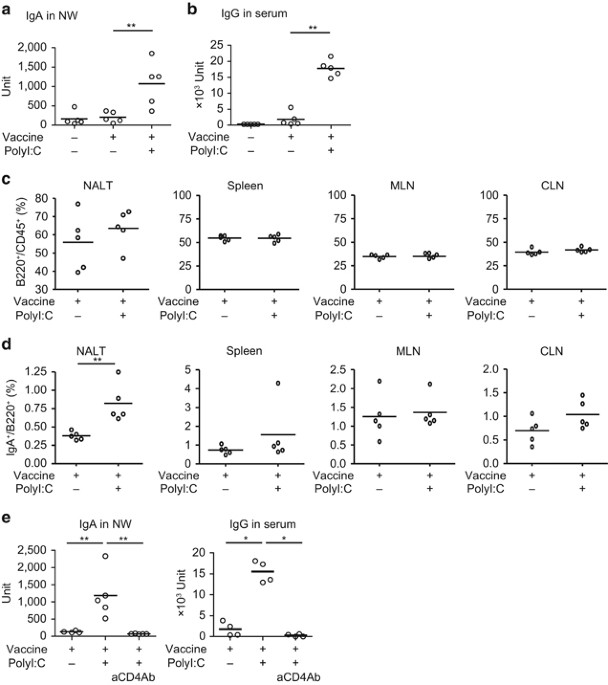
- Select a language for the TTS:
- UK English Female
- UK English Male
- US English Female
- US English Male
- Australian Female
- Australian Male
- Language selected: (auto detect) - EN
Play all audios:
ABSTRACT Intranasal inoculation with influenza hemagglutinin subunit with polyinosine-polycytidylic (polyI:C), a synthetic analog for double-stranded RNA, enhances production of
vaccine-specific immunoglobulin (Ig) A, which is superior to IgG in prophylactic immunity. The mechanism whereby polyI:C skews to IgA production in the nasal-associated lymph tissue (NALT)
was investigated in mouse models. Nasally instilled polyI:C was endocytosed into CD103+ dendritic cells (DCs) and induced T-cell activation, including interferon (IFN)-γ production.
According to knockout mouse studies, polyI:C activated the Toll-like receptor 3 signal via the adapter TICAM-1 (also called TRIF), that mainly caused T-cell-dependent IgA production. Nasal
CD103+ DCs activated transforming growth factor-β signaling and activation-induced cytidine deaminase upon polyI:C stimulation. IgA rather than IgG production was impaired in _Batf3_−/−
mice, where CD103+ DCs are defective. Genomic recombination occurred in IgA-producing cells in association with polyI:C-stimulated DCs and nasal microenvironment. PolyI:C induced
B-cell-activating factor expression and weakly triggered T-cell-independent IgA production. PolyI:C simultaneously activated mitochondrial antiviral signaling and then type I IFN receptor
pathways, which only minimally participated in IgA production. Taken together, CD103+ DCs in NALT are indispensable for the adjuvant activity of polyI:C in enhancing vaccine-specific IgA
induction and protective immunity against influenza viruses. SIMILAR CONTENT BEING VIEWED BY OTHERS ADP-RIBOSYLATING ADJUVANT REVEALS PLASTICITY IN CDC1 CELLS THAT DRIVE MUCOSAL TH17 CELL
DEVELOPMENT AND PROTECTION AGAINST INFLUENZA VIRUS INFECTION Article Open access 13 April 2022 AN IL-17-EGFR-TRAF4 AXIS CONTRIBUTES TO THE ALLEVIATION OF LUNG INFLAMMATION IN SEVERE
INFLUENZA Article Open access 03 June 2023 ILC2-DERIVED LIF LICENCES PROGRESS FROM TISSUE TO SYSTEMIC IMMUNITY Article Open access 07 August 2024 INTRODUCTION Influenza is an uncontrolled
epidemic infectious disease caused by influenza virus that occurs worldwide every year. The inactivated split-vaccine against influenza virus has been administered subcutaneously to elicit
anti-hemagglutinin (HA)-specific immunoglobulin (Ig) G in serum, while the cold-adapted live influenza vaccine has been administered via intranasal (i.n.) inoculation to induce
anti-HA-specific secretory immunoglobulin A (sIgA) in the nasal mucosa and upper respiratory tract.1 Although antigen (Ag)-specific IgG induced by vaccination is effective against homologous
virus, it poorly crossreacts with heterogeneous strains. In contrast, sIgA induced by i.n. vaccination efficiently crossreacts with off-target strains.1 Thus sIgA induction is important in
eliciting high levels of protection against different strains of influenza virus. Strategies to produce sIgA with adjuvant would be required for prophylactic vaccination against influenza.
