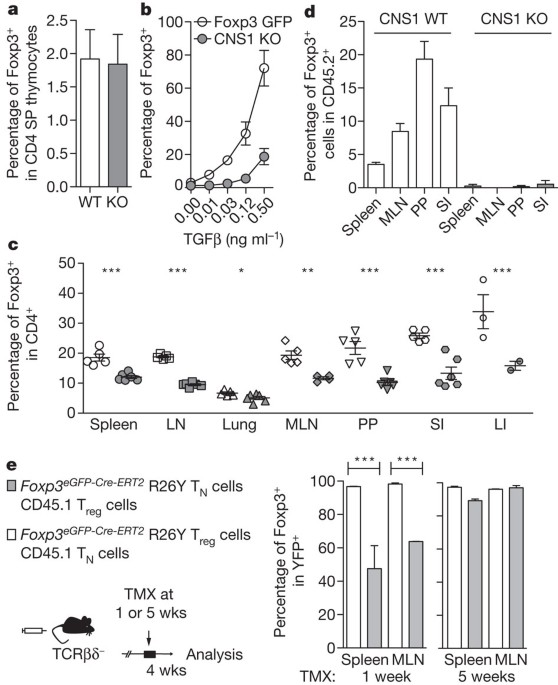
- Select a language for the TTS:
- UK English Female
- UK English Male
- US English Female
- US English Male
- Australian Female
- Australian Male
- Language selected: (auto detect) - EN
Play all audios:
ABSTRACT A balance between pro- and anti-inflammatory mechanisms at mucosal interfaces, which are sites of constitutive exposure to microbes and non-microbial foreign substances, allows for
efficient protection against pathogens yet prevents adverse inflammatory responses associated with allergy, asthma and intestinal inflammation1. Regulatory T (Treg) cells prevent systemic
and tissue-specific autoimmunity and inflammatory lesions at mucosal interfaces. These cells are generated in the thymus (tTreg cells) and in the periphery (induced (i)Treg cells), and their
dual origin implies a division of labour between tTreg and iTreg cells in immune homeostasis. Here we show that a highly selective blockage in differentiation of iTreg cells in mice did not
lead to unprovoked multi-organ autoimmunity, exacerbation of induced tissue-specific autoimmune pathology, or increased pro-inflammatory responses of T helper 1 (TH1) and TH17 cells.
However, mice deficient in iTreg cells spontaneously developed pronounced TH2-type pathologies at mucosal sites—in the gastrointestinal tract and lungs—with hallmarks of allergic
inflammation and asthma. Furthermore, iTreg-cell deficiency altered gut microbial communities. These results suggest that whereas Treg cells generated in the thymus appear sufficient for
control of systemic and tissue-specific autoimmunity, extrathymic differentiation of Treg cells affects commensal microbiota composition and serves a distinct, essential function in
restraint of allergic-type inflammation at mucosal interfaces. Access through your institution Buy or subscribe This is a preview of subscription content, access via your institution ACCESS
OPTIONS Access through your institution Subscribe to this journal Receive 51 print issues and online access $199.00 per year only $3.90 per issue Learn more Buy this article * Purchase on
SpringerLink * Instant access to full article PDF Buy now Prices may be subject to local taxes which are calculated during checkout ADDITIONAL ACCESS OPTIONS: * Log in * Learn about
institutional subscriptions * Read our FAQs * Contact customer support SIMILAR CONTENT BEING VIEWED BY OTHERS SELECTIVE IL-27 PRODUCTION BY INTESTINAL REGULATORY T CELLS PERMITS GUT-SPECIFIC
REGULATION OF TH17 CELL IMMUNITY Article 06 November 2023 MUCOSAL TISSUE REGULATORY T CELLS ARE INTEGRAL IN BALANCING IMMUNITY AND TOLERANCE AT PORTALS OF ANTIGEN ENTRY Article 29 November
2021 NOVEL ANTIGEN-PRESENTING CELL IMPARTS TREG-DEPENDENT TOLERANCE TO GUT MICROBIOTA Article Open access 07 September 2022 REFERENCES * Maloy, K. J. & Powrie, F. Intestinal homeostasis
and its breakdown in inflammatory bowel disease. _Nature_ 474, 298–306 (2011) Article ADS CAS Google Scholar * Sakaguchi, S., Yamaguchi, T., Nomura, T. & Ono, M. Regulatory T cells
and immune tolerance. _Cell_ 133, 775–787 (2008) Article CAS Google Scholar * Chen, W. et al. Conversion of peripheral CD4+CD25− naive T cells to CD4+CD25+ regulatory T cells by TGF-β
induction of transcription factor Foxp3. _J. Exp. Med._ 198, 1875–1886 (2003) Article CAS Google Scholar * Tone, Y. et al. Smad3 and NFAT cooperate to induce Foxp3 expression through its
enhancer. _Nature Immunol._ 9, 194–202 (2008) Article CAS Google Scholar * Zheng, Y. et al. Role of conserved non-coding DNA elements in the _Foxp3_ gene in regulatory T-cell fate.
_Nature_ 463, 808–812 (2010) Article ADS CAS Google Scholar * Hill, J. A. et al. Retinoic acid enhances Foxp3 induction indirectly by relieving inhibition from CD4+CD44hi cells.
_Immunity_ 29, 758–770 (2008) Article CAS Google Scholar * Nolting, J. et al. Retinoic acid can enhance conversion of naive into regulatory T cells independently of secreted cytokines.
_J. Exp. Med._ 206, 2131–2139 (2009) Article CAS Google Scholar * Xu, L. et al. Positive and negative transcriptional regulation of the _Foxp3_ gene is mediated by access and binding of
the Smad3 protein to enhancer I. _Immunity_ 33, 313–325 (2010) Article CAS Google Scholar * Liu, Y. et al. A critical function for TGF-β signaling in the development of natural
CD4+CD25+Foxp3+ regulatory T cells. _Nature Immunol._ 9, 632–640 (2008) Article ADS CAS Google Scholar * Ouyang, W., Beckett, O., Ma, Q. & Li, M. O. Transforming growth factor-β
signaling curbs thymic negative selection promoting regulatory T cell development. _Immunity_ 32, 642–653 (2010) Article CAS Google Scholar * Curotto de Lafaille, M. A. & Lafaille, J.
J. Natural and adaptive foxp3+ regulatory T cells: more of the same or a division of labor? _Immunity_ 30, 626–635 (2009) Article CAS Google Scholar * Mucida, D. et al. Oral tolerance in
the absence of naturally occurring Tregs. _J. Clin. Invest._ 115, 1923–1933 (2005) Article CAS Google Scholar * Bautista, J. L. et al. Intraclonal competition limits the fate
determination of regulatory T cells in the thymus. _Nature Immunol._ 10, 610–617 (2009) Article CAS Google Scholar * Leung, M. W., Shen, S. & Lafaille, J. J. TCR-dependent
differentiation of thymic Foxp3+ cells is limited to small clonal sizes. _J. Exp. Med._ 206, 2121–2130 (2009) Article CAS Google Scholar * Moran, A. E. et al. T cell receptor signal
strength in Treg and iNKT cell development demonstrated by a novel fluorescent reporter mouse. _J. Exp. Med._ 208, 1279–1289 (2011) Article CAS Google Scholar * Lathrop, S. K. et al.
Peripheral education of the immune system by colonic commensal microbiota. _Nature_ 478, 250–254 (2011) Article ADS CAS Google Scholar * Komatsu, N. et al. Heterogeneity of natural
Foxp3+ T cells: a committed regulatory T-cell lineage and an uncommitted minor population retaining plasticity. _Proc. Natl Acad. Sci. USA_ 106, 1903–1908 (2009) Article ADS CAS Google
Scholar * Hori, S. Regulatory T cell plasticity: beyond the controversies. _Trends Immunol._ 32, 295–300 (2011) Article CAS Google Scholar * Zhou, X. et al. Instability of the
transcription factor Foxp3 leads to the generation of pathogenic memory T cells _in vivo_. _Nature Immunol._ 10, 1000–1007 (2009) Article CAS Google Scholar * Rubtsov, Y. P. et al.
Stability of the regulatory T cell lineage _in vivo_. _Science_ 329, 1667–1671 (2010) Article ADS CAS Google Scholar * Josefowicz, S. Z. & Rudensky, A. Control of regulatory T cell
lineage commitment and maintenance. _Immunity_ 30, 616–625 (2009) Article CAS Google Scholar * Gottschalk, R. A., Corse, E. & Allison, J. P. TCR ligand density and affinity determine
peripheral induction of Foxp3 _in vivo_. _J. Exp. Med._ 207, 1701–1711 (2010) Article CAS Google Scholar * Kretschmer, K. et al. Inducing and expanding regulatory T cell populations by
foreign antigen. _Nature Immunol._ 6, 1219–1227 (2005) Article CAS Google Scholar * Tian, L. et al. Foxp3 regulatory T cells exert asymmetric control over murine helper responses by
inducing Th2 cell apoptosis. _Blood_ 118, 1845–1853 (2011) Article CAS Google Scholar * Chaudhry, A. et al. CD4+ regulatory T cells control TH17 responses in a Stat3-dependent manner.
_Science_ 326, 986–991 (2009) Article ADS CAS Google Scholar * Koch, M. A. et al. The transcription factor T-bet controls regulatory T cell homeostasis and function during type 1
inflammation. _Nature Immunol._ 10, 595–602 (2009) Article CAS Google Scholar * Zheng, Y. et al. Regulatory T-cell suppressor program co-opts transcription factor IRF4 to control TH2
responses. _Nature_ 458, 351–356 (2009) Article ADS CAS Google Scholar * Wang, Y., Su, M. A. & Wan, Y. Y. An essential role of the transcription factor GATA-3 for the function of
regulatory T cells. _Immunity_ 35, 337–348 (2011) Article CAS Google Scholar * Wohlfert, E. A. et al. GATA3 controls Foxp3+ regulatory T cell fate during inflammation in mice. _J. Clin.
Invest._ 121, 4503–4515 (2011) Article CAS Google Scholar * Turnbaugh, P. J. et al. An obesity-associated gut microbiome with increased capacity for energy harvest. _Nature_ 444,
1027–1031 (2006) Article ADS Google Scholar * Stromnes, I. M. & Goverman, J. M. Active induction of experimental allergic encephalomyelitis. _Nature Protocols_ 1, 1810–1819 (2006)
Article CAS Google Scholar * Burich, A. et al. Helicobacter-induced inflammatory bowel disease in IL-10- and T cell-deficient mice. _Am. J. Physiol. Gastrointest. Liver Physiol._ 281,
G764–G778 (2001) Article CAS Google Scholar * Ubeda, C. et al. Vancomycin-resistant Enterococcus domination of intestinal microbiota is enabled by antibiotic treatment in mice and
precedes bloodstream invasion in humans. _J. Clin. Invest._ 120, 4332–4341 (2010) Article CAS Google Scholar * Nossa, C. W. et al. Design of 16S rRNA gene primers for 454 pyrosequencing
of the human foregut microbiome. _World J. Gastroenterol._ 16, 4135–4144 (2010) Article CAS Google Scholar * Schloss, P. D. et al. Introducing mothur: open-source, platform-independent,
community-supported software for describing and comparing microbial communities. _Appl. Environ. Microbiol._ 75, 7537–7541 (2009) Article CAS Google Scholar * Edgar, R. C. et al. UCHIME
improves sensitivity and speed of chimera detection. _Bioinformatics_ 27, 2194–2200 (2011) Article CAS Google Scholar * Magurran, A. E. _Measuring Biological Diversity_ (Blackwell, 2004)
Google Scholar * Wang, Q. et al. Naive Bayesian classifier for rapid assignment of rRNA sequences into the new bacterial taxonomy. _Appl. Environ. Microbiol._ 73, 5261–5267 (2007) Article
CAS Google Scholar * Anders, S. & Huber, W. Differential expression analysis for sequence count data. _Genome Biol._ 11, R106 (2010) Article CAS Google Scholar Download references
ACKNOWLEDGEMENTS We thank T. Tedder for depleting CD20 antibody, R. Tudor for assistance interpreting lung pathology, P. DeRoos for assistance with Ig ELISA assays, B. Johnson for
immunohistochemical expertise, Y. Chen for assistance with airway measurements, and E. Pamer, L. Lipuma, A. Gobourne and R. Khanin for help with analysis of intestinal microbiota. This work
was supported by NIH MSTP grant GM07739 and NINDS grant 1F31NS073203-01 (R.E.N.), Strategic Young Researcher Overseas Visits Program for Accelerating Brain Circulation from Department of
Microbiology and Immunology, Keio University School of Medicine (T.C.) and NIH grant R37 AI034206 (A.Y.R.). A.Y.R is an investigator with the Howard Hughes Medical Institute. AUTHOR
INFORMATION Author notes * Steven Z. Josefowicz and Rachel E. Niec: These authors contributed equally to this work. AUTHORS AND AFFILIATIONS * Howard Hughes Medical Institute and Immunology
Program, Sloan Kettering Institute, New York, 10021, New York, USA Steven Z. Josefowicz, Rachel E. Niec, Takatoshi Chinen & Alexander Y. Rudensky * Laboratory of Chromatin Biology and
Epigenetics, The Rockefeller University, New York, 10065, New York, USA Steven Z. Josefowicz * Division of Immunology, Children’s Hospital, Harvard Medical School, Boston, 02115,
Massachusetts, USA Hye Young Kim & Dale T. Umetsu * Department of Comparative Medicine, and Histology and Imaging Core, School of Medicine, University of Washington, Seattle, 98195,
Washington, USA Piper Treuting * Department of Microbiology and Immunology, Keio University School of Medicine, Tokyo 160-8582, Japan, Takatoshi Chinen * Nomis Foundation Laboratories for
Immunobiology and Microbial Pathogenesis, Salk Institute for Biological Studies, La Jolla, 92037, California, USA Ye Zheng Authors * Steven Z. Josefowicz View author publications You can
also search for this author inPubMed Google Scholar * Rachel E. Niec View author publications You can also search for this author inPubMed Google Scholar * Hye Young Kim View author
publications You can also search for this author inPubMed Google Scholar * Piper Treuting View author publications You can also search for this author inPubMed Google Scholar * Takatoshi
Chinen View author publications You can also search for this author inPubMed Google Scholar * Ye Zheng View author publications You can also search for this author inPubMed Google Scholar *
Dale T. Umetsu View author publications You can also search for this author inPubMed Google Scholar * Alexander Y. Rudensky View author publications You can also search for this author
inPubMed Google Scholar CONTRIBUTIONS S.Z.J., R.E.N. and H.Y.K. performed experiments and analysed data, with assistance from T.C. for tissue Ig ELISA experiments, and P.T. for
immunohistochemistry and histopathology analysis. D.T.U., S.Z.J., R.E.N., H.Y.K, and A.Y.R designed and interpreted AHR experiments. Y.Z. generated CNS1- mice. S.Z.J., R.E.N. and A.Y.R.
designed experiments and wrote the paper. CORRESPONDING AUTHOR Correspondence to Alexander Y. Rudensky. ETHICS DECLARATIONS COMPETING INTERESTS The authors declare no competing financial
interests. SUPPLEMENTARY INFORMATION SUPPLEMENTARY FIGURES This file contains Supplementary Figures 1-21 with legends. (PDF 5434 kb) POWERPOINT SLIDES POWERPOINT SLIDE FOR FIG. 1 POWERPOINT
SLIDE FOR FIG. 2 POWERPOINT SLIDE FOR FIG. 3 POWERPOINT SLIDE FOR FIG. 4 RIGHTS AND PERMISSIONS Reprints and permissions ABOUT THIS ARTICLE CITE THIS ARTICLE Josefowicz, S., Niec, R., Kim,
H. _et al._ Extrathymically generated regulatory T cells control mucosal TH2 inflammation. _Nature_ 482, 395–399 (2012). https://doi.org/10.1038/nature10772 Download citation * Received: 06
July 2011 * Accepted: 06 December 2011 * Published: 08 February 2012 * Issue Date: 16 February 2012 * DOI: https://doi.org/10.1038/nature10772 SHARE THIS ARTICLE Anyone you share the
following link with will be able to read this content: Get shareable link Sorry, a shareable link is not currently available for this article. Copy to clipboard Provided by the Springer
Nature SharedIt content-sharing initiative