60, 15582–15584 (1999). Article CAS Google Scholar * Schliemann, J., Egues, J. C. & Loss, D. Nonballistic
spin-field-effect transistor. _Phys. Rev. Lett._ 90, 146801 (2003). Article CAS Google Scholar * Bernevig, B. A., Orenstein, J. & Zhang, S.-C. Exact SU(2) symmetry and persistent spin
helix in a spin-orbit coupled system. _Phys. Rev. Lett._ 97, 236601 (2006). Article CAS Google Scholar * Koralek, J. D. et al. Emergence of the persistent spin helix in semiconductor
quantum wells. _Nature_ 458, 610–613 (2009). Article CAS Google Scholar * Sasaki, A. et al. Direct determination of spin–orbit interaction coefficients and realization of the persistent
spin helix symmetry. _Nature Nanotech._ 9, 703–709 (2014). Article CAS Google Scholar * Slonczewski, J. C. Current-driven excitation of magnetic multilayers. _J. Magn. Magn. Mater._ 159,
L1–L7 (1996). Article CAS Google Scholar * Berger, L. Emission of spin waves by a magnetic multilayer traversed by a current. _Phys. Rev. B_ 54, 9353–9358 (1996). Article CAS Google
Scholar * Bernevig, B. A. & Vafek, O. Piezo-magnetoelectric effects in p-doped semiconductors. _Phys. Rev. B_ 72, 033203 (2005). Article CAS Google Scholar * Manchon, A. & Zhang,
S. Theory of nonequilibrium intrinsic spin torque in a single nanomagnet. _Phys. Rev. B_ 78, 212405 (2008). Article CAS Google Scholar * Miron, I. M. et al. Perpendicular switching of a
single ferromagnetic layer induced by in-plane current injection. _Nature_ 476, 189–193 (2011). Article CAS Google Scholar * Chernyshov, A. et al. Evidence for reversible control of
magnetization in a ferromagnetic material by means of spin–orbit magnetic field. _Nature Phys._ 5, 656–659 (2009). Article CAS Google Scholar * Miron, I. M. et al. Current-driven spin
torque induced by the Rashba effect in a ferromagnetic metal layer. _Nature Mater._ 9, 230–234 (2010). Article CAS Google Scholar * Kurebayashi, H. et al. An antidamping spin–orbit torque
originating from the Berry curvature. _Nature Nanotech._ 9, 211–217 (2014). Article CAS Google Scholar * Garello, K. et al. Symmetry and magnitude of spin–orbit torques in ferromagnetic
heterostructures. _Nature Nanotech._ 8, 587–593 (2013). Article CAS Google Scholar * Liu, L. et al. Spin-torque switching with the giant spin Hall effect of tantalum. _Science_ 336,
555–558 (2012). Article CAS Google Scholar * Mellnik, A. R. et al. Spin-transfer torque generated by a topological insulator. _Nature_ 511, 449–451 (2014). Article CAS Google Scholar *
Fan, Y. et al. Magnetization switching through giant spin–orbit torque in a magnetically doped topological insulator heterostructure. _Nature Mater._ 13, 699–704 (2014). Article CAS
Google Scholar * Železný, J. et al. Relativistic Néel-order fields induced by electrical current in antiferromagnets. _Phys. Rev. Lett._ 113, 157201 (2014). Article CAS Google Scholar *
Wadley, P. et al. Electrical switching of an antiferromagnet. Preprint at http://arxiv.org/abs/1503.03765 (2015). * Dzyaloshinskii, I. E. Thermodynamic theory of weak ferromagnetism in
antiferromagnetic substances. _Sov. Phys. JETP_ 5, 1259–1262 (1957). Google Scholar * Moriya, T. Anisotropic superexchange interaction and weak ferromagnetism. _Phys. Rev._ 120, 91–98
(1960). Article CAS Google Scholar * Nagaosa, N. & Tokura, Y. Topological properties and dynamics of magnetic skyrmions. _Nature Nanotech._ 8, 899–911 (2013). Article CAS Google
Scholar * Zakeri, K. et al. Asymmetric spin-wave dispersion on Fe(110): Direct evidence of the Dzyaloshinskii–Moriya interaction. _Phys. Rev. Lett._ 104, 137203 (2010). Article CAS Google
Scholar * Onose, Y. et al. Observation of the magnon Hall effect. _Science_ 329, 297–299 (2010). Article CAS Google Scholar * Matsumoto, R. & Murakami, S. Theoretical prediction of
a rotating magnon wave packet in ferromagnets. _Phys. Rev. Lett._ 106, 197202 (2011). Article CAS Google Scholar * Manchon, A., Ndiaye, P. B., Moon, J., Lee, H. & Lee, K.