Polyinosine-polycytidylic (polyI:C) and polyI:polyC12U, synthetic analogs of double-stranded RNA, act as adjuvants to effectively induce Ag-specific sIgA production in the nasal cavity when
they are administrated via i.n. inoculation with a split-product HA vaccine.2, 3 PolyI:C is recognized by endosomal Toll-like receptor 3 (TLR3) and cytosolic retinoic acid-inducible
gene-I-like receptors, including melanoma differentiation-associated protein 5 and retinoic acid-inducible gene-I, to signal via Toll/IL-1β receptor homology domain-containing adaptor
molecule (TICAM)-1 and mitochondrial antiviral signaling protein (MAVS), respectively.4, 5 The activation of these signaling pathways induces production of type I interferon (IFN) and
inflammatory cytokines, leading to the activation of host immunity, including Ig production.6 Nasal vaccination of HA would provoke IgA class switch in B cells through unknown events
involving innate adjuvanticity of polyI:C. IgA class switching recombination (CSR) is initiated by the expression of α germline transcripts and requires activation-induced cytidine deaminase
(AID) and categorized into T-cell-dependent (TD) and independent (TI) pathways.7 The TD pathway requires cytokine (transforming growth factor (TGF)-β, interleukin (IL)-4, IL-5 and IL-6)
signaling and binding of CD40 on B cells to its ligand CD40L on T cells.7, 8 The TI pathway is activated by B-cell-activating factor (BAFF), a proliferation-inducing ligand (APRIL),9 and TLR
ligands instead of CD40 signaling. BAFF, APRIL, and the TLR4 ligand lipopolysaccaride induce nuclear factor (NF)-κB activation to express AID,7, 10 which is an initiation protein for Ig
class switching in B cells.11 Mucosal dendritic cells (DCs) have a pivotal role for the induction of TI and TD IgA production. In the gastrointestinal tract, tumor necrosis factor-α and
inducible nitric oxide synthase double-positive DCs and plasmacytoid DCs highly express BAFF and APRIL and regulate IgA production.12, 13 TLR5-expressing CD103+CD11b+ DCs sense commensal
bacteria and express retinaldehyde dehydrogenase 2, resulting in the induction of IgA+ plasma cells.14 In lung tissue, retinaldehyde dehydrogenase 2-expressing CD103+CD11b− and
CD103−CD11b+CD24+ DCs induce IgA CSR in a retinoic acid and TGF-β-dependent manner.15 Compared with gut-associated lymph tissue and lung tissue, IgA-producing mechanisms and DC subsets
involved in IgA induction have been poorly investigated in oral and nasal mucosa. The nasal-associated lymphoid tissue (NALT), located in the i.n. cavity, is an inductive site for mucosal
immunity in the upper respiratory tract. Multiple immune cells, including T cells, B cells, macrophages, and DCs, exist in the NALT and elicit immune responses against not only invading
microbes but also vaccines.16, 17, 18 Although i.n. inoculation of polyI:C induces the expression of cytokines in the respiratory tract,19 it is unclear what immune cells are activated in
NALT in response to i.n. inoculation of polyI:C to elicit effective vaccination. In this study, we aimed at elucidating how polyI:C enhances vaccine-specific IgA production in the nasal
cavity and the role of CD103+ DCs, T cells and B cells in IgA CSR in NALT. RESULTS POLYI:C ENHANCES VACCINE-SPECIFIC IGA PRODUCTION IN NALT THROUGH THE TICAM-1 PATHWAY We first tested
whether i.n. inoculation of polyI:C promoted IgA production by vaccination. Wild-type (WT) C57B6/J mice were immunized via i.n. inoculation with A/California/07/2009 (H1N1) split-vaccine
with or without polyI:C. Two weeks after the second vaccination, we harvested nasal wash (NW) and serum from the mice. The levels of anti-HA IgA in NW and anti-HA IgG in serum were measured
by enzyme-linked immunosorbent assay (ELISA). Although vaccine treatment alone did not induce IgA in NW, vaccine treatment with polyI:C significantly enhanced IgA production (Figure 1a). IgG
