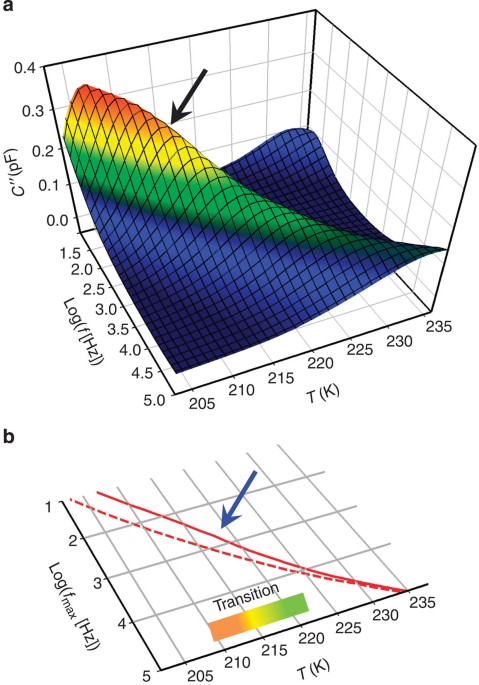
- Select a language for the TTS:
- UK English Female
- UK English Male
- US English Female
- US English Male
- Australian Female
- Australian Male
- Language selected: (auto detect) - EN
Play all audios:
ABSTRACT The nature of the glass transition, the transformation of a liquid into a disordered solid, still remains one of the most intriguing unsolved problems in materials science. Recent
models rationalize crucial features of vitrification with the presence of medium-range ordered regions coexisting with the isotropic liquid. Here, in line with this prediction, we report an
extraordinary enhancement in bond orientational order in ultrathin films of supercooled polyols, grown by physical vapour deposition. By varying the deposition conditions and the molecular
size, we could tune the kinetic stability of the liquid phase enriched in bond orientational order towards conversion into the ordinary liquid phase. We observed a strong increase in the
dielectric strength with respect to the ordinary supercooled liquid and slower structural dynamics, suggesting the existence of a metastable liquid phase with improved orientational
correlations. You have full access to this article via your institution. Download PDF SIMILAR CONTENT BEING VIEWED BY OTHERS REVEALING THE ROLE OF LIQUID PREORDERING IN CRYSTALLISATION OF
SUPERCOOLED LIQUIDS Article Open access 04 August 2022 EMERGENT BIAXIALITY IN CHIRAL HYBRID LIQUID CRYSTALS Article Open access 16 November 2024 ON THE MOLECULAR ORIGINS OF THE FERROELECTRIC
SPLAY NEMATIC PHASE Article Open access 16 August 2021 INTRODUCTION Isotropic liquids are characterized by the absence of any long-range positional order. Operationally, they are
distinguished from solids on the basis of their mechanical response as expressed by the structural relaxation time, _τ_, defined via Maxwell’s relation1 as the ratio between the shear
viscosity _η_ and the instantaneous shear modulus _G_∞. Materials in which _τ_ is shorter than the experimental observation time are considered liquids, whereas in the opposite case, they
are regarded as (disordered) solids. Upon cooling or pressurizing, liquids can transform into the solid state. Depending on their energy landscape and on the thermodynamic and kinetic path
they follow, they acquire a periodic structure with full translational order (crystals) or maintain a morphology lacking in long-range order (glasses)2,3,4,5. The structural relaxation time
increases upon cooling, and the glassy state is achieved when _τ_ exceeds the experimental observation time; upon quenching, this condition occurs at the glass transition temperature, _T_g.