Magnon-mediated Dzyaloshinskii–Moriya torque in homogeneous ferromagnets. _Phys. Rev. B_ 90, 224403 (2014). Article CAS Google Scholar * Hasan, M. Z. & Kane, C. L. Colloquium:
Topological insulators. _Rev. Mod. Phys._ 82, 3045–3067 (2010). Article CAS Google Scholar * Kane, C. L. & Mele, E. J. Quantum spin Hall effect in graphene. _Phys. Rev. Lett._ 95,
226801 (2005). Article CAS Google Scholar * Bernevig, B. A., Hughes, T. L. & Zhang, S.-C. Quantum spin Hall effect and topological phase transition in HgTe quantum wells. _Science_
314, 1757–1761 (2006). Article CAS Google Scholar * König, M. et al. Quantum spin Hall insulator state in HgTe quantum wells. _Science_ 318, 766–770 (2007). Article CAS Google Scholar
* Knez, I., Du, R.-R. & Sullivan, G. Evidence for helical edge modes in inverted InAs/GaSb quantum wells. _Phys. Rev. Lett._ 107, 136603 (2011). Article CAS Google Scholar * Hsieh, D.
et al. A tunable topological insulator in the spin helical Dirac transport regime. _Nature_ 460, 1101–1105 (2009). Article CAS Google Scholar * Roushan, P. et al. Topological surface
states protected from backscattering by chiral spin texture. _Nature_ 460, 1106–1109 (2009). Article CAS Google Scholar * Beenakker, C. W. J. Search for Majorana fermions in
superconductors. _Annu. Rev. Condens. Matter Phys._ 4, 113–136 (2013). Article CAS Google Scholar * Fu, L. & Kane, C. Superconducting proximity effect and Majorana fermions at the
surface of a topological insulator. _Phys. Rev. Lett._ 100, 096407 (2008). Article CAS Google Scholar * Středa, P. & Šeba, P. Antisymmetric spin filtering in one-dimensional electron
systems with uniform spin-orbit coupling. _Phys. Rev. Lett._ 90, 256601 (2003). Article CAS Google Scholar * Lutchyn, R., Sau, J. & Das Sarma, S. Majorana fermions and a topological
phase transition in semiconductor-superconductor heterostructures. _Phys. Rev. Lett._ 105, 077001 (2010). Article CAS Google Scholar * Oreg, Y., Refael, G. & von Oppen, F. Helical
liquids and Majorana bound states in quantum wires. _Phys. Rev. Lett._ 105, 177002 (2010). Article CAS Google Scholar * Mourik, V. et al. Signatures of Majorana fermions in hybrid
superconductor-semiconductor nanowire devices. _Science_ 336, 1003–1007 (2012). Article CAS Google Scholar * Nadj-Perge, S. et al. Observation of Majorana fermions in ferromagnetic atomic
chains on a superconductor. _Science_ 346, 602–607 (2014). Article CAS Google Scholar * Choy, T.-P., Edge, J. M., Akhmerov, A. R. & Beenakker, C. W. J. Majorana fermions emerging
from magnetic nanoparticles on a superconductor without spin-orbit coupling. _Phys. Rev. B_ 84, 195442 (2011). Article CAS Google Scholar * Moore, G. & Read, N. Nonabelions in the
fractional quantum Hall effect. _Nucl. Phys. B_ 360, 362–396 (1991). Article Google Scholar * Nayak, C., Simon, S., Stern, A., Freedman, M. & Das Sarma, S. Non-Abelian anyons and
topological quantum computation. _Rev. Mod. Phys._ 80, 1083–1159 (2008). Article CAS Google Scholar * Fu, L. Topological crystalline insulators. _Phys. Rev. Lett._ 106, 106802 (2011).
