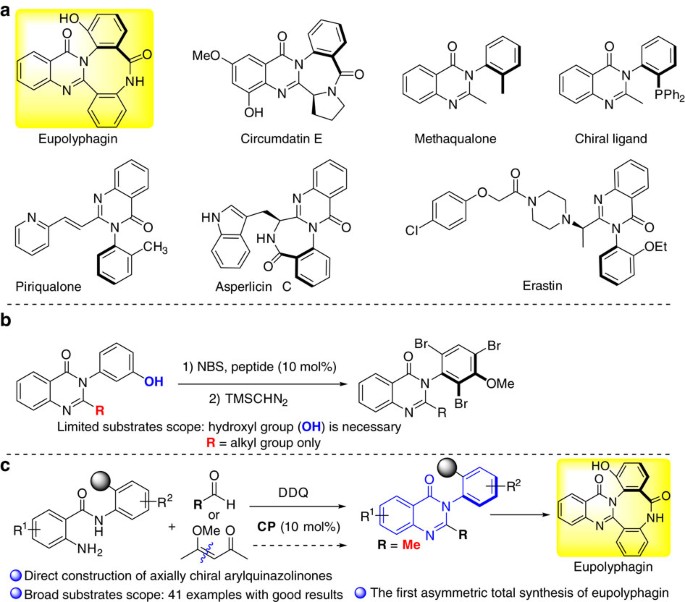
- Select a language for the TTS:
- UK English Female
- UK English Male
- US English Female
- US English Male
- Australian Female
- Australian Male
- Language selected: (auto detect) - EN
Play all audios:
ABSTRACT The axially chiral arylquinazolinone acts as a privileged structural scaffold, which is present in a large number of natural products and biologically active compounds as well as in
chiral ligands. However, a direct catalytic enantioselective approach to access optically pure arylquinazolinones has been underexplored. Here we show a general and efficient approach to
access enantiomerically pure arylquinazolinones in one-pot fashion catalysed by chiral phosphoric acids. A variety of axially chiral arylquinazolinones were obtained in high yields with good
to excellent enantioselectivities under mild condition. Furthermore, we disclosed a method for atroposelective synthesis of alkyl-substituted arylquinazolinones involving Brønsted
acid-catalysed carbon–carbon bond cleavage strategy. Finally, the asymmetric total synthesis of eupolyphagin bearing a cyclic arylquinazolinone skeleton was accomplished with an overall
yield of 32% in six steps by utilizing the aforementioned methodology. SIMILAR CONTENT BEING VIEWED BY OTHERS ORGANOCATALYTIC ATROPOSELECTIVE HETEROCYCLOADDITION TO ACCESS AXIALLY CHIRAL
2-ARYLQUINOLINES Article Open access 13 October 2021 METAL-FREE OXIDATIVE CROSS-COUPLING ENABLED PRACTICAL SYNTHESIS OF ATROPISOMERIC QUINOL AND ITS DERIVATIVES Article Open access 22 April
2021 BRØNSTED ACID-ENHANCED COPPER-CATALYZED ATROPOSELECTIVE CYCLOISOMERIZATION TO AXIALLY CHIRAL ARYLQUINOLIZONES VIA DEAROMATIZATION OF PYRIDINE Article Open access 18 January 2022
INTRODUCTION The axially chiral arylquinazolinones constitutes a privileged structural scaffold found in a large number of natural products and biologically active compounds as exemplified
by those shown in Fig. 1a (refs 1-8, 2, 3, 4, 5, 6, 7, 8). This motif represents a well-known class of therapeutics that displays hypnotic, anxiolytic, anticonvulsant and antitumor effects.
For example, eupolyphagin1 and asperlicins2 are known as potent cholescystokinin antagonists; Erastin3 is an antitumor agent used for targeting selective tumor cells containing oncogenic
RAS, which yields ferroptosis by changing the mitochondrial voltage-dependent anion channel gating thus allowing cations into the mitochondria, resulting to the release of oxidative species.
In addition, this motif could also be used as chiral ligand for asymmetric catalysis9,10. The significance of these privileged axially chiral skeletons has led to a great demand for
efficient synthetic methods, particularly those producing enantiomerically pure arylquinazolinones. In this regard, there have only existed a few transformations for the construction of
these structural skeletons, which usually employ either enantiopure starting materials or conventional resolution to construct the optically pure arylquinazolinone derivatives11,12. Levacher
and co-workers developed a divergent stereoselective synthesis of bridged arylquinazolinones by using the popular Meyers’ diastereoselective lactamization under dehydrating conditions12.