Glasses are thus systems that are out of thermodynamic equilibrium. Although these materials are commonly regarded as disordered systems, crucial features of the glass transition, such as
the increase in heterogeneity and the larger cooperativity of the liquid dynamics approaching solidification6,7,8,9, can be rationalized by the presence of medium-range ordered regions (MRO)
in the liquid phase. Frustration models10,11,12, developed in the past few decades, ascribe this behaviour to the presence of icosahedra, locally preferred structures (LPSs) present in the
isotropic liquid at temperatures below the melting point, _T_m. The formation of these solid-like clusters is driven by maximizing the number of directional bonds that lock the system to
local minima in the free-energy landscape. As a consequence, LPSs are metastable, long-lived structures, showing bond orientational order (BOO) while lacking the long-range topological order
of the crystal. At low pressures, icosahedra are energetically favoured with respect to clusters with crystallographic symmetry, and the resulting geometric frustration prevents the
development of long-range order. In contrast, the two-order parameter model13 predicts that the frustration of crystallization is due to both kinetic and energetic factors: the presence of
an anisotropic potential of intermolecular interaction generates LPSs, whose spatial symmetry is not compatible with the structure of the equilibrium crystal. According both to the paradigm
of the frustration models and to the two-order parameter model, supercooled liquids consist of a heterogeneous mosaic of MRO regions, grown in proximity to LPSs and surrounded by the
isotropic ordinary liquid (OL). Evidence of such structures has been found in metallic glass formers14,15,16, where the presence of quasi-crystalline clusters with a few topological defects
has been detected by diffraction techniques, such as atomic-scale high-resolution transmission electron microscopy and by X-ray, neutron and electron diffraction. An enhanced degree of
topological order may be achieved via structural rearrangements, either by annealing below the glass transition temperature (physical aging)17 or pressurizing18, which permits the nucleation
of MRO regions in proximity to the quasi-crystalline clusters and the consecutive propagation of a similarly ordered structure. For some metallic glasses, this approach has allowed access
to smaller, pre-existing crystalline structures, otherwise undetectable by the aforementioned experimental techniques because the structure factor of an amorphous phase containing
topological order is virtually undistinguishable from the structure factor of a glass where molecules are randomly distributed19. A method used to grow extended ordered regions is physical
vapour deposition (PVD), which has been successfully employed to produce a large family of inorganic misfit layers20 (where the basic periodicities of one or two interpenetrating layers do
not coincide), which are otherwise impossible to obtain. A successive annealing step locks the system into local free-energy minima, dictated by the precursor obtained by PVD. Similarly, PVD
of organic glass formers below _T_g might yield ultradense glasses with highly efficient molecular packing, approaching the limit of the crystal21,22. Those new materials are characterized
by an extremely low excess enthalpy and by a thermodynamic and kinetic stability neither achievable in ordinary glasses, nor by physical aging, hence the name ‘ultrastable glasses’. In
contrast to approaching the glass transition by quenching or pressurizing the liquid, vapour deposition in the glassy state operates in thermodynamic equilibrium, taking advantage of the
enhanced molecular mobility at the free surface23, where evaporated molecules can rapidly sample the energetic landscape and reach deeper minima before being buried by the upcoming layers.
This preparation method thus proves to be, at present, the most efficient way to produce glasses with the lowest thermodynamic excess quantities (enthalpy, volume, entropy)24. In this
report, we present a new method to extend the investigation of medium-range order to organic glass-forming materials by the molecular beam deposition of polyols in the glassy state, followed
by annealing in the liquid state. The severe changes in the dielectric permittivity of samples prepared via our method is consistent with an enrichment of the isotropic liquid in
long-living MRO regions. RESULTS EXPERIMENTAL APPROACH TO ASSESSING HIDDEN ORIENTATIONAL ORDER We exploited the improved sampling of the energy landscape to obtain a liquid phase enriched in
regions with medium-range order, following an approach analogous to that introduced to produce misfit layers. We applied this method to prepare ultrathin films of glycerol, threitol and
xylitol, glass-forming materials known to form, already in the liquid state, temporary H-bonded networks that rely on highly directional, that is, anisotropic, intermolecular interactions25
(_E_OH···O=8–11 _k_B_T_ per bond)26. This feature, in combination with the improved sampling of the energy landscape, enhances the formation of precursor LPSs located at deep minima. The
resulting MRO regions are thus expected to survive long enough to be investigated. The main challenge was the choice of the experimental technique to detect the presence of local order in
organic glass-forming systems. In these materials, in contrast to metallic glasses, no evidence of MRO is seen by scattering and diffraction techniques because of the higher degree of
freedom, which causes a loss of symmetry in the molecular arrangement and thus prevents the generation of any measurable signal. We probed our films instead by dielectric spectroscopy to
gain information about the degree of correlation in the molecular orientation, that is the BOO. After depositing our films in the glassy state, we monitored their dielectric response during
annealing above _T_g, which permitted the measurement of their dielectric strength Δ_ε_, that is, the contribution of the orientational polarization to the dielectric constant (see below).
This quantity is a robust parameter for measuring the BOO, as it is sensitive to the orientation correlation among neighbouring dipoles, via the Kirkwood factor _g_. Indeed,
Δ_ε_≈_g_<_μ_2>/_k_B_T_ (ref. 27), where _μ_ is the molecular dipole moment and _g_ accounts for the correlation among neighbour molecular dipole moments. The value of g is given by
where _z_ is the number of correlated molecules around a given central molecule, and <cos _ψ_> is a statistically averaged cosine of the angle _ψ_ between two neighbouring dipoles.