Article CAS Google Scholar * Alexandradinata, A., Fang, C., Gilbert, M. J. & Bernevig, B. A. Spin-orbit-free topological insulators without time-reversal symmetry. _Phys. Rev. Lett._
113, 116403 (2014). Article CAS Google Scholar * Ikegami, H., Tsutsumi, Y. & Kono, K. Chiral symmetry breaking in 3He-A. _Science_ 341, 59–62 (2013). Article CAS Google Scholar *
Wehling, T. O., Black-Schaffer, A. M. & Balatsky, A. V. Dirac materials. _Adv. Phys._ 63, 1–76 (2014). Article CAS Google Scholar * Volovik, G. E. An analog of the quantum Hall effect
in a superfluid 3He film. _Sov. Phys. JETP_ 67, 1804–1811 (1988). Google Scholar * Katsnelson, M. I., Novoselov, K. S. & Geim, A. K. Chiral tunnelling and the Klein paradox in
graphene. _Nature Phys._ 2, 620–625 (2006). Article CAS Google Scholar * Slonczewski, J. C. & Weiss, P. R. Band structure of graphite. _Phys. Rev._ 109, 272–279 (1958). Article CAS
Google Scholar * Zhang, Y., Tan, Y.-W., Stormer, H. L. & Kim, P. Experimental observation of the quantum Hall effect and Berry's phase in graphene. _Nature_ 438, 201–204 (2005).
Article CAS Google Scholar * Han, W., Kawakami, R. K., Gmitra, M. & Fabian, J. Graphene spintronics. _Nature Nanotech._ 9, 794–807 (2014). Article CAS Google Scholar * Xiao, D.,
Liu, G.-B., Feng, W., Xu, X. & Yao, W. Coupled spin and valley physics in monolayers of MoS2 and other group-VI dichalcogenides. _Phys. Rev. Lett._ 108, 196802 (2012). Article CAS
Google Scholar * Roth, A. et al. Nonlocal transport in the quantum spin Hall state. _Science_ 325, 294–297 (2009). Article CAS Google Scholar * Qiao, Z. et al. Quantum anomalous Hall
effect in graphene proximity coupled to an antiferromagnetic insulator. _Phys. Rev. Lett._ 112, 116404 (2014). Article CAS Google Scholar * Haldane, F. D. M. Model for a quantum Hall
effect without Landau levels: Condensed-matter realization of the 'parity anomaly'. _Phys. Rev. Lett._ 61, 2015–2018 (1988). Article CAS Google Scholar * Balakrishnan, J. et al.
Giant spin Hall effect in graphene grown by chemical vapour deposition. _Nature Commun._ 5, 4748 (2014). Article CAS Google Scholar * Young, A. F. et al. Tunable symmetry breaking and
helical edge transport in a graphene quantum spin Hall state. _Nature_ 505, 528–532 (2014). Article CAS Google Scholar * Marchenko, D. et al. Giant Rashba splitting in graphene due to
hybridization with gold. _Nature Commun._ 3, 1232 (2012). Article CAS Google Scholar * Xiao, D., Yao, W. & Niu, Q. Valley-contrasting physics in graphene: Magnetic moment and
topological transport. _Phys. Rev. Lett._ 99, 236809 (2007). Article CAS Google Scholar * Rycerz, A., Tworzydło, J. & Beenakker, C. W. J. Valley filter and valley valve in graphene.
_Nature Phys._ 3, 172–175 (2007). Article CAS Google Scholar * Hunt, B. et al. Massive Dirac fermions and Hofstadter butterfly in a van der Waals heterostructure. _Science_ 340, 1427–1430
(2013). Article CAS Google Scholar * Abanin, D. A. et al. Giant nonlocality near the Dirac point in graphene. _Science_ 332, 328–330 (2011). Article CAS Google Scholar * Gorbachev, R.