production in serum was modestly induced by vaccine treatment alone and significantly enhanced by polyI:C (Figure 1b). Thus polyI:C mostly governs IgA production in the nasal mucosa and
largely affects IgG production. We examined the proportion of IgA+ B cells in lymph node (LN) after vaccination (Figure 1c,d and Supplementary Figure S1a online). Inoculation of polyI:C
barely brought increases of B220+ cells to the spleen, LNs, and NALT (Figure 1c and Supplementary Figure S1b). However, the proportion of IgA+ B220+ cells was increased specifically in NALT
after polyI:C inoculation (Figure 1d). IgA+ B cells actually accumulated in NALT prepared from vaccinated WT mice after vaccination with polyI:C (see Supplementary Figure S1c). The
proportion of IgA+ B220+ cells in the spleen, mesenteric LN, and cervical LN was unchanged by polyI:C treatment (Figure 1c,d). Total numbers of B220+ B cells were similar in NALT
irrespective of polyI:C inoculation (see Supplementary Figure S1b). PolyI:C-enhanced Ig production was abrogated in CD4+ T-depleted mice (Figure 1e), suggesting that CD4+ T-cell help is
crucial for the enhancement of Ig production by polyI:C. Next, to clarify the signaling pathway responsible for the adjuvanticity of polyI:C, we immunized vaccine to _Ticam1_−/− and
_Mavs_−/− mice with or without i.n. inoculation of polyI:C. _Ticam1_−/− mice abolished polyI:C-dependent IgA production in comparison with WT or _Mavs_−/− mice (Figure 2a,b). In parallel,
the numbers of IgA+ B cells in NALT from _Ticam1_−/− mice vaccinated with polyI:C was decreased in contrast to those in WT mice (see Supplementary Figure S1c). The total IgA+ B cells and
B220+ B cells were unchanged by _Ticam1_−/− in mice (see Supplementary Figure S1c,d). Similar but less prominent tendencies were observed in IgG production in _Ticam1_−/− mice (Figure 2b).
The polyI:C-augmented production of IgA and IgG was impaired in _Tlr3_−/− mice (Figure 2c). In contrast, _Mavs_ disruption did not affect the production of IgA and IgG (Figure 2a,b).
Although the type I IFN induction largely covers adjuvanticity of polyI:C as reported,6, 20 _Ifnar_−/− mice, where the type I IFN receptor (IFNAR) is defective, produced almost the same
amounts of vaccine-specific IgA in NW and IgG in serum as per WT mice (Figure 2d). Interferon regulatory factor 3 (IRF3) is the transcription factor mainly involved in TLR3-mediated type I
IFN production,21 but _Irf3_−/− mice showed no defect in either IgA or IgG production by polyI:C (Figure 2d). Thus the i.n. inoculation of polyI:C strongly augments the production of
vaccine-specific IgA and IgG through the TLR3-TICAM-1 pathway independent of MAVS, IRF3, and type I IFN. Actually, polyI:C and HA vaccine exhibited sufficient prophylactic effect on H1NI
influenza virus infection in WT mice, whereas the same recipe exhibited far less effect in _Ticam1_−/− mice, judged by body weight and survival (Figure 2e,f), the results being consistent
with those previously reported.2 AG-SPECIFIC T-CELL RESPONSE IS INDUCED THROUGH THE TICAM-1 PATHWAY To examine whether polyI:C potentiates Ag-specific T-cell responses, we prepared
splenocytes from the immunized mice and restimulated the cells with the split-vaccine (Figure 3). With polyI:C, vaccine significantly enhanced IFN-γ production, which is an indicator of
Ag-specific T-cell responses (Figure 3a–c). The levels of IFN-γ and the ratios of IFN-γ+CD4+ T and CD8+ T cells in splenocytes were incremented in response to Ag restimulation and comparable
between polyI:C-treated _Mavs_−/− and WT mice (Figure 3d–f). In contrast, the increases of these parameters were abrogated in splenocytes prepared from polyI:C-treated _Ticam1_−/− mice
