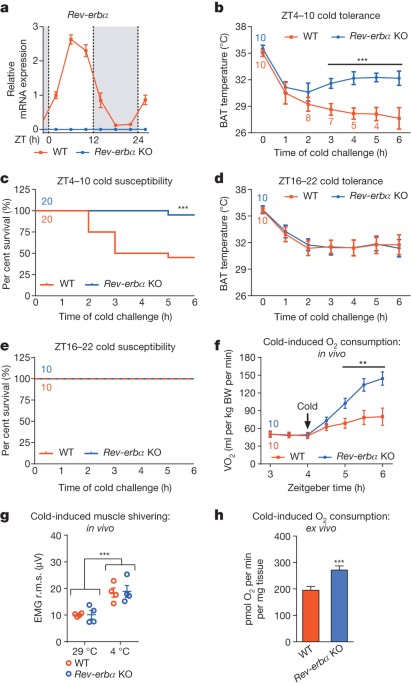
- Select a language for the TTS:
- UK English Female
- UK English Male
- US English Female
- US English Male
- Australian Female
- Australian Male
- Language selected: (auto detect) - EN
Play all audios:
ABSTRACT Circadian oscillation of body temperature is a basic, evolutionarily conserved feature of mammalian biology1. In addition, homeostatic pathways allow organisms to protect their core
temperatures in response to cold exposure2. However, the mechanism responsible for coordinating daily body temperature rhythm and adaptability to environmental challenges is unknown. Here
we show that the nuclear receptor Rev-erbα (also known as Nr1d1), a powerful transcriptional repressor, links circadian and thermogenic networks through the regulation of brown adipose
tissue (BAT) function. Mice exposed to cold fare considerably better at 05:00 (Zeitgeber time 22) when Rev-erbα is barely expressed than at 17:00 (Zeitgeber time 10) when Rev-erbα is
abundant. Deletion of _Rev-erbα_ markedly improves cold tolerance at 17:00, indicating that overcoming Rev-erbα-dependent repression is a fundamental feature of the thermogenic response to
cold. Physiological induction of uncoupling protein 1 (Ucp1) by cold temperatures is preceded by rapid downregulation of _Rev-erbα_ in BAT. Rev-erbα represses Ucp1 in a
brown-adipose-cell-autonomous manner and BAT Ucp1 levels are high in _Rev-erbα_-null mice, even at thermoneutrality. Genetic loss of _Rev-erbα_ also abolishes normal rhythms of body
temperature and BAT activity. Thus, Rev-erbα acts as a thermogenic focal point required for establishing and maintaining body temperature rhythm in a manner that is adaptable to
environmental demands. Access through your institution Buy or subscribe This is a preview of subscription content, access via your institution ACCESS OPTIONS Access through your institution
Subscribe to this journal Receive 51 print issues and online access $199.00 per year only $3.90 per issue Learn more Buy this article * Purchase on SpringerLink * Instant access to full
article PDF Buy now Prices may be subject to local taxes which are calculated during checkout ADDITIONAL ACCESS OPTIONS: * Log in * Learn about institutional subscriptions * Read our FAQs *
Contact customer support SIMILAR CONTENT BEING VIEWED BY OTHERS COLD-INDUCTION OF AFADIN IN BROWN FAT SUPPORTS ITS THERMOGENIC CAPACITY Article Open access 07 May 2021 ATF4 EXPRESSION IN
THERMOGENIC ADIPOCYTES IS REQUIRED FOR COLD-INDUCED THERMOGENESIS IN MICE VIA FGF21-INDEPENDENT MECHANISMS Article Open access 18 January 2024 NARDILYSIN IN ADIPOCYTES REGULATES UCP1
EXPRESSION AND BODY TEMPERATURE HOMEOSTASIS Article Open access 02 March 2022 REFERENCES * Bass, J. Circadian topology of metabolism. _Nature_ 491, 348–356 (2012) Article ADS CAS Google
Scholar * Cannon, B. & Nedergaard, J. Brown adipose tissue: function and physiological significance. _Physiol. Rev._ 84, 277–359 (2004) Article CAS Google Scholar * Takahashi, J. S.,
Hong, H.-K., Ko, C. H. & McDearmon, E. L. The genetics of mammalian circadian order and disorder: implications for physiology and disease. _Nature Rev. Genet._ 9, 764–775 (2008) Article