V. et al. Detecting topological currents in graphene superlattices. _Science_ 346, 448–451 (2014). Article CAS Google Scholar * Xu, X., Yao, W., Xiao, D. & Heinz, T. F. Spin and
pseudospins in layered transition metal dichalcogenides. _Nature Phys._ 10, 343–350 (2014). Article CAS Google Scholar * Mak, K. F., He, K., Shan, J. & Heinz, T. F. Control of valley
polarization in monolayer MoS2 by optical helicity. _Nature Nanotech._ 7, 494–498 (2012). Article CAS Google Scholar * Zeng, H., Dai, J., Yao, W., Xiao, D. & Cui, X. Valley
polarization in MoS2 monolayers by optical pumping. _Nature Nanotech._ 7, 490–493 (2012). Article CAS Google Scholar * Mak, K. F., McGill, K. L., Park, J. & McEuen, P. L.
Valleytronics. The valley Hall effect in MoS2 transistors. _Science_ 344, 1489–1492 (2014). Article CAS Google Scholar * Yuan, H. et al. Generation and electric control of
spin-valley-coupled circular photogalvanic current in WSe2 . _Nature Nanotech._ 9, 851–857 (2014). Article CAS Google Scholar * Zhang, Y. J., Oka, T., Suzuki, R., Ye, J. T. & Iwasa,
Y. Electrically switchable chiral light-emitting transistor. _Science_ 344, 725–728 (2014). Article CAS Google Scholar * Wu, S. et al. Electrical tuning of valley magnetic moment through
symmetry control in bilayer MoS2 . _Nature Phys._ 9, 149–153 (2013). Article CAS Google Scholar * Lin, Y.-J., Jimenez-Garcıa, K. & Spielman, I. B. Spin–orbit-coupled Bose–Einstein
condensates. _Nature_ 471, 83–86 (2011). Article CAS Google Scholar * Aidelsburger, M. Experimental realization of strong effective magnetic fields in an optical lattice. _Phys. Rev.
Lett._ 107, 255301 (2011). Article CAS Google Scholar * Zhang, J.-Y. et al. Collective dipole oscillations of a spin-orbit coupled Bose–Einstein condensate. _Phys. Rev. Lett._ 109, 115301
(2012). Article CAS Google Scholar * Qu, C., Hamner, C., Gong, M., Zhang, C. & Engels, P. Observation of zitterbewegung in a spin-orbit-coupled Bose–Einstein condensate. _Phys. Rev.
A_ 88, 021604 (2013). Article CAS Google Scholar * Ji, S.-C. et al. Experimental determination of the finite-temperature phase diagram of a spin–orbit coupled Bose gas. _Nature Phys._ 10,
314–320 (2014). Article CAS Google Scholar * Wang, P., Yu, Z., Fu, Z., Miao, J. & Huang, L. Spin-orbit coupled degenerate Fermi gases. _Phys. Rev. Lett._ 109, 095301 (2012). Article
CAS Google Scholar * Cheuk, L. W. et al. Spin-injection spectroscopy of a spin-orbit coupled Fermi gas. _Phys. Rev. Lett._ 109, 095302 (2012). Article CAS Google Scholar * Jotzu, G.
et al. Experimental realization of the topological Haldane model with ultracold fermions. _Nature_ 515, 237–240 (2014). Article CAS Google Scholar * Williams, R. A. et al. Synthetic
partial waves in ultracold atomic collisions. _Science_ 335, 314–317 (2011). Article CAS Google Scholar * Cole, W. S., Zhang, S., Paramekanti, A. & Trivedi, N. Bose–Hubbard models
with synthetic spin-orbit coupling: Mott insulators, spin textures, and superfluidity. _Phys. Rev. Lett._ 109, 085302 (2012). Article CAS Google Scholar * Radić, J., Di Ciolo, A., Sun, K.
& Galitski, V. Exotic quantum spin models in spin-orbit-coupled Mott insulators. _Phys. Rev. Lett._ 109, 085303 (2012). Article CAS Google Scholar * Cocks, D. et al.
Time-reversal-invariant Hofstadter–Hubbard model with ultracold Fermions. _Phys. Rev. Lett._ 109, 205303 (2012). Article CAS Google Scholar * Cai, Z., Zhou, X. & Wu, C. Magnetic
phases of bosons with synthetic spin-orbit coupling in optical lattices. _Phys. Rev. A_ 85, 061605 (2012). Article CAS Google Scholar * Chen, X., Gu, Z.-C., Liu, Z.-X. & Wen, X.-G.