(Figure 3d–f). _Tlr3_−/− mice also impaired the induction of Ag-specific IFN-γ production in splenic T cells (data not shown). Although the levels of Ig production were indistinguishable in
_Ifnar_−/−, _Irf3_−/−, and WT mice (Figure 2d), Ag-specific T-cell response was abrogated in _Ifnar_−/− and _Irf3_−/− mice (Figure 3g,h). Taken together, polyI:C augments Ag-specific T-cell
responses through the TLR3-TICAM-1 pathway. Type I IFN and IRF3 are dispensable for Ig production but optionally required for T-cell response in nasal vaccine in support of the polyI:C
adjuvanticity. CD103+DCS IN NALT EXPRESS TLR3 AND ARE ACTIVATED BY POLYI:C To clarify the mechanisms by which polyI:C enhances IgA production in the nasal cavity, we tried to identify the
TLR3-expressing cells in NALT. TLR3 is restrictedly expressed in certain DC subsets, such as CD8α+ and CD103+ DCs in mouse.22, 23 Actually, we found that TLR3 expression in CD11b+ or CD11c+
cells were selectively diminished in NALT of the _Tlr3_−/− mice (see Supplementary Figure S2a). As no CD8α+ DCs were detected in NALT (see Supplementary Figure S2b), we focused on CD103+ DCs
for further analysis. The results from flow cytometric and immunohistochemical analysis using an anti-TLR3 antibody (Ab) showed that CD11b−CD11c+CD103+ DCs (CD103+ DCs) expressed TLR3 in
NALT, as well as other CD11c+ cells (Figure 4a,b). The TLR3 expression was confirmed by reverse transcriptase–PCR in CD103+ DCs in NALT (Figure 4c). We next posed the question about whether
polyI:C is incorporated into TLR3-expressing DCs. Mice were administered with rhodamine-polyI:C (R-PIC) via i.n. inoculation. After 2 h, NALT sections were obtained and stained with
anti-CD11c and anti-TLR3 Abs. R-PIC was incorporated into CD11c+ DCs and colocalized with endosomal TLR3 (Figure 4d,e). CD103+ cells also took up R-PIC, which was colocalized with endosomal
TLR3 (Figure 4d,e). Isolated CD103+ DCs were able to incorporate polyI:C into TLR3 endosome _in vitro_ (Figure 4f). Thus at least TLR3-positive CD103+ DCs participate in polyI:C response. To
investigate whether nasal inoculation with polyI:C activates CD103+ DCs in NALT, we assessed the activation of DCs after i.n. polyI:C inoculation. CD86 and major histocompatibility complex
class II, activation makers of DCs, were upregulated on CD11c+ and CD103+ DCs in NALT in response to polyI:C (Figure 5a). Although CD11c+ DC activation occurred in _Ticam1_−/− mice, the
activation of CD103+ DCs was abolished in _Ticam1_−/− but not in _Mavs_−/− mice (Figure 5b). These findings by fluorescence-activated cell sorting analysis further endorse the imaging
results that CD103+ DCs in NALT take up polyI:C to mature through the TLR3-TICAM-1 pathway. The activation levels of _Ticam1_−/− CD11c+ and CD103+ DCs stimulated by cholera toxin (CT) were
comparable to those of WT DCs (data not shown), suggesting that _Ticam1_−/− CD11c+ and CD103+ DCs equivalently respond to an adjuvant that does not require TICAM-1. CD103+ DCS ARE
INDISPENSABLE FOR POLYI:C-ENHANCED IGA PRODUCTION Basic leucine zipper transcription factor ATF-like 3 (BATF3) is an essential transcriptional factor in the development of CD8α+ and CD103+
DCs.24 _Batf3_−/− mice exhibit loss of CD8α+ and CD103+ DCs in LNs.24 To examine the involvement of CD103+ DCs in Ig production, we evaluated IgA and IgG induction in _Batf3_−/− mice. CD103+
cells in NALT were abolished in _Batf3_−/− mice (Figure 6a). IgA induction by polyI:C was largely and IgG production in serum was less significantly decreased in _Batf3_−/− mice than in WT
mice (Figure 6b). Thus BATF3-dependent pathway is important for enhancement of IgA and, to a lesser extent, IgG production by polyI:C. The numbers and ratios of IgA+ B cells in
polyI:C-treated _Batf3_−/− NALT were also decreased compared with those in WT (see Supplementary Figure S1a,c), whereas the total numbers of B220+ B cells were unchanged after polyI:C