Thus, if the dipoles have a tendency for parallel orientation, then _g_>1, whereas 0<_g_<1 implies an antiparallel orientation. A random orientation of the dipoles, instead, results
in _g_=1. Accordingly, if an enrichment in MRO regions is finally obtained, the orientational correlation is locally enhanced, leading to an increase or a decrease in the value of _g_,
depending on the spatial organization of the molecules in the local clusters. We anticipate that in the specific case of our investigation, we found a clear enhancement of Δ_ε_ and a
consequent increase in _g_, indicating a parallel orientational correlation among the dipoles, which can be justified by the presence of (locally preferred) anisotropic structures. Moreover,
such an increase rules out the presence of long-range order and crystal-like BOO, which would instead cause a decrease in the dielectric response due to the suppression of the rotational
and translational modes in these clusters. Furthermore, as a consequence of a larger correlation length, the MRO regions are expected to have slower structural dynamics (corresponding to
~2–10 _τ_) with respect to the isotropic liquid28,29. DIELECTRIC RESPONSE OF A LIQUID WITH ENHANCED BOO In glycerol films (~10–130 nm), we found a tremendous enhancement in the dielectric
strength (Fig. 1), exceeding by more than threefold the value measured for bulk glycerol films deposited well above _T_g (ref. 30). Such a large value cannot be rationalized by a mere
increase in density, which would prompt non-physical changes, nor by a larger effective dipole moment, due to a very unlikely perfectly planar orientation in the direction of the electric
field. We ascribe this increase to a higher orientational correlation among the dipole moments, which is consistent with the concept of a liquid state having enhanced MRO and BOO, compared
with the OL. Hereafter, this particular liquid phase will be denoted as medium-range order enriched liquid (MROL). Concurrently, we observed structural relaxation times 4–5-fold longer than
the relaxation time of the OL, corresponding to a shift in _T_g of ~4 K. This finding is in line with the quantitative predictions proposed both for Lennard–Jones liquids28 and for model
polymeric systems29. To quantify the perturbation in the molecular orientation in the MROL, we analysed the temperature evolution of Δ_ε_N=Δ_ε_MROL/Δ_ε_OL. The isothermal dielectric spectra
acquired upon annealing showed that Δ_ε_N begins to decrease at an onset temperature depending on the material, on the thickness of the films and on the temperature of deposition (Figs 2 and
3), which indicates the metastable nature of the ordered structures. We were able to observe the MROL→OL transition via the temperature evolution of Δ_ε_N (Fig. 2, Methods and Supplementary
Fig. S1). The OL behaviour, that is, Δ_ε_N=1, is eventually recovered after prolonged annealing at _T_>_T_g. It must be noted that, compared with Δ_ε_, _τ_ is a less sensitive parameter
to the presence of MRO. The variation of Δ_ε_ with the concentration of MRO regions is remarkably larger with respect to the evolution of _τ_, which allows a better resolution in monitoring
the MROL→OL transition (Supplementary Fig. S2). Similar perturbations to the dielectric function have been observed for natural rubber, following the application of a tensile stress:
although the value of Δ_ε_ tripled, _τ_ remained unaffected by stretching31. In glycerol films (thicker than 20 nm), we observed an enhancement by a factor of 3.7 in Δ_ε_N and a simultaneous
increase of 4.5-fold in the relaxation time; during the MROL→OL transition, these alterations led to a broadening of the relaxation peak in the dielectric loss, without separation between
the contributions from the MRO regions and from the amorphous liquid (Supplementary Figs S1 and S3). For these reasons, we concentrated our investigation mainly on the dielectric strength.
Additional experiments on larger homologous molecules, such as threitol and xylitol, revealed the same trends as for glycerol, but to a lesser extent (smaller Δ_ε_N and lower onset
temperatures, Fig. 2), which further supports the H-bonding interaction as the origin of the enhanced orientational correlation. Indeed, the larger multiplicity of molecular conformations
that arise from the higher intramolecular degrees of freedom with increasing molecular size, likely reduces the efficiency of the formation of an ordered intermolecular network. Such a trend
is in further agreement with the idea that the more fragile glass formers (_m_xylitol>_m_threitol>_m_glycerol, (ref. 32) with _m_ the fragility) are characterized by lower
frustration, that is, by a lower concentration of (locally) ordered structures11,28, which explains the smaller increase in polarization with increasing molecular size. LIFETIME AND LENGTH
SCALE OF THE ENHANCEMENT IN POLARIZATION We gained further insight into the origin of the MRO regions and the related increase in Δ_ε_ of the MROL phase by investigating the dielectric
response of 30-nm-thick glycerol films, grown holding the substrate at different temperatures, _T_sub. After deposition, we measured Δ_ε_N during identical temperature scans, and we analysed