Symmetry-protected topological orders in interacting bosonic systems. _Science_ 338, 1604–1606 (2012). Article CAS Google Scholar * Vishwanath, A. & Senthil, T. Physics of
three-dimensional bosonic topological insulators: Surface-deconfined criticality and quantized magnetoelectric effect. _Phys. Rev. X_ 3, 011016 (2013). Google Scholar * Hanson, R. &
Awschalom, D. D. Coherent manipulation of single spins in semiconductors. _Nature_ 453, 1043–1049 (2008). Article CAS Google Scholar * Yang, B.-J. & Nagaosa, N. Emergent topological
phenomena in thin films of pyrochlore iridates. _Phys. Rev. Lett._ 112, 246402 (2014). Article CAS Google Scholar * Liu, Z. K. et al. Discovery of a three-dimensional topological Dirac
semimetal, Na3Bi. _Science_ 343, 864–867 (2014). Article CAS Google Scholar * Xu, S. et al. Observation of Fermi arc surface states in a topological metal. _Science_ 347, 294–298 (2015).
Article CAS Google Scholar * Witczak-Krempa, W., Chen, G., Kim, Y. B. & Balents, L. Correlated quantum phenomena in the strong spin-orbit regime. _Annu. Rev. Condens. Matter Phys._ 5,
57–82 (2014). Article CAS Google Scholar * Naaman, R. & Waldeck, D. H. Chiral-induced spin selectivity effect. _J. Phys. Chem. Lett._ 3, 2178–2187 (2012). Article CAS Google
Scholar * Ast, C. et al. Giant spin splitting through surface alloying. _Phys. Rev. Lett._ 98, 186807 (2007). Article CAS Google Scholar * Winkler, R. _Spin-Orbit Coupling effects in
Two-Dimensional Electron and Hole Systems_ (Springer, 2003). Book Google Scholar * Dyakonov, M. I. & Kachorovskii, V. Y. Spin relaxation of two-dimensional electrons in
noncentrosymmetric semiconductors. _Sov. Phys. Semicond._ 20, 110–112 (1986). Google Scholar * Bihlmayer, G., Koroteev, Y. M., Echenique, P. M., Chulkov, E. V. & Blügel, S. The
Rashba-effect at metallic surfaces. _Surf. Sci._ 600, 3888–3891 (2006). Article CAS Google Scholar * Park, Y. H. et al. Separation of Rashba and Dresselhaus spin-orbit interactions using
crystal direction dependent transport measurements. _Appl. Phys. Lett._ 103, 252407 (2013). Article CAS Google Scholar * Nakamura, H., Koga, T. & Kimura, T. Experimental evidence of
cubic Rashba effect in an inversion-symmetric oxide. _Phys. Rev. Lett._ 108, 206601 (2012). Article CAS Google Scholar * LaShell, S., McDougall, B. & Jensen, E. Spin splitting of an
Au(111) surface state band observed with angle resolved photoelectron spectroscopy. _Phys. Rev. Lett._ 77, 3419–3422 (1996). Article CAS Google Scholar * Varykhalov, A. et al. Ir(111)
surface state with giant Rashba splitting persists under graphene in air. _Phys. Rev. Lett._ 108, 066804 (2012). Article CAS Google Scholar * King, P. D. C. et al. Large tunable Rashba
spin splitting of a two-dimensional electron gas in Bi2Se3 . _Phys. Rev. Lett._ 107, 096802 (2011). Article CAS Google Scholar * Ishizaka, K. et al. Giant Rashba-type spin splitting in
bulk BiTeI. _Nature Mater._ 10, 521–526 (2011). Article CAS Google Scholar * Moser, J. et al. Tunneling anisotropic magnetoresistance and spin-orbit coupling in Fe/GaAs/Au tunnel
junctions. _Phys. Rev. Lett._ 99, 056601 (2007). Article CAS Google Scholar * Park, B. G. et al. A spin-valve-like magnetoresistance of an antiferromagnet-based tunnel junction. _Nature
Mater._ 10, 347–351 (2011). Article CAS Google Scholar * Heisenberg, W. _Über den Bau der Atomkerne_. _I. Z. Phys._ 77, 1–11 (1932). Article CAS Google Scholar * Pesin, D. A. &
MacDonald, A. H. Spintronics and pseudospintronics in graphene and topological insulators. _Nature Mater._ 11, 409–416 (2012). Article CAS Google Scholar * Liu, X., Borunda, M. F., Liu,
X. & Sinova, J. Effect of induced spin-orbit coupling for atoms via laser fields. _Phys. Rev. Lett._ 102, 046402 (2009). Article CAS Google Scholar * Dalibard, J., Gerbier, F.,
Juzeliunas, G. & Öhberg, P. Artificial gauge potentials for neutral atoms. _Rev. Mod. Phys._ 83, 1523–1543 (2011). Article CAS Google Scholar Download references ACKNOWLEDGEMENTS The
authors thank E.I. Rashba, M.I. Dyakonov, D. Xiao, L. Fritz and A.H. MacDonald for useful discussions. A.M. was supported by the King Abdullah University of Science and Technology (KAUST).