Miller reported a pioneering approach for accessing the enantioenriched arylquinazolinone via peptide-catalysed atroposelective bromination of preformed quinazolinones, representing the only
asymmetric catalytic example (Fig. 1b)13. However, the hydroxyl group is necessary to direct the atroposelective bromination, and only alkyl group is tolerant in the reaction, which
restricted the further application for diversity-oriented-synthesis. Although these were elegant and creative strategies towards the synthesis of these skeletons, the direct catalytic
enantioselective approach to access optically pure arylquinazolinones was yet to be described and would be of great value with respect to synthetic efficiency and broader substrate scope.
Since the pioneering reports of Akiyama14 and Terada15, chiral phosphoric acids have been widely used as organocatalysts in the simultaneous activation of nucleophile and/or electrophile for
asymmetric transformations16,17,18,19. Recently, several elegant examples for asymmetric synthesis of axially chiral compounds20,21,22,23,24,25,26 were reported by using phosphoric
acids27,28,29,30,31,32,33,34. In this context, Akiyama and co-workers achieved a significant breakthrough by using phosphoric acid to enable the asymmetric atroposelective bromination,
providing a useful approach for accessing axially biaryldiols27. Recently, they also developed an enantiodivergent synthesis of axially chiral biaryls via asymmetric reductive amination
catalysed by a chiral phosphoric acid28. Motivated by these successful examples and the previous efforts on phosphoric acid-catalysed asymmetric synthesis of dihydroquinazolinones35,36 via
amidation37, we envisioned that chiral phosphoric acids may also be capable of facilitating the construction of axially chiral arylquinazolinones enantioselectively by accelerating the imine
formation and the intramolecular nucleophilic addition to form hemiaminal followed by the oxidative dehydrogenation (Fig. 1c). However, several challenges are associated with the direct
asymmetric construction of the axially chiral arylquinazolinones in a one-pot fashion: The atroposelective construction of axially chiral arylquinazolinones has rarely been investigated in
asymmetric catalysis; the previous reports on asymmetric synthesis of dihydroquinazolinones did not investigate the _N_-aryl anthranilamides; the concomitant control of the
stereoselectivities of dihydroquinazolinones bearing a stereogenic carbon center and an axial chirality might be a great challenge; the development of a suitable oxidation condition for
preserving the enantiopurity needs to be addressed. As part of our continuous efforts in the asymmetric construction of axially chiral compounds38 and inspired by the elegant work of
Rodriguez and Bonne involving oxidative central-to-axial chirality conversion strategy39,40, here we describe the results of the investigation on addressing the aforementioned challenges,
leading to the phosphoric acid-catalysed highly enantioselective synthesis of axially chiral arylquinazolinones with high enantiopurity and structural diversity (Fig. 1c). In addition, the
phosphoric acid-catalysed carbon–carbon bond cleavage for direct atroposelective construction of alkyl-substituted arylquinazolinones under mild condition suggests an approach of great
importance to medicinal chemistry and diversity-oriented synthesis. RESULTS OPTIMIZATION OF REACTION CONDITIONS To validate the feasibility of the hypothesis, our initial investigations were
carried out by using the reaction of _N_-aryl anthranilamides (1A) and benzaldehyde (2A) as the model substrates with 10 mol% of phosphoric acid CP1 in CHCl3 at 0 °C in the presence of
2,3-dichloro-5,6-dicyano-1,4-benzoquinone (DDQ). Despite its high steric hindrance, the reaction proceeded cleanly to give the axially chiral arylquinazolinone (3A) with 68% ee, albeit with
a low conversion (Table 1, entry 1). This preliminary result obviously demonstrated that the control of the axial chirality of arylquinazolinone by using chiral phosphoric acid-catalysed
asymmetric cyclocondensation and oxidative dehydrogenation is feasible. To improve the reactivity and stereoselectivity, we next turned our attention to evaluate the different phosphoric
acid catalysts available. As shown in Table 1, the electron property and steric bulk on the aromatic ring, as well as the backbone displayed remarkable effects on the outcome of the reaction
(Table 1, entries 2–8). Fortunately, we found the catalyst CP3 gave the best yield of 88% and enantioselectivity of 96% ee (Table 1, entry 3). It is worthy of note that the more acidic
_N_-triflylphosphoramides CP7, which were first reported by Yamamoto and Nakashima41, did not improve the results (entry 7). The solvent had an important role in the transformation, and