CAS Google Scholar * Sahar, S. & Sassone-Corsi, P. Metabolism and cancer: the circadian clock connection. _Nature Rev. Cancer_ 9, 886–896 (2009) Article CAS Google Scholar *
Asher, G. & Schibler, U. Crosstalk between components of circadian and metabolic cycles in mammals. _Cell Metab._ 13, 125–137 (2011) Article CAS Google Scholar * Bass, J. &
Takahashi, J. S. Circadian integration of metabolism and energetics. _Science_ 330, 1349–1354 (2010) Article ADS CAS Google Scholar * Feng, D. & Lazar, M. A. Clocks, metabolism, and
the epigenome. _Mol. Cell_ 47, 158–167 (2012) Article CAS Google Scholar * Buhr, E. D., Yoo, S.-H. & Takahashi, J. S. Temperature as a universal resetting cue for mammalian circadian
oscillators. _Science_ 330, 379–385 (2010) Article ADS CAS Google Scholar * Feng, D. et al. A circadian rhythm orchestrated by histone deacetylase 3 controls hepatic lipid metabolism.
_Science_ 331, 1315–1319 (2011) Article ADS CAS Google Scholar * Woldt, E. et al. Rev-erb-α modulates skeletal muscle oxidative capacity by regulating mitochondrial biogenesis and
autophagy. _Nature Med._ (2013) * Bugge, A. et al. Rev-erbα and Rev-erbβ coordinately protect the circadian clock and normal metabolic function. _Genes Dev._ 26, 657–667 (2012) Article CAS
Google Scholar * Cho, H. et al. Regulation of circadian behaviour and metabolism by Rev-erb-α and Rev-erb-β. _Nature_ 485, 123–127 (2012) Article ADS CAS Google Scholar * Delezie, J.
et al. The nuclear receptor Rev-erbα is required for the daily balance of carbohydrate and lipid metabolism. _FASEB J._ 26, 3321–3335 (2012) Article CAS Google Scholar * Le Martelot, G.
et al. Rev-erbα participates in circadian SREBP signaling and bile acid homeostasis. _PLoS Biol._ 7, e1000181 (2009) Article Google Scholar * Solt, L. A. et al. Regulation of circadian
behaviour and metabolism by synthetic Rev-erb agonists. _Nature_ 485, 62–68 (2012) Article ADS CAS Google Scholar * Cannon, B. & Nedergaard, J. Nonshivering thermogenesis and its
adequate measurement in metabolic studies. _J. Exp. Biol._ 214, 242–253 (2011) Article Google Scholar * Preitner, N. et al. The orphan nuclear receptor Rev-erbα controls circadian
transcription within the positive limb of the mammalian circadian oscillator. _Cell_ 110, 251–260 (2002) Article CAS Google Scholar * Lim, S. et al. Cold-induced activation of brown
adipose tissue and adipose angiogenesis in mice. _Nature Protocols_ 7, 606–615 (2012) Article CAS Google Scholar * Golozoubova, V. et al. Only UCP1 can mediate adaptive nonshivering
thermogenesis in the cold. _FASEB J._ 15, 2048–2050 (2001) Article CAS Google Scholar * Talan, M. I., Tatelman, H. M. & Engel, B. T. Cold tolerance and metabolic heat production in
male C57BL/6J mice at different times of day. _Physiol. Behav._ 50, 613–616 (1991) Article CAS Google Scholar * Puigserver, P. et al. A cold-inducible coactivator of nuclear receptors
linked to adaptive thermogenesis. _Cell_ 92, 829–839 (1998) Article CAS Google Scholar * Pearen, M. A. et al. The orphan nuclear receptor, NOR-1, is a target of β-adrenergic signaling in
skeletal muscle. _Endocrinology_ 147, 5217–5227 (2006) Article CAS Google Scholar * Cypess, A. M. et al. Cold but not sympathomimetics activates human brown adipose tissue _in vivo_.