H.C.K. was supported by the KIST and KU-KIST Institutional Programmes. J.N. acknowledges support by the Grants-in-Aid from the Japan Society for the Promotion of Science (JSPS; no.
22226001). S.M.F. acknowledges ONR BRC on Majorana Fermions, National Science Foundation (NSF), Sloan Foundation, the Charles E. Kaufman foundation and Nanoscience Foundation. R.A.D. is
supported by the Stichting voor Fundamenteel Onderzoek der Materie (FOM), the European Research Council (ERC) and is part of the D-ITP consortium, a programme of the Netherlands Organisation
for Scientific Research (NWO) that is funded by the Dutch Ministry of Education, Culture and Science (OCW). AUTHOR INFORMATION AUTHORS AND AFFILIATIONS * Physical Science and Engineering
Division, King Abdullah University of Science and Technology (KAUST), Thuwal, 23955-6900, Saudi Arabia A. Manchon * Center for Spintronics, Korea Institute of Science and Technology (KIST),
39-1 Hawolgok-dong, Seongbukgu, Seoul, 136-791, Korea H. C. Koo * KU-KIST Graduate School of Converging Science and Technology, Korea University, Seoul, 136-701, Korea H. C. Koo * Department
of Materials Science, Tohoku University, Sendai, 980-8579, Miyagi, Japan J. Nitta * Department of Physics and Astronomy, University of Pittsburgh, Pittsburgh, 15260, Pennsylvania, USA S. M.
Frolov * Institute for Theoretical Physics and Center for Extreme Matter and Emergent Phenomena, Utrecht University, Leuvenlaan 4, Utrecht, 3584 CE, The Netherlands R. A. Duine Authors * A.
Manchon View author publications You can also search for this author inPubMed Google Scholar * H. C. Koo View author publications You can also search for this author inPubMed Google Scholar
* J. Nitta View author publications You can also search for this author inPubMed Google Scholar * S. M. Frolov View author publications You can also search for this author inPubMed Google
Scholar * R. A. Duine View author publications You can also search for this author inPubMed Google Scholar CORRESPONDING AUTHOR Correspondence to A. Manchon. ETHICS DECLARATIONS COMPETING
INTERESTS The authors declare no competing financial interests. RIGHTS AND PERMISSIONS Reprints and permissions ABOUT THIS ARTICLE CITE THIS ARTICLE Manchon, A., Koo, H., Nitta, J. _et al._
New perspectives for Rashba spin–orbit coupling. _Nature Mater_ 14, 871–882 (2015). https://doi.org/10.1038/nmat4360 Download citation * Received: 18 January 2015 * Accepted: 22 June 2015 *
Published: 20 August 2015 * Issue Date: September 2015 * DOI: https://doi.org/10.1038/nmat4360 SHARE THIS ARTICLE Anyone you share the following link with will be able to read this content:
Get shareable link Sorry, a shareable link is not currently available for this article. Copy to clipboard Provided by the Springer Nature SharedIt content-sharing initiative