the impact of _T_sub on the maximum value of the increase in dielectric strengths, Δ_ε_Nmax, as observed before the onset of the transition to the OL (Fig. 3, red circles). To relate this
parameter to the kinetic stability of the MROL phase, we compared its temperature dependence with the temperature dependence of the conversion time _t_c normalized to the value of _τ_ at the
same temperature (blue stars). The excellent match between the trends of these two quantities proves that an enhancement in dielectric strength, that is, a higher orientational order, is
correlated with a greater stability of the MROL phase. The normalized dielectric strength and the value of log(_t_c/_τ_) remained almost constant up to _T_sub=206 K, where an abrupt
simultaneous drop in both parameters took place. Within the limits of our experimental method, we observed no enhancement in Δ_ε_N for films deposited at _T_sub≥213 K, which determined the
limiting temperature where we could detect a deviation from the OL. This behaviour suggests the onset of the stability of a liquid phase with enhanced orientational order below _T_*~213 K
(<_T_m). We conducted further experiments that verified that the increase in the dielectric susceptibility of the metastable liquid state also varies with the film thickness (Fig. 4). The
increase in Δ_ε_N exceeds 5.0 at 9 nm, and Δ_ε_Nmax decays progressively before settling to a constant value of 3.7, in films thicker than 15–20 nm. Given the purely additive contribution
of each layer to the dielectric strength in our experimental configuration33, we might imagine a higher BOO in proximity to the interfaces, penetrating for a few nanometres. Such a length
scale is larger than has been observed in metallic liquids in proximity to the crystal growth front34, where only a few atomic layers are affected. We were able to fit the interfacial excess
in Δ_ε_N with a single exponential with a characteristic decay length of 5.9±1.0 nm. This value can be treated as an upper bound for the actual spatial extent of the extra BOO in proximity
to the substrate, and thus also for the size of the region where the liquid dynamics is affected by the MRO35. To discern the impact of the interface-induced ordering caused by steric
effects from the possible impact of molecular interactions with the substrate36, we investigated MROL glycerol films grown on OL layers of the same material, previously deposited well above
_T_g (see Supplementary Methods for further details). We obtained quantitative agreement with the values measured in the single-layer films of comparable thickness, proving that the
interactions between the polyols and the substrate of the interdigitated electrodes (IDE) do not influence the structure of the MROL phase. To further analyse the transformation process, we
prepared glycerol films with different thicknesses, and we estimated the velocity _v_ of the conversion of the MROL into the OL by analysing the isothermal decay in Δ_ε_N at increasing
annealing temperatures. We found conversion rates on the order of 10−4 nm s−1 at _T_g+50 K for films thicker than 15 nm, while below this threshold, _v_ increases exponentially, with a
characteristic length of (7.2±2.0) nm, similar to the value found for Δ_ε_Nmax (Fig. 4). The outcome of this experiment, in which we kept _T_sub constant and we varied the layer thickness,
is in line with the data in Fig. 3, relative to measurements where we evaluated the conversion time at constant thickness, but at different _T_sub. Such behaviour, with the temperature
dependence observed for films of larger homologous molecules (Fig. 2), provides a further confirmation that higher values of Δ_ε_N correspond to a greater kinetic stability of the MROL.
Moreover, the linear decay of Δ_ε_N during isothermal transformation, indicating a constant transformation rate, is consistent with the scenario of a growth front-like transformation process
where the liquid–liquid interface between the two phases propagates through the film at a constant velocity, inversely proportional to the increase in orientational polarization of the
structural process in the liquid state37. DISCUSSION Further investigations will be necessary to unveil the molecular origin of the transformation process and the origin of such high kinetic
stability. A rationalization of the observed values of _t_c/_τ_ would require determining the lifetime of the LPSs and their stability against annealing. The MRO enrichment should persist
as long as the LPSs have not recovered their equilibrium concentration at a given temperature. Until the achievement of this condition, the MRO regions continuously destroy and nucleate,
driven by the minimization of local free energy, achieved via spatial reorganization at the time scale of _τ_ (refs 11, 28). This long-lasting behaviour permitted us to detect the
differences in the orientational polarization. At present, no simulation methods are available to compute the distribution and the exact lifetime of these metastable structures, even using
state-of-the-art computer resources11. However, recent Monte Carlo simulations have verified that metastable states associated with lower inherent structure energy (that is, LPSs) can
survive for lifetimes longer than the lifetimes predicted by simple diffusion models and can thus coexist with the isotropic liquid38. We speculate that our preparation method permitted us
to obtain similar long-lived structures, ensuring the persistence of the enhanced MRO in our systems. This success was possible because, as anticipated, performing PVD below _T_g has been
proven to be the most efficient way to age glasses. In the supporting information, we analyse the similarities between the results from our experiment and previous investigations of ordinary
and ultrastable glasses (Supplementary Figs S4–S6). Because of the importance of such domains in determining the properties of the glass transition, we believe that the procedure described
in this work, which allows the preparation of isotropic liquids enriched in conformations present in the glassy state, represents a possible method of achieving hidden liquid phases with
intriguing materials properties. We hope that our work will stimulate further investigation of this new liquid phase via different experimental approaches, which will make it possible to
test theoretical models to clarify the nature of the glass transition. METHODS MOLECULAR BEAM DEPOSITION IN THE GLASSY STATE Films of glycerol, threitol and xylitol (degree of purity
>99%, purchased from Aldrich), were prepared by organic molecular beam deposition directly on the IDE30,39,40, which made it possible to probe their dielectric response. The bulk material
was heated above its melting temperature in a crucible connected, via a pneumatic valve, to a vacuum chamber maintained at a pressure _p_~10–8 mbar. The thickness of the films, monitored
via a quartz crystal microbalance (QCM), was varied by switching the valve; the temperature of the crucible was adjusted to keep the evaporation rate below 3 × 10−2 nm s−1. During
evaporation, the temperature of the substrate, _T_sub, was maintained at 0.88_T_g; this value was very close to the temperature providing the best thermal and kinetic stability of the MROL,
as indicated by additional experiments performed on 50-nm-thick glycerol films grown at different _T_sub in the range from 0.75_T_g to _T_g. To prepare the glycerol bilayers, layers of
different thickness (6 and 36 nm) were deposited at 223 K (1.2_T_g) and immediately after deposition cooled to 0.88_T_g, where the evaporation of an 11-nm capping layer followed the same
protocol used for the single layers. DIELECTRIC MEASUREMENTS To perform _in situ_ dielectric spectroscopy while permitting a continuous evaporation of material on the target substrate, we
employed IDE structures, which present an open-electrode configuration. The sensors, produced by UV lithography, were composed of 100 gold fingers (height=200 nm; width=15 μm) deposited on a
quartz substrate. Each pair of fingers is spaced 1.25 μm apart, resulting in a total capacitance of 5 pF. Silver wires were glued on the connectors of the IDE via a thermally and
electrically conductive epoxy resin filled with silver nanoparticles (Ecolit E 325) and soldered to a high-vacuum feedthrough, which allowed connection to an impedance analyser (ALPHA,
Novocontrol). The intensity of the applied electric field _E_ was <106 V m−1, a regime in which the polarization response is linearly proportional to _E_. The IDE was mechanically
attached to a metallic plate located inside the vacuum chamber and held at the desired temperature by the combined action of a resistance heating and a cooling flux of gaseous nitrogen. A
Pt100 sensor was glued to the substrate of the IDE with Elecolit E 325 to constantly monitor _T_sub. In addition to the direct QCM measurements, the thickness of the film could also be
checked based on the dielectric signal, according to the equation Δ_C_∞=_N ε_0(_ε_∞−1)(_p_/_d_) _L_, where _L_ is the thickness of the film; Δ_C_∞ is the resulting change in the capacitance
of the system; _ε_0 is the vacuum permittivity; _ε_∞ is the dielectric constant of the material; _N_ is the total number of fingers; and _p_ and _d_ indicate the length of the fingers of the
IDE and their mutual distance, respectively. Such a procedure was used below 30 nm, where the error in determining the thickness by QCM, due to the different position of the two sensors,
exceeded 10%. The measured increase of the dielectric strength is a strong proof of the lack of bubbles inside our films, which, acting as dielectric cavities (_ε_∞vacuum<_ε_∞glycerol)
would reduce the polarization and thus the dielectric signal. CHARACTERIZATION OF THE MROL PHASE After deposition, the films were heated to a temperature _T_=_T_g+10 K and subsequently
annealed up to _T_g+50 K in steps of 1 K. Isothermal dielectric spectra were acquired at each temperature, in the frequency range between 10−1 and 106 Hz. The values of the relaxation time
_τ_ were derived from the relation _τ_=2_π_/_f_max, where _f_max is the frequency at which the imaginary part of the dielectric permittivity _ε_″(_ω_) shows a maximum. The values of the
dielectric strength were obtained by analysing the isothermal spectra via the empirical Havriliak–Negami (HN) function: where Δ_ε_, _τ_HN, _α_, and _β_ are the dielectric strength, the
relaxation time and the shape parameters, respectively, accounting for the width and the asymmetry of the loss curves. Further isothermal measurements were performed on glycerol films of
different thicknesses (9, 15, 18, 26, 30, 55, 89 nm): the temperature of the IDE was varied in the same range as above, but in steps of 5 K. The sample was held at each annealing temperature
for 2 h. The conversion rate into the OL phase, _v_, was estimated by fitting isothermal scans with linear decays _v_=Δ_l_/(_t_−_t_0), where Δ_l_ is the fraction of transformed film, and
_t_0 indicates the onset of measurement. The value of Δ_l_ is obtained from the time evolution of the dielectric strength under the assumption that Δ_ε_ is the sum of the contribution of the
two liquid phases averaged over their volume fractions according to Δ_ε_TOT=_x_OL·_R_Δ_ε_OL+_x_MROLΔ_ε_OL=_R_Δ_ε_OL(_L_−Δ_l_)/_L_+Δ_ε_OL·Δ_l_/_L_, where _x_ is the volume fraction,
_R_=Δ_ε_Nmax/Δ_ε_OL. The dielectric strength of the film normalized by the value in the OL, Δ_ε_OL, is thus given by Δ_ε_N(_t_−_t_0)=Δ_ε_TOT(_t_−_t_0)/(Δ_ε_OL·_L_)=_R_−(_R_−1)·Δ_l_/_L_,
which simplifies to Δ_ε_N (_t_0)−Δ_ε_N(_t_−_t_0)=(_R_−1)·Δ_l_/_L_. Finally, _v_=_L/_(_t_−_t_0)·[Δ_ε_N (_t_0)−Δ_ε_N(_t_−_t_0)]/(_R_−1). We ruled out the possibility that the larger values of
Δ_ε_ and of _τ_ observed in the medium-range crystalline order phase are actually induced by the presence of water or other contamination in the films. Water molecules would instead enhance
the structural relaxation time (plasticizing effect) and reduce the dielectric strength by breaking the OH bonds.41,42 Contamination by other impurities would also break the intermolecular
OH bonds, whose fluctuations contribute to the Δ_ε_ of polyols, and consequently reduce the dielectric strength. Furthermore, we considered whether the observed enhancement in the dielectric
strength and its thermal evolution could be due to the presence of ionic impurities intruded into the films during deposition, and subsequently desorbing at higher temperatures, during
annealing. Such a mechanism could mimic the conversion of the MROL phase into the OL. In addition to increase in the value of the dielectric strength, ionic contaminants would, in fact, also
yield a larger conductivity _σ_, see Supplementary Methods and Supplementary Equation S2. To verify the validity of this conjecture, we performed isothermal experiments at 228 K, a
temperature high enough to simultaneously observe the evolution of the dielectric strength and of the conductivity. The results (Supplementary Fig. S7) show that the dielectric strength
decays linearly with the annealing time, whereas the conductivity remains at a constant value, comparable with that of films deposited at _T_ »_T_g. This evidence strongly excludes the
possibility that the observed enhancement in dielectric strength is due to the presence of contaminants. ADDITIONAL INFORMATION HOW TO CITE THIS ARTICLE: Capponi, S. _et al._ Supercooled
liquids with enhanced orientational order. _Nat. Commun._ 3:1233 doi: 10.1038/ncomms2228 (2012). REFERENCES * Maxwell J. C. A dynamical theory of the electromagnetic field. _Philos. Trans.
R. Soc. Lond._ 155, 459–512 (1865). Article ADS Google Scholar * Angell C. A. Formation of glasses from liquids and biopolymers. _Science_ 267, 1924–1935 (1995). Article CAS ADS Google
Scholar * Debenedetti P. G., Stillinger F. H. Supercooled liquids and the glass transition. _Nature_ 410, 259–267 (2001). Article CAS ADS Google Scholar * Larini L., Ottochian A., De
Michele C., Leporini D. Universal scaling between structural relaxation and vibrational dynamics in glass-forming liquids and polymers. _Nat. Phys._ 4, 42–45 (2008). Article CAS Google
Scholar * Sastry S., Debenedetti P. G., Stillinger F. H. Signatures of distinct dynamical regimes in the energy landscape of a glass-forming liquid. _Nature_ 393, 554–557 (1998). Article
CAS ADS Google Scholar * Ngai K. L. _Relaxation and Diffusion in Complex Systems_ Springer (2011). * Corezzi S., Fioretto D., Rolla P. Bond-controlled configurational entropy reduction in
chemical vitrification. _Nature_ 420, 653–656 (2002). Article CAS ADS Google Scholar * Dyre J. C. Colloquium: the glass transition and elastic models of glass-forming liquids. _Rev.
Mod. Phys._ 78, 953–972 (2006). Article CAS ADS Google Scholar * Ossi P. M. _Disordered Materials_ Springer (2006). * Frank F. C. Supercooling of liquids. _Proc. R. Soc. Lond., Ser. A_
215, 43–46 (1952). Article CAS ADS Google Scholar * Kivelson D., Kivelson S. A., Zhao X. L., Nussinov Z., Tarjus G. A thermodynamic theory of supercooled liquids. _Physica A_ 219, 27–38
(1995). Article CAS ADS Google Scholar * Tarjus G., Kivelson S. A., Nussinov Z., Viot P. The frustration-based approach of supercooled liquids and the glass transition: a review and
critical assessment. _J. Phys.:Condes. Matter_ 17, R1143–R1182 (2005). CAS ADS Google Scholar * Shintani H., Tanaka H. Frustration on the way to crystallization in glass. _Nat. Phys._ 2,
200–206 (2006). Article CAS Google Scholar * Ma D., Stoica A. D., Wang X. L. Power-law scaling and fractal nature of medium-range order in metallic glasses. _Nat. Mater._ 8, 30–34 (2009).
Article CAS ADS Google Scholar * Salmon P. S., Martin R. A., Mason P. E., Cuello G. J. Topological versus chemical ordering in network glasses at intermediate and extended length
scales. _Nature_ 435, 75–78 (2005). Article CAS ADS Google Scholar * Sheng H. W., Luo W. K., Alamgir F. M., Bai J. M., Ma E. Atomic packing and short-to-medium-range order in metallic
glasses. _Nature_ 439, 419–425 (2006). Article CAS ADS Google Scholar * Jiang W. H., Liu F. X., Choo H., Liaw P. K. Effect of structural relaxation on mechanical behavior of a Zr-based
bulk-metallic glass. _Mater. Trans._ 48, 1781–1784 (2007). Article CAS Google Scholar * Zhilyaev P., Nurislamova G. V., Kim B. K., Barò M. D., Szpunar J. A., Langdon T. G. Experimental
parameters influencing grain refinement and microstructural evolution during high-pressure torsion. _Acta Mater._ 51, 753–765 (2003). Article CAS Google Scholar * Zeng Q. et al.
Long-range topological order in metallic glass. _Science_ 332, 1404–1406 (2011). Article CAS ADS Google Scholar * Heideman C. et al. The synthesis and characterization of new
[(BiSe)1.10]m[NbSe2]n, [(PbSe)1.10]m[NbSe2]n, [(CeSe)1.14]m[NbSe2]n and [(PbSe)1.12]m[TaSe2]n misfit layered compounds. _J. Solid State Chem._ 181, 1701–1706 (2008). Article CAS ADS
Google Scholar * Swallen S. F. et al. Organic glasses with exceptional thermodynamic and kinetic stability. _Science_ 315, 353–356 (2007). Article CAS ADS Google Scholar * Guo Y. L. et
al. Ultrastable nanostructured polymer glasses. _Nat. Mater._ 11, 337–343 (2012). Article CAS ADS Google Scholar * Fakhraai Z., Forrest J. A. Measuring the surface dynamics of glassy
polymers. _Science_ 319, 600–604 (2008). Article CAS Google Scholar * Kearns K. L., Swallen S. F., Ediger M. D., Wu T., Sun Y., Yu L. Hiking down the energy landscape: progress toward the
Kauzmann temperature via vapor deposition. _J. Phys. Chem. B_ 112, 4934–4942 (2008). Article CAS Google Scholar * Pauling L. _The Nature of the Chemical Bond_ Cornell University Press
(1931). * Douglas B. E., McDaniel D. H., Alexander J. J. _Concepts and Models of Inorganic Chemistry_ John Wiley & Sons (1994). * Frohlich H. _Theory of Dielectrics: Dielectric Constant
and Dielectric Loss_ Clarendon Press (1958). * Tanaka H., Kawasaki T., Shintani H., Watanabe K. Critical-like behaviour of glass-forming liquids. _Nat. Mater._ 9, 324–331 (2010). Article
CAS ADS Google Scholar * Asai M., Shibayama M., Koike Y. Common origin of dynamics heterogeneity and cooperatively rearranging region in polymer melts. _Macromolecules_ 44, 6615–6624
(2011). Article CAS ADS Google Scholar * Capponi S., Napolitano S., Behrnd N. R., Couderc G., Hulliger J., Wübbenhorst M. Structural relaxation in nanometer thin layers of glycerol. _J.
Phys. Chem. C_ 114, 16696–16699 (2010). Article CAS Google Scholar * Hernandez M., Lopez-Manchado M. A., Sanz A., Nogales A., Ezquerra T. A. Effects of strain-induced crystallization on
the segmental dynamics of vulcanized natural rubber. _Macromolecules_ 44, 6574–6580 (2012). Article ADS Google Scholar * Paluch M., Grzybowska K., Grzybowski A. Effect of high pressure on
the relaxation dynamics of glass-forming liquids. _J. Phys.:Condes. Matter_ 19, 205117 (2007). ADS Google Scholar * Peter S., Napolitano S., Meyer H., Wübbenhorst M., Baschnagel J.
Modeling dielectric relaxation in polymer glass simulations: dynamics in the bulk and in supported polymer films. _Macromolecules_ 41, 7729–7743 (2008). Article CAS ADS Google Scholar *
Oh S. H., Kauffmann Y., Scheu C., Kaplan W. D., Ruhle M. Ordered liquid aluminum at the interface with sapphire. _Science_ 310, 661–663 (2005). Article CAS ADS Google Scholar * Watanabe
K., Kawasaki T., Tanaka H. Structural origin of enhanced slow dynamics near a wall in glass-forming systems. _Nat. Mater._ 10, 512–520 (2011). Article CAS ADS Google Scholar * Napolitano
S., Wübbenhorst M. The lifetime of the deviations from bulk behaviour in polymers confined at the nanoscale. _Nat. Commun._ 2, 260 (2011). Article ADS Google Scholar * Swallen S. F.,
Traynor K., McMahon R. J., Ediger M. D., Mates T. E. Stable glass transformation to supercooled liquid via surface-initiated growth front. _Phys. Rev. Lett._ 102, 065503 (2009). Article ADS
Google Scholar * Jack R. L., Hedges L. O., Garrahan J. P., Chandler D. Preparation and relaxation of very stable glassy states of a simulated liquid. _Phys. Rev. Lett._ 107, 275702
(2011). Article ADS Google Scholar * Rotella C., Napolitano S., Wübbenhorst M. Segmental mobility and glass transition temperature of freely suspended ultrathin polymer membranes.
_Macromolecules_ 42, 1415–1417 (2009). Article CAS ADS Google Scholar * Scott M. C., Stevens D. R., Bochinski J. R., Clarke L. I. Dynamics within alkylsiloxane self-assembled monolayers
studied by sensitive dielectric spectroscopy. _ACS Nano_ 2, 2392–2400 (2008). Article CAS Google Scholar * Hayashi Y., Puzenko A., Balin I., Ryabov Y. E., Feldman Y. Relaxation dynamics
in glycerol-water mixtures. 2. Mesoscopic feature in water rich mixtures. _J. Phys. Chem. B_ 109, 9174–9177 (2005). Article CAS Google Scholar * Hayashi Y., Puzenko A., Feldman Y. Slow
and fast dynamics in glycerol-water mixtures. _J. Non-Cryst. Solids_ 352, 4696–4703 (2006). Article CAS ADS Google Scholar Download references ACKNOWLEDGEMENTS We thank Haijme Tanaka
(Institute of Industrial Science, University of Tokyo) for a fruitful discussion. S.C. and M.W. acknowledge financial support from the Research Council of the K.U. Leuven, projects No.
OT/06/30 and OT/11/065, and financial support from FWO (Fonds Wetenschappelijk Onderzoeks—Vlaanderen) within the project G.0642.08. AUTHOR INFORMATION Author notes * Simona Capponi Present
address: Present address: School of Physics, University College Dublin, Belfield, Dublin, Ireland., AUTHORS AND AFFILIATIONS * Department of Physics and Astronomy, Laboratory of Acoustics
and Thermal Physics, Katholieke Universiteit Leuven, Celestijnenlaan 200D, Leuven, 3001, Belgium Simona Capponi & Michael Wübbenhorst * Laboratory of Polymer and Soft Matter Dynamics,
Faculté des Sciences, Université Libre de Bruxelles (ULB), Boulevard du Triomphe, Bâtiment NO, Bruxelles, 1050, Belgium Simone Napolitano Authors * Simona Capponi View author publications
You can also search for this author inPubMed Google Scholar * Simone Napolitano View author publications You can also search for this author inPubMed Google Scholar * Michael Wübbenhorst
View author publications You can also search for this author inPubMed Google Scholar CONTRIBUTIONS S.C., S.N. and M.W contributed equally to this work. CORRESPONDING AUTHOR Correspondence to
Simone Napolitano. ETHICS DECLARATIONS COMPETING INTERESTS The authors declare no competing financial interests. SUPPLEMENTARY INFORMATION SUPPLEMENTARY METHODS Supplementary Figures S1-S7,
Supplementary Methods and Supplementary References (PDF 867 kb) RIGHTS AND PERMISSIONS Reprints and permissions ABOUT THIS ARTICLE CITE THIS ARTICLE Capponi, S., Napolitano, S. &
Wübbenhorst, M. Supercooled liquids with enhanced orientational order. _Nat Commun_ 3, 1233 (2012). https://doi.org/10.1038/ncomms2228 Download citation * Received: 30 August 2012 *
Accepted: 26 October 2012 * Published: 04 December 2012 * DOI: https://doi.org/10.1038/ncomms2228 SHARE THIS ARTICLE Anyone you share the following link with will be able to read this
content: Get shareable link Sorry, a shareable link is not currently available for this article. Copy to clipboard Provided by the Springer Nature SharedIt content-sharing initiative