CHCl3 was proved to be the best choice (Table 1, entries 9–12). Expectedly, the presence of 4 Å molecular sieves had a remarkable effect on the enantioselectivity because of the generation
of water during the reaction (Table 1, entry 13). Further optimization of the conditions revealed that the reaction was complete to furnish 3A in 96% isolated yield with 96% ee (Table 1,
entry 15). It was demonstrated that the oxidant DDQ could be added after the first cyclization reaction was accomplished without any effect on the reactivity and stereoselectivity (Table 1,
entry 16). The absolute configuration of 3A was determined as being (_a_ _R_) by X-ray diffraction analysis (See Supplementary Fig. 1, CCDC 1509246) and those of other products were assigned
by analogy. SUBSTRATE SCOPE With the optimal reaction conditions in hand, we set out to explore the substrate generality of this transformation. First, we evaluated various substituted
anthranilamides (1B–1M). Most reactions reached completion within 96 h and gave axially chiral arylquinazolinones (Table 2, 3B–3M) in high yields (90–99%) with good to exellent
enantioselectivities (85–97% ee). The results revealed that both the position and electronic property of the substituents on the aromatic ring have a slight effect on the reaction efficiency
and enantioselectivity of this transformation. With respect to the _N_-aryl group, it is noteworthy to point out that the ortho group is not only restricted to _tert_-butyl (_t_Bu) group,
and the bromo, iodo, phenyl or diphenyl phosphine oxide (Ph2OP) group at this position could also be suitable substrates that yielded the desired products (Table 2, 3N–3U) with good to
excellent enantiocontrol (83–95% ee). Notably, the presence of Cl, Br or I substituent in the arylquinazolinone derivatives obtained is very important for setting up a compound library due
to the high reactivity in many transition-metal-catalysed reactions42. Encouraged by these results, we, therefore, expanded the generality of the reaction with regard to the variation of the
aldehydes. Various aromatic aldehydes were applicable and afforded the desired products with good yields and excellent enantioselectivities (Table 3). It was shown that the position and the
electronic nature of the substituents on the aromatic ring have limited effect on the stereoselectivity. For example, aldehydes bearing electron withdrawing (R=F, Cl, Br) or
electron-donating groups (R=Me, OMe) at different positions (para, meta, ortho) of the phenyl ring reacted efficiently to afford the corresponding products 4A–4J with 63–98% yields and
85–95% ee. Moreover, the 2-furaldehyde and 2-naphthaldehyde could be well tolerated without obviously affecting the reaction results (4K and 4L). When aliphatic aldehydes were investigated,
the reaction proceeded very messy under the optimized conditions. To our delight, the expected arylquinazolinone (4M) was produced when DDQ was added after the first step was completed. In
the meanwhile, an unexpected product 4N was obtained with excellent enantioselectivity (92% ee) using phenyliodinediacetate as oxidant. Disappointedly, although we tried our best to optimize
the reaction conditions for linear aliphatic aldehydes, no good results were obtained. C–C BOND CLEAVAGE STRATEGY To further expand the generality to get alkyl-substituted
arylquinazolinone, we were interested in a promising strategy via phosphorous acid-catalysed cyclocondensation and selective C–C bond cleavage to meet this challenge. As we all known, C–C
bond cleavage is a topic of significant importance in organic synthesis43,44. Despite significant advances in the past decades, it is still a big challenge to selectively cleave unstrained
C–C bond by using an organocatalyst. In this regard, Zhou and co-workers45 developed an elegant approach to deliver quinazolinones involving organocatalytic reactions of ketoesters/diketones
with 2-aminobenzamides and selective C–C bond cleavage. Inspired by this beautiful work, we envisioned that the axially chiral arylquinazolinone might be produced by using the reaction of
_N_-aryl anthranilamides (1H) with ketoester or diketone under the catalysis of chiral phosphoric acids. To our disappointment, almost no desired product was obtained when ketoester was
tested. After substantial optimization (for optimized details, see Supplementary Table 1), the axially chiral arylquinazolinone 6A was obtained with good results by treating the reaction
with 4-methoxypentenone 5A and 1H catalysed by a more acidic _N_-triflylphosphoramides CP9 at 60 °C in a mixture of _c_-hexane and CHCl3 in the presence of MgSO4 (Table 4, product 6A). The
absolute configuration of 3A was determined to be (_a_ _R_) by X-ray diffraction analysis (Supplementary Fig. 2, CCDC 1509247). Having identified the optimized conditions, we proceeded to
investigate the substrate scope of the reaction. Several substituted _N_-aryl anthranilamides were successfully applied in the reaction with 4-methoxypentenone (Table 4). The corresponding
axially chiral methyl-substituted arylquinazolinones 6A–6F were isolated with good chemical yields (75–95%) and enantioselectivities (83–95% ee). It should be noted that other diketone
derivatives produce the corresponding products with poor results, which shows the limitation of the current method. However, the resultant product 6F can be further transformed into other
diversely functionalized axially chiral arylquinazolinones 7A–7C without any erosion of enantioselectivities, thus expanding the substrate scope and compensating the limitation to some
extent. ASYMMETRIC TOTAL SYNTHESIS OF EUPPLYPHAGIN The efficient construction of a series of functionalized axially chiral arylquinazolinones motivated our investigation of the asymmetric
total synthesis of eupolyphagin (Fig. 2). Our primary concept is to construct the key axially chiral intermediate 8C by using the above-mentioned approach and followed by construction of
eight-member lactam 8E via palladium-catalysed carbonylation of aryl halides. We started our investigation from the optimization of the construction of the key axially chiral intermediate
8C. After great efforts, the corresponding product was obtained with moderate enantioselectivity by treating the reaction with 8B and 2-FmocNHPhCHO catalysed by (_S_)-CP3 at 40 °C (other
_N_-protected aldehydes and anthraniamides gave poor results. For details, see Supplementary Table 2). Fortunately, the enantiopurity of 8C can be enriched to 95% ee by using
recrystallization from dichloromethane (DCM)/ethyl acetate (EA). The free amine 8D was obtained by removing the Fmoc protection group in excellent yield without any effect on the
enantioselectivity. Although palladium-catalysed carbonylation of aryl halides provided a simple method that generated a range of carboxylic acid derivatives, there was only one report for
the construction of eight-member lactam46. Considering the complicated catalyst and high carbon monoxide pressure that may have a strong effect on the conversion of aryl iodide 8D to the key
intermediate 8E, we selected Arndtsen’s modified procedure47. Our preliminary investigation by using _t_Bu3P as ligand and TBACl as chloride source afforded the desired 9E with moderate
yield. Further optimization (for optimized details, see Supplementary Table 3) resulted in an improved protocol which could provide 8E in satisfactory isolated yield (95%) without any effect
on enantioselectivity. Finally, the methyl protecting group was removed by using BBr3 to obtain eupolyphagin as a white solid in 98% yield with 95% ee48. It should be emphasized that it
represents the asymmetric total synthesis of this axially chiral natural product and the overall yield for the six steps is 32%. CONTROLLED EXPERIMENTS AND PROPOSED REACTION PROCESSES In
order to get some insights of the reaction mechanism, we have conducted several control experiments (Fig. 3). In the beginning, we performed a control experiment in the absence of oxidant
and successfully isolated the key aminal intermediate 9A in 90% yield and 96% ee and only one diastereoisomer was detected (dr>20:1), indicating that the double chirality was controlled
completely. The following oxidation of 9A with DDQ afforded the desired product 3A with excellent yield and 94% ee (Fig. 3a). However, when we isolated another intermediate 9B, the crude-NMR
clearly showed that the dr of 9B was 20:1. The two diastereoisomers yielded the corresponding axially chiral product 3T with opposite configuration (Fig. 3b). In addition, when we attempted
to isolate the intermediate 9C, we found that the two diastereoisomers (dr=3.6:1 in CDCl3) cannot be separated on silica gel chromatography and only two peeks were detected by chiral
high-performance liquid chromatography analysis. The transformation of this intermediate afforded the final product 3P with excellent yield and 81% ee (Fig. 3c). Based on these results, we
deduced that the C–N bond can rotate freely in the intermediate 9C and the central chirality can be efficiently transferred into axial chirality during the oxidation. These results also
indicated that the axial chirality of the final product was controlled in the cyclization step and the control of the central chirality was the key point for achieving good
enantioselectivity. As for the synthesis of alkyl substituted arylquinazolinone, we have isolated the enamine 9D and failed to separate the cyclization intermediate due to the unstability.
The final product 6A was isolated with 50% yield and 77% ee under standard condition (Fig. 3d). On the basis of above-mentioned control experiments and previous reports35,36,37, we proposed
tentative reaction processes. As shown in Fig. 3e, these reactions probably go through the typical Brønsted acid-catalysed aminal formation process. Initially, imines or enamines were
generated from the condensation of 1 with aldehyde or 4-methoxypentenone, respectively. Then _N_,_N_-aminal cyclization intermediates were produced via intramolecular amidation. Finally, the
desired arylquinazolinones were obtained by dehydrogenation in the presence of an oxidant or the C−C bond cleavage under acidic condition48. It is clear that the enantioselectivity is
determined during the step of intramolecular nucleophilic attack under the catalysis of chiral Brønsted acid. At the current stage, the asymmetric induction and the chirality transfer from
central to axial chirality remains unclear, which is ongoing in our laboratory. DISCUSSION We have successfully developed a general and efficient approach to access enantiomerically pure
arylquinazolinones catalysed by chiral phosphoric acids. A series of axially chiral arylquinazolinones with potential biological activities were obtained in high yields (up to 99%) with good
to excellent enantioselectivities (up to 97% ee) under mild conditions. To expand the reaction generality, we further disclosed a method of great importance for the atroposelective
construction of alkyl-substituted arylquinazolinones using Brønsted acid-catalysed carbon–carbon bond cleavage strategy. Applying this methodology, the asymmetric total synthesis of axially
chiral natural product eupolyphagin was achieved with an overall yield of 32% in six steps. We anticipate that this promising strategy could be applied to the synthesis of other natural
products and the axially chiral arylquinazolinones may have further potential applications in asymmetric catalysis and drug discovery. METHODS PROCEDURE FOR THE ENANTIOSELECTIVE SYNTHESIS OF
3 AND 4A–4L To a dry Schlenk tube (10 ml), 400 mg activated 4 Å MS (molecular sieves) was added and then the MS was reactivated under reduced pressure for 15 min. After the tube was cooled
down, _N_-aryl anthranilamides 1 (0.10 mmol), CP3 (7.0 mg, 0.01 mmol), DDQ (31.8 mg, 0.14 mmol) and anhydrous CHCl3 (4.0 ml) was added under Ar. The resulting mixture was stirred for 10 min
at 0 °C, and then aromatic aldehyde 2 (0.2 mmol) was added in one portion. After stirred for 96 h at 0 °C, the mixture was purified by flash column chromatography on silica gel (gradient
elution with PE/EA) to give the pure product. PROCEDURE FOR THE ENANTIOSELECTIVE SYNTHESIS OF 6 To a 10 ml dry Schlenk tube, _N_-aryl anthranilamides 1H, 1M or 1V-1Y (0.10 mmol), 5A (22.8
mg, 0.2 mmol), MgSO4 (6.0 mg), CP9 (8.0 mg, 0.01 mmol) and anhydrous _c_-hexane/CHCl3=1/1 (4.0 ml) was added under Ar. The resulting solution was heated to 60 °C. After stirred for 96 h, the
solution was cooled down to room temperature and then purified by flash column chromatography on silica gel (gradient elution with PE/EA) to give the pure product. For NMR spectra of the
new compounds in this article, see Supplementary Figs 3–209. Full experimental details and characterization of compounds are given in Supplementary Notes 1–5. DATA AVAILABILITY The X-ray
crystallographic coordinates for structures reported in this Article have been deposited at the Cambridge Crystallographic Data Centre (CCDC), under deposition numbers CCDC 1509246, CCDC
1509247. Crystal data and structure refinement for 3A and 6F were displayed in Supplementary Tables 4 and 5. These data can be obtained free of charge from The Cambridge Crystallographic
Data Centre via http://www.ccdc.cam.ac.uk/data_request/cif. ADDITIONAL INFORMATION HOW TO CITE THIS ARTICLE: Wang, Y.-B. _et al_. Brønsted acid-catalysed enantioselective construction of
axially chiral arylquinazolinones. _Nat. Commun._ 8, 15489 doi: 10.1038/ncomms15489 (2017). PUBLISHER’S NOTE: Springer Nature remains neutral with regard to jurisdictional claims in
published maps and institutional affiliations. REFERENCES * Jiang, H.-L. et al. New isocoumarins and alkaloid from Chinese insect medicine, Eupolyphaga sinensis Walker. _Fitoterapia_ 83,
1275–1280 (2012). CAS PubMed Google Scholar * Joshi, B. K., Gloer, J. B., Wicklow, D. T. & Dowd, P. F. Sclerotigenin: a new antiinsectan benzodiazepine from the sclerotia of
Penicillium sclerotigenum. _J. Nat. Prod._ 62, 650–652 (1999). CAS PubMed Google Scholar * Rahbæk, L. & Breinholt, J. Circumdatins D, E, and F: further fungal benzodiazepine analogues
from Aspergillus ochraceus. _J. Nat. Prod._ 62, 904–905 (1999). PubMed Google Scholar * Yagoda, N. et al. RAS–RAF–MEK-dependent oxidative cell death involving voltage-dependent anion
channels. _Nature_ 447, 865–869 (2007). ADS CAS Google Scholar * Dai, J.-R. et al. Circumdatin G, a new alkaloid from the fungus _Aspergillus ochraceus_. _J. Nat. Prod._ 64, 125–126
(2001). CAS PubMed Google Scholar * Sun, H. H., Barrow, C. J., Sedlock, D. M., Gillum, A. M. & Cooper, R. Benzomalvins, new suhstance P inhibitors from a Penicillium sp. _J.
Antibiot._ 47, 515–522 (1994). CAS PubMed Google Scholar * Sun, H. H., Barrow, C. J. & Cooper, R. Benzomalvin D, a new 1, 4-benzodiazepine atropisomer. _J. Nat. Prod._ 58, 1575–1580
(1995). CAS Google Scholar * Gangadhar, N. M. & Stockwell, B. R. Chemical genetic approaches to probing cell death. _Curr. Opin. Chem. Biol._ 11, 83–87 (2007). CAS PubMed Google
Scholar * Rahbæk, L., Breinholt, J., Frisvad, J. C. & Christophersen, C. Circumdatin A, B, and C: three new benzodiazepine alkaloids isolated from a culture of the fungus _Aspergillus
ochraceus_. _J. Org. Chem._ 64, 1689–1692 (1999). PubMed Google Scholar * Dai, X., Wong, A. & Virgil, S. C. Synthesis and resolution of quinazolinone atropisomeric phosphine ligands.
_J. Org. Chem._ 63, 2597–2600 (1998). CAS PubMed Google Scholar * Tokitoh, T. et al. Diastereoselective synthesis of atropisomeric 3-(2-substituted aryl) quinazolin-4-ones and their
stereochemical properties. _Heterocycles_ 70, 93–99 (2006). CAS Google Scholar * Penhoat, M. et al. New development of Meyers’ methodology: stereoselective preparation of an axially chiral
5, 7-fused bicyclic lactam related to circumdatins/benzomalvins and asperlicins. _Tetrahedron: Asymmetry_ 17, 281–286 (2006). CAS Google Scholar * Diener, M. E., Metrano, A. J., Kusano,
S. & Miller, S. J. Enantioselective synthesis of 3-arylquinazolin-4 (3 H)-ones via peptide-catalysed atroposelective bromination. _J. Am. Chem. Soc._ 137, 12369–12377 (2015). CAS PubMed
PubMed Central Google Scholar * Akiyama, T., Itoh, J., Yokota, K. & Fuchibe, K. Enantioselective Mannich-type reaction catalysed by a chiral Brønsted acid. _Angew. Chem. Int. Ed._
43, 1566–1568 (2004). CAS Google Scholar * Uraguchi, D. & Terada, M. Chiral Brønsted acid-catalysed direct Mannich reactions via electrophilic activation. _J. Am. Chem. Soc._ 126,
5356–5357 (2004). CAS PubMed Google Scholar * Akiyama, T. Stronger Brønsted acids. _Chem. Rev._ 107, 5744–5758 (2007). CAS PubMed Google Scholar * Terada, M. Binaphthol-derived
phosphoric acid as a versatile catalyst for enantioselective carbon-carbon bond forming reactions. _Chem. Commun._ 2008, 4097–4112 (2008). Google Scholar * Rueping, M., Kuenkel, A. &
Atodiresei, I. Chiral Brønsted acids in enantioselective carbonyl activations–activation modes and applications. _Chem. Soc. Rev._ 40, 4539–4549 (2011). CAS PubMed Google Scholar *
Parmar, D., Sugiono, E., Raja, S. & Rueping, M. Complete field guide to asymmetric BINOL-phosphate derived Brønsted acid and metal catalysis: history and classification by mode of
activation; Brønsted acidity, hydrogen bonding, ion pairing, and metal phosphates. _Chem. Rev._ 114, 9047–9153 (2014). CAS PubMed Google Scholar * Gustafson, J. L., Lim, D. & Miller,
S. J. Dynamic kinetic resolution of biaryl atropisomers via peptide-catalysed asymmetric bromination. _Science_ 328, 1251–1255 (2010). ADS CAS PubMed PubMed Central Google Scholar *
Garand, E. et al. Determination of noncovalent docking by infrared spectroscopy of cold gas-phase complexes. _Science_ 335, 694–698 (2012). ADS CAS PubMed PubMed Central Google Scholar
* Barrett, K. T., Metrano, A. J., Rablen, P. R. & Miller, S. J. Spontaneous transfer of chirality in an atropisomerically enriched two-axis system. _Nature_ 509, 71–75 (2014). ADS CAS
PubMed PubMed Central Google Scholar * Miyaji, R., Asano, K. & Matsubara, S. Bifunctional organocatalysts for the enantioselective synthesis of axially chiral isoquinoline N-Oxides.
_J. Am. Chem. Soc._ 137, 6766–6769 (2015). CAS PubMed Google Scholar * Lotter, D., Neuburger, M., Rickhaus, M., Häussinger, D. & Sparr, C. Stereoselective arene-forming aldol
condensation: synthesis of configurationally stable oligo-1, 2-naphthylenes. _Angew. Chem. Int. Ed._ 55, 2920–2923 (2016). CAS Google Scholar * Zheng, J., Cui, W.-J., Zheng, C. & You,
S.-L. Synthesis and application of chiral spiro Cp ligands in rhodium-catalyzed asymmetric oxidative coupling of biaryl compounds with alkenes. _J. Am. Chem. Soc._ 138, 5242–5245 (2016). CAS
PubMed Google Scholar * Yu, C., Huang, H., Li, X., Zhang, Y. & Wang, W. Dynamic kinetic resolution of biaryl lactones via a chiral bifunctional amine thiourea catalysed highly
atropo-enantioselective transesterification. _J. Am. Chem. Soc._ 138, 6956–6959 (2016). CAS PubMed Google Scholar * Mori, K. et al. Enantioselective synthesis of multisubstituted biaryl
skeleton by chiral phosphoric acid catalysed desymmetrization/kinetic resolution sequence. _J. Am. Chem. Soc._ 135, 3964–3970 (2013). CAS PubMed Google Scholar * Mori, K., Itakura, T.
& Akiyama, T. Enantiodivergent atroposelective synthesis of chiral biaryls by asymmetric transfer hydrogenation: chiral phosphoric acid catalysed dynamic kinetic resolution. _Angew.
Chem. Int. Ed._ 55, 11642–11646 (2016). CAS Google Scholar * Li, G.-Q. et al. Organocatalytic aryl–aryl bond formation: an atroposelective [3, 3]-rearrangement approach to BINAM
derivatives. _J. Am. Chem. Soc._ 135, 7414–7417 (2013). CAS PubMed Google Scholar * De, C. K., Pesciaioli, F. & List, B. Catalytic asymmetric benzidine rearrangement. _Angew. Chem.
Int. Ed._ 52, 9293–9295 (2013). CAS Google Scholar * Zhang, H.-H. et al. Design and enantioselective construction of axially chiral naphthyl-indole skeletons. _Angew. Chem. Int. Ed._ 56,
116–121 (2017). CAS Google Scholar * Chen, Y.-H. et al. Atroposelective synthesis of axially chiral biaryldiols via organocatalytic arylation of 2-naphthols. _J. Am. Chem. Soc._ 137,
15062–15065 (2015). CAS PubMed Google Scholar * Wang, J.-Z. et al. Symmetry in cascade chirality-transfer processes: a catalytic atroposelective direct arylation approach to BINOL
derivatives. _J. Am. Chem. Soc._ 138, 5202–5205 (2016). CAS PubMed PubMed Central Google Scholar * Wang, J., Chen, M.-W., Ji, Y., Hu, S.-B. & Zhou, Y.-G. Kinetic resolution of
axially chiral 5- or 8-substituted quinolines via asymmetric transfer hydrogenation. _J. Am. Chem. Soc._ 138, 10413–10416 (2016). CAS PubMed Google Scholar * Cheng, X., Vellalath, S.,
Goddard, R. & List, B. Direct catalytic asymmetric synthesis of cyclic aminals from aldehydes. _J. Am. Chem. Soc._ 130, 15786–15787 (2008). CAS PubMed Google Scholar * Huang, D., Li,
X., Xu, F., Li, L. & Lin, X. Highly enantioselective synthesis of dihydroquinazolinones catalysed by SPINOL-phosphoric acids. _ACS Catal._ 3, 2244–2247 (2013). CAS Google Scholar *
Rowland, G. B. et al. Brønsted acid-catalyzed imine amidation. _J. Am. Chem. Soc._ 127, 15696–15697 (2005). CAS PubMed Google Scholar * Zhang, J.-W. et al. Discovery and enantiocontrol of
axially chiral urazoles via organocatalytic tyrosine click reaction. _Nat. Commun._ 7, 10677 (2016). ADS PubMed PubMed Central Google Scholar * Quinonero, O. et al. Combining
organocatalysis with central-to-axial chirality conversion: atroposelective Hantzsch-type synthesis of 4-arylpyridines. _Angew. Chem. Int. Ed._ 55, 1401–1405 (2016). CAS Google Scholar *
Raut, V. S. et al. Enantioselective syntheses of furan atropisomers by an oxidative central-to-axial chirality conversion strategy. _J. Am. Chem. Soc._ 139, 2140–2143 (2017). CAS PubMed
Google Scholar * Nakashima, D. & Yamamoto, H. Design of chiral N-triflyl phosphoramide as a strong chiral Brønsted acid and its application to asymmetric Diels–Alder reaction. _J. Am.
Chem. Soc._ 128, 9626–9627 (2006). CAS PubMed Google Scholar * Miyaura, N. & Suzuki, A. Palladium-catalysed cross-coupling reactions of organoboron compounds. _Chem. Rev._ 95,
2457–2483 (1995). CAS Google Scholar * Jun, C.-H. Transition metal-catalysed carbon-carbon bond activation. _Chem. Soc. Rev._ 33, 610–618 (2004). CAS PubMed Google Scholar * Chen, F.,
Wang, T. & Jiao, N. Recent advances in transition-metal-catalysed functionalization of unstrained carbon–carbon bonds. _Chem. Rev._ 114, 8613–8661 (2014). CAS PubMed Google Scholar *
Li, Z. et al. Metal-and oxidant-free synthesis of quinazolinones from β-ketoesters with o-aminobenzamides via phosphorous acid-catalysed cyclocondensation and selective C–C bond cleavage.
_J. Org. Chem._ 80, 9392–9400 (2015). CAS PubMed Google Scholar * Lu, S.-M. & Alper, H. Intramolecular carbonylation reactions with recyclable palladium-complexed dendrimers on
silica: synthesis of oxygen, nitrogen, or sulfur-containing medium ring fused heterocycles. _J. Am. Chem. Soc._ 127, 14776–14784 (2005). CAS PubMed Google Scholar * Quesnel, J. S. &
Arndtsen, B. A. A palladium-catalysed carbonylation approach to acid chloride synthesis. _J. Am. Chem. Soc._ 135, 16841–16844 (2013). CAS PubMed Google Scholar * Weitl, F. L. &
Raymond, K. N. Specific sequestering agents for the actinides. 3. Polycatecholate ligands derived from 2, 3-dihydroxy-5-sulfobenzoyl conjugates of diaza-and tetraazaalkanes. _J. Am. Chem.
Soc._ 102, 2289–2293 (1980). CAS Google Scholar Download references ACKNOWLEDGEMENTS We are thankful for the financial support from the National Natural Science Foundation of China (Nos.
21572095), Shenzhen special funds for the development of biomedicine, internet, new energy, and new material industries (JCYJ20150430160022510). B.T. thanks the Thousand Young Talents
Program for financial support. Dedicated to prof Scott J. Miller for his great contribution on construction of axially chiral compounds via peptide catalysis. AUTHOR INFORMATION AUTHORS AND
AFFILIATIONS * Department of Chemistry, South University of Science and Technology of China, Shenzhen, 518055, China Yong-Bin Wang, Sheng-Cai Zheng, Yu-Mei Hu & Bin Tan * Department of
Chemistry, Fudan University, 220 Handan Road, Shanghai, 200433, China Yong-Bin Wang Authors * Yong-Bin Wang View author publications You can also search for this author inPubMed Google
Scholar * Sheng-Cai Zheng View author publications You can also search for this author inPubMed Google Scholar * Yu-Mei Hu View author publications You can also search for this author
inPubMed Google Scholar * Bin Tan View author publications You can also search for this author inPubMed Google Scholar CONTRIBUTIONS Y.-B.W. performed experiments and prepared the
Supplementary Information. S.-C.Z. and Y.-M.H. helped with characterizing some new compounds. B.T. conceived and directed the project and wrote the paper. CORRESPONDING AUTHOR Correspondence
to Bin Tan. ETHICS DECLARATIONS COMPETING INTERESTS The authors declare no competing financial interests. SUPPLEMENTARY INFORMATION SUPPLEMENTARY INFORMATION Supplementary figures,
supplementary tables, supplementary notes and supplementary references. (PDF 21296 kb) PEER REVIEW FILE (PDF 77 KB) RIGHTS AND PERMISSIONS This work is licensed under a Creative Commons
Attribution 4.0 International License. The images or other third party material in this article are included in the article’s Creative Commons license, unless indicated otherwise in the
credit line; if the material is not included under the Creative Commons license, users will need to obtain permission from the license holder to reproduce the material. To view a copy of
this license, visit http://creativecommons.org/licenses/by/4.0/ Reprints and permissions ABOUT THIS ARTICLE CITE THIS ARTICLE Wang, YB., Zheng, SC., Hu, YM. _et al._ Brønsted acid-catalysed
enantioselective construction of axially chiral arylquinazolinones. _Nat Commun_ 8, 15489 (2017). https://doi.org/10.1038/ncomms15489 Download citation * Received: 11 February 2017 *
Accepted: 03 April 2017 * Published: 19 May 2017 * DOI: https://doi.org/10.1038/ncomms15489 SHARE THIS ARTICLE Anyone you share the following link with will be able to read this content: Get
shareable link Sorry, a shareable link is not currently available for this article. Copy to clipboard Provided by the Springer Nature SharedIt content-sharing initiative