_Proc. Natl Acad. Sci. USA_ 109, 10001–10005 (2012) Article ADS CAS Google Scholar * Vosselman, M. J. et al. Systemic β-adrenergic stimulation of thermogenesis is not accompanied by
brown adipose tissue activity in humans. _Diabetes_ 61, 3106–3113 (2012) Article CAS Google Scholar * Nedergaard, J. & Cannon, B. UCP1 mRNA does not produce heat. _Biochim. Biophys.
Acta_ 1831, 943–949 (2013) Article CAS Google Scholar * Cypess, A. M. et al. Identification and importance of brown adipose tissue in adult humans. _N. Engl. J. Med._ 360, 1509–1517
(2009) Article CAS Google Scholar * Van Marken Lichtenbelt, W. D. et al. Cold-activated brown adipose tissue in healthy men. _N. Engl. J. Med._ 360, 1500–1508 (2009) Article CAS Google
Scholar * Virtanen, K. A. et al. Functional brown adipose tissue in healthy adults. _N. Engl. J. Med._ 360, 1518–1525 (2009) Article CAS Google Scholar * Van der Veen, D. R., Shao, J.,
Chapman, S., Leevy, W. M. & Duffield, G. E. A diurnal rhythm in glucose uptake in brown adipose tissue revealed by _in vivo_ PET-FDG imaging. _Obesity_ 20, 1527–1529 (2012) Article CAS
Google Scholar * Saito, S., Saito, C. T. & Shingai, R. Adaptive evolution of the uncoupling protein 1 gene contributed to the acquisition of novel nonshivering thermogenesis in
ancestral eutherian mammals. _Gene_ 408, 37–44 (2008) Article CAS Google Scholar Download references ACKNOWLEDGEMENTS We thank the Functional Genomics Core (J. Schug) and the Mouse
Phenotyping, Physiology, and Metabolism Core (R. Ahima and R. Dhir) of the Penn Diabetes Research Center (NIH P30 DK19525). We also thank the Small Animal Imaging Facility of the Perelman
School of Medicine at the University of Pennsylvania (E. Blankemeyer). This work was supported by NIH grants R01 DK45586 (M.A.L.) and F-32 DK095563 (Z.G.-H.) and the JPB Foundation. A.B. was
funded by the Novo Nordisk STAR postdoctoral program. AUTHOR INFORMATION Author notes * Dan Feng and Matthew J. Emmett: These authors contributed equally to this work. AUTHORS AND
AFFILIATIONS * Division of Endocrinology, Department of Medicine, Department of Genetics, Diabetes, and Metabolism, Perelman School of Medicine at the University of Pennsylvania,
Philadelphia, 19104, Pennsylvania, USA Zachary Gerhart-Hines, Dan Feng, Matthew J. Emmett, Logan J. Everett, Erika R. Briggs, Anne Bugge & Mitchell A. Lazar * The Institute for Diabetes,
Obesity, and Metabolism, Perelman School of Medicine at the University of Pennsylvania, Philadelphia, 19104, Pennsylvania, USA Zachary Gerhart-Hines, Dan Feng, Matthew J. Emmett, Logan J.
Everett, Erika R. Briggs, Anne Bugge, Patrick Seale & Mitchell A. Lazar * Department of Physiology, Perelman School of Medicine at the University of Pennsylvania, Philadelphia, 19104,
Pennsylvania, USA Emanuele Loro & Tejvir S. Khurana * Pennsylvania Muscle Institute, Perelman School of Medicine at the University of Pennsylvania, Philadelphia, 19104, Pennsylvania, USA
Emanuele Loro & Tejvir S. Khurana * Department of Radiology, Perelman School of Medicine at the University of Pennsylvania, Philadelphia, 19104, Pennsylvania, USA Catherine Hou &
Daniel A. Pryma * Department of Pediatrics, Children’s Hospital of Philadelphia, Philadelphia, 19104, Pennsylvania, USA Christine Ferrara * Department of Cell and Developmental Biology,
Perelman School of Medicine at the University of Pennsylvania, Philadelphia, 19104, Pennsylvania, USA Patrick Seale Authors * Zachary Gerhart-Hines View author publications You can also
search for this author inPubMed Google Scholar * Dan Feng View author publications You can also search for this author inPubMed Google Scholar * Matthew J. Emmett View author publications
You can also search for this author inPubMed Google Scholar * Logan J. Everett View author publications You can also search for this author inPubMed Google Scholar * Emanuele Loro View
author publications You can also search for this author inPubMed Google Scholar * Erika R. Briggs View author publications You can also search for this author inPubMed Google Scholar * Anne
Bugge View author publications You can also search for this author inPubMed Google Scholar * Catherine Hou View author publications You can also search for this author inPubMed Google
Scholar * Christine Ferrara View author publications You can also search for this author inPubMed Google Scholar * Patrick Seale View author publications You can also search for this author
inPubMed Google Scholar * Daniel A. Pryma View author publications You can also search for this author inPubMed Google Scholar * Tejvir S. Khurana View author publications You can also
search for this author inPubMed Google Scholar * Mitchell A. Lazar View author publications You can also search for this author inPubMed Google Scholar CONTRIBUTIONS D.F., M.J.E., L.J.E.,
E.R.B., A.B. and C.F. performed key experiments/data analysis and read the manuscript. P.S. provided advice and read the manuscript. E.L. and T.S.K. designed, performed and analysed EMG
studies and read the manuscript. C.H. and D.A.P. designed, performed and analysed 18FDG scans and read the manuscript. Z.G.H. performed many of the experiments, and Z.G.H. and M.A.L.
conceived the project, designed experiments, analysed all results and wrote the manuscript. CORRESPONDING AUTHOR Correspondence to Mitchell A. Lazar. ETHICS DECLARATIONS COMPETING INTERESTS
The authors declare no competing financial interests. EXTENDED DATA FIGURES AND TABLES EXTENDED DATA FIGURE 1 THE BAT CORE CLOCK IS LARGELY UNAFFECTED BY _REV-ERBΑ_ DELETION. A, Rev-erbα
protein levels in BAT of wild-type and _Rev-erbα_ knockout mice (_n_ = 2; each lane of the western blot represents pooled biological duplicates). B, BAT mRNA for indicated genes from
wild-type and _Rev-erbα_ knockout mice collected at the indicated times over a 24-h time course (_n_ = 3). EXTENDED DATA FIGURE 2 REV-ERBΑ CONTROLS COLD AND NORADRENALINE-INDUCED OXIDATIVE
METABOLISM INDEPENDENTLY OF SKELETAL MUSCLE METABOLISM. A, Food intake from cold-challenged _Rev-erbα_ knockout mice and control littermates in Fig. 1f. B, r.m.s. derivation of EMG
measurement from Fig. 1g. C, Oxygen consumption rates of _Rev-erbα_ KO mice and control littermates following noradrenaline administration (1 mg kg−1 s.c.) (_n_ = 6). D, E, r.m.s. derivation
of EMG measurements performed on wild-type and _Rev-erbα_ knockout mice following noradrenaline administration (1 mg kg−1 s.c.) (_n_ = 4). ***_P_ < 0.001 as determined by Student’s
_t_-test. Data are expressed as mean ± s.d. EXTENDED DATA FIGURE 3 _REV-ERBΑ_, BUT NOT _REV-ERBΒ_, IS DECREASED IN A COLD-DEPENDENT MANNER. A–C, _Rev-erbβ_ (A), _Bmal1_ (B) and _Pgc1a_ (C)
mRNA levels in BAT during a cold-exposure time course (_n_ = 3 for mRNA). D, BAT gene expression following moderate (20 °C) or acute (4 °C) cold challenges (_n_ = 3). E, BAT protein levels
after 3 h noradrenaline administration (1 mg kg−1 i.p.) or cold exposure (_n_ = 3). *_P_ < 0.05, **_P_ < 0.01, ***_P_ < 0.001 as determined by one-way ANOVA with multiple
comparisons and a Tukey’s post-test. Data are expressed as mean ± s.d. EXTENDED DATA FIGURE 4 REV-ERBΑ NEGATIVELY REGULATES _UCP1_. A, B, BAT mRNA (A) and protein (B) from wild-type and
_Rev-erbα_ knockout mice exposed to cold for 6 h as described in Fig. 3a, b. C, mRNA levels in preadipocytes isolated from wild-type mice, differentiated in culture and collected at the
indicated times after synchronization by serum shock (_n_ = 4). **_P_ < 0.01, ***_P_ < 0.001 as determined by one-way ANOVA with multiple comparisons and a Tukey’s post-test. Data are
expressed as mean ± s.d. EXTENDED DATA FIGURE 5 REV-ERBΑ CONTROLS CIRCADIAN OSCILLATION OF SURFACE TEMPERATURE AND BAT ACTIVITY. A, Infrared images from the thermographic surface temperature
analysis performed in Fig. 4c. B, Genotypic differences between BAT and core temperatures from wild-type and _Rev-erbα_ knockout mice acclimated to thermoneutrality (_n_ = 6). C, 18FDG
imaging (_n_ = 4) of _Rev-erbα_ knockout mice and wild-type littermates during the light and dark phases. Representative sagittal planes are shown for each group. *_P_ < 0.05, Δcore
temperature versus ΔBAT temperature; †_P_ < 0.05, core temperature versus _Rev-erbα_ knockout core temperature; ‡_P_ < 0.001, wild-type BAT temperature versus _Rev-erbα_ knockout BAT
temperature as determined by Student’s _t_-test. Data are expressed as mean ± s.e.m. EXTENDED DATA FIGURE 6 THE NUCLEAR RECEPTOR REV-ERBΑ CONTROLS CIRCADIAN THERMOGENIC PLASTICITY. Rev-erbα
regulates the circadian rhythm of body temperature through direct suppression of thermogenesis and BAT activity. Cold exposure during the light phase rapidly overrides Rev-erbα-dependent
repression to induce thermogenic programs. POWERPOINT SLIDES POWERPOINT SLIDE FOR FIG. 1 POWERPOINT SLIDE FOR FIG. 2 POWERPOINT SLIDE FOR FIG. 3 POWERPOINT SLIDE FOR FIG. 4 RIGHTS AND
PERMISSIONS Reprints and permissions ABOUT THIS ARTICLE CITE THIS ARTICLE Gerhart-Hines, Z., Feng, D., Emmett, M. _et al._ The nuclear receptor Rev-erbα controls circadian thermogenic
plasticity. _Nature_ 503, 410–413 (2013). https://doi.org/10.1038/nature12642 Download citation * Received: 15 March 2013 * Accepted: 09 September 2013 * Published: 27 October 2013 * Issue
Date: 21 November 2013 * DOI: https://doi.org/10.1038/nature12642 SHARE THIS ARTICLE Anyone you share the following link with will be able to read this content: Get shareable link Sorry, a
shareable link is not currently available for this article. Copy to clipboard Provided by the Springer Nature SharedIt content-sharing initiative