treatment (see Supplementary Figure S1d). Furthermore, the polyI:C-enhanced T-cell response was abolished in _Batf3_−/− mice (Figure 6c). Thus CD103+ DCs in NALT are essential for the
upregulation of Ag-specific IgA production and Ag-specific T-cell responses in response to i.n. inoculation of polyI:C. As _Batf3_−/− mice lack CD8α+ and CD103+ DCs, which are involved in
cross-presentation, they may impair Ag-specific CD8+ T-cell response.24 In addition, _Batf3_−/− mice also failed to induce Ag-specific CD4+ T-cell response in this study (Figure 6c). CD103+
DCs therefore support the function of CD8+ T as well as CD4+ T cells in nasal vaccination. As B cells marginally express TLR3 in NALT (Figure 7a), we next examined whether polyI:C directly
targets B cells for activation. PolyI:C and vaccine inoculation induced subtle activation of B cells in NALT prepared from WT and _Mavs_−/− mice but less induced in NALT from _Ticam1_−/−
mice (Figure 7b,c). However, B-cell activation was comparable in between _Batf3_−/− and WT mice (Figure 7b,c). Therefore, minor B-cell activation occurs independent of CD103+ DCs but
depending on TICAM-1, suggesting that i.n. inoculation of polyI:C participates in a direct route for B-cell activation in NALT, which appears through the TLR3 pathway. However, the direct
activation of B cells via the TLR3 pathway by polyI:C far less contributes to IgA production than CD103+ DCs in response to polyI:C. _In vitro_ IgA production in _Ticam1_−/− and _Batf3_−/− B
cells was normal relative to WT B cells (Figure 7d,e). Furthermore, CT, a representative mucosal adjuvant independent of the TLR3-TICAM-1 pathway, similarly induced IgA production and IgA+
B cells in NALT in WT, _Tlr3_−/−, and _Batf3_−/− mice (see Supplementary Figure S3). These data suggest that there is no global defect in Ab-producing ability in _Ticam1_−/− mice and the B
cells normally respond to CT for IgA production in _Ticam1_−/− and _Batf3_−/− mice. GERMINAL CENTER (GC) FORMATION IN NALT WAS ENHANCED BY POLYI:C Next we examined the effect of polyI:C on
GC formation in NALT. CD4+PD-1+CXCR5+ and B220+GL7+Fas+ are reported as markers for representing follicular T (Tfh) and GC B cells, respectively.25 PolyI:C significantly increased both
proportions and numbers of GC B and Tfh cells in WT mice after vaccination with polyI:C (Figure 8a–f). Treatment with PolyI:C failed to promote the GC formation in _Ticam1_−/− mice (Figure
8a–f), whereas polyI:C weakly increased the proportion of Tfh cells in _Batf3_−/− mice after vaccination with polyI:C (Figure 8d,e). The numbers of Tfh cells were not significantly
upregulated after polyI:C treatment in _Batf3_−/− mice (Figure 8f). Hence, the NALT IgA response occurs by vaccination with vaccine and polyI:C in a T-cell-mediated context in GC under the
DC network, which is abrogated in NALT of _Ticam1_−/− or _Batf3_−/− mice. _Ticam1_−/− mice have normal T-cell responses after influenza infection,26 suggesting that T-cell responses in
_Ticam1_−/− mice are sufficient in infection or _Ticam1_-independent stimuli, but selectively abrogated in polyI:C stimulation, that acts mainly on DCs. TLR3-TICAM-1 PATHWAY INDUCES IGA
CLASS SWITCH GENES IN NALT As TGF-β signaling in B cells and cytokines are crucial for the induction of TD IgA CSR,7, 8 we measured the expression of TGF-β-related genes and cytokines in
NALT after vaccination. PolyI:C did not induce _Tgfb1_, _2_, and _3_ mRNA expression in NALT (Figure 9a). Metalloprotease 9 (_Mmp9_), a protease that converts inactive TGF-β to the active
form to engage TGF-β receptors,27 was induced by vaccine and polyI:C inoculation (Figure 9b). Serpine peptidase inhibitor, clade E, member 1 (_Serpine1_) and TGF-β-induced protein (_Tgfbi_),
which are TGF-β-inducible genes,28, 29 were also upregulated by vaccine and polyI:C inoculation (Figure 9b). The expression of _Mmp9_, _Serpine1_, and _Tgfbi_ mRNA in response to vaccine
and polyI:C stimulation was abrogated in _Tlr3_−/− mice (Figure 9b). To confirm activation of TGF-β signaling in B cells, we examined phosphorylation of Smad2 protein in NALT by flow
cytometric analysis. The phosphorylated Smad2 protein was detected in B220+ B cells in WT mice by treatment with vaccine and polyI:C, while phosphorylated Smad2 protein was barely detected
in _Ticam1_−/− mice (Figure 9c). Moreover, the expression of cytokines involved in IgA class switching in NALT was upregulated in WT but not in _Ticam1_−/− (data not shown) or _Tlr3__−/−_
mice in response to polyI:C (Figure 9d). IL-21, which is one of the effector cytokines for formation of GC,30 was upregulated in WT but not in _Tlr3_−/− mice upon polyI:C inoculation (Figure
9d). PolyI:C-induced _Ifn-β_ mRNA expression in _Ticam1_−/− (data not shown) or _Tlr3_−/− mice was comparable to that in WT mice (Figure 9d), suggesting participation of the MAVS pathway.
The expression of CD40L on T cells and CD40 on B cells remained unchanged after vaccination with polyI:C (Figure 9e). As BAFF and APRIL on DCs are involved in the IgA CSR in the intestinal
tract and lung,8, 15 we examined the expression of these molecules on CD103+ DCs. BAFF expression was higher in NALT CD103+ DCs than in CD103− DCs (Figure 9f). BAFF expression was
upregulated after polyI:C treatment in WT mice but not in _Tlr3_−/− mice (Figure 9g). In contrast, APRIL expressed similarly between CD103+ and CD103− DCs (Figure 9f) and minimally induced
by polyI:C in WT and _Trl3_−/− mice (Figure 9g). Hence, polyI:C mainly upregulates the expression of BAFF in CD103+ DCs in a TLR3-TICAM-1-dependent manner. The expression of α germline
transcripts, a maker of initiation of class switching from Cμ to Cα,7 was specifically detected in NALT B cells prepared from mice with i.n. inoculation with vaccine and polyI:C (Figure 9h).
AID, which is an important factor for isotype switching in B cells,11 was expressed in NALT B cells 5 days after i.n. inoculation with polyI:C in WT mice, which was completely abrogated in
_Ticam1_−/− mice (Figure 9i). Taken together, the TLR3–TICAM-1 axis in NALT governs TGF-β signal-mediated T helper 2 skewing to induce class switching after i.n. inoculation with vaccine and
polyI:C. Our findings provide evidence that polyI:C induces various genes involved in the IgA CSR in NALT CD103+ DCs, resulting in the induction of AID expression to initiate Ig class
switching in B cells. DISCUSSION The purpose of this study was to clarify the mechanism by which polyI:C enhances vaccine-specific IgA production in the nasal cavity. CD103+ DCs are deeply
involved in IgA production, which is followed by the T- and B-cell responses in a TLR3-TICAM-1-dependent manner. MAVS, IRF3, and IFNAR barely participate in IgA production. PolyI:C activates
DCs through TLR3-TICAM-1 in NALT and allows B cells to induce the expression of AID via the DC/T-cell context. Expression of T helper 2–type cytokines and TGF-β-activating factors in NALT
are crucial for TGF-β signaling in B cells, although the source of TGF-β in NALT cells remains unidentified. Moreover, CD103+ DCs expressing TLR3 in NALT increasingly liberate BAFF in
response to polyI:C. PolyI:C directly activates B cells via TICAM-1 also, but this response is rather peripheral. Although a part of IgG isotype Ab production at steady state is slightly
impaired in _Myd88_−/−_Trif_Lps2/Lps2, TLR-associated enhancement of Ab productions are still controversial in terms of MyD88 (myeloid differentiation primary response gene 88) and TRIF
signaling.31 Furthermore, the signal pathway participating in sIgA response has remained unclear. This study sheds light on this issue: polyI:C-enhanced IgA production is clearly impaired in
_Tlr3_−/− or _Ticam1_−/− mice but these knockout mice retain sufficient capacity for T- and B-cell activation in NALT. Type I IFNs enhance Ag-specific Ig production and cellular responses,
thus having been used as a cytokine adjuvant.32, 33 However, the degrees of vaccine-specific Ig production in NALT appear to be similar in this study in _Ifnar_−/− and WT mice (Figure 2d)
and impaired in _Tlr3_−/− mice irrespective of _Ifn-β_ mRNA expression (Figure 9d), indicating that differential roles exist between the polyI:C and IFN therapy. PolyI:C-mediated T-cell
activation occurs depending upon IRF3 unlike IgA production, suggesting the presence of IRF3-bypass pathway for Ig production in DCs. Ultimately, TLR3-TICAM-1 participates in T-cell
activation and IgA production through two distinct pathways, IRF3/IFN and IRF3-independent axis. The TLR3 pathway activates transcription factors, IRF3, NF-κB, and activator protein-1, which
in turn induce type I IFN and inflammatory cytokines.5, 34 TICAM-1 specifically induces IL-1235 and activates TANK-binding kinase 1/I-kappa-B kinase ɛ and receptor-interacting protein 1/3
kinases in TLR3 signaling, which involves transcriptional activation of NF-κB and activator protein-1 for viral RNA-mediated host innate responses.21 Indeed, vaccine-specific Ig production
in _Irf3_−/− mice is significantly elevated by polyI:C treatment (Figure 2d). IRF3-bypass induction of B-cell activation may happen in myeloid DCs in response to viral infection.36
Therefore, the activation of NF-κB and activator protein-1 contributes to polyI:C-mediated Ig production in addition to IRF3-mediated T-cell activation. In accordance with previous
studies,24, 37 _Batf3_−/− mice lacking CD8α+ and CD103+ DCs abrogate Ag-specific CD8+ and CD4+ T-cell responses in our study (Figure 6). As CD103+ DCs in the skin and lung tissue are able to
present Ags to CD8+ T cells and CD4+ T cells,38, 39 NALT CD103+ DCs activated by polyI:C might also have the ability to prime CD8+ and CD4+ T cells. There are various DC subsets that sense
viral RNAs in mice,40, 41 but their roles in Ig production have been poorly defined yet. Ag-specific Ig induction after vaccine and polyI:C treatment is abrogated in CD4+ T-cell-depleted
mice (Figure 1e). Hence, polyI:C-enhanced Ig production requires the help of CD4+ T cells and entitles TD IgA production. The expression levels of genes involved in IgA induction, B-cell
activation, and phosphorylation of Smad2 in NALT were decreased in polyI:C-treated _Tlr3_−/− mice compared with WT mice (Figure 9c,d and data not shown). Therefore, TLR3-dependent DC
activation in concert with CD4+ T cells is induced by polyI:C and has an important role in TD IgA induction. The first study reported using _Batf3_−/− mice show that Ag-specific IgG and IgM
production is comparable with WT mice;24 but IgA production has not been examined. According to a recent study, IgA production in response to i.n. vaccination is impaired in _Batf3_−/−
mice.15 Consistently, _Batf3_−/− mice exhibit strong suppression of polyI:C-mediated IgA production in our study, albeit subtle reduction of IgG production (Figure 6b). These results infer
that NALT CD103+ DCs more potentially engage the production of IgA than IgG. The discrepancy between an earlier report24 and our present findings on IgG production in _Batf3_−/− mice remains
unknown but possibly lies on differences in the setting, administration route, type of Ags, and genetic background of the knockout mice. Although _Batf3_−/− mice with the 129 background
lack CD8α+ and CD103+ DCs, those with the C57B6/J background exhibit only partial depletion of CD8α+ and CD103+ DCs.42 Therefore, the remaining CD8α+ and CD103+ DCs in _Batf3_−/− mice with
the C57B6/J background are likely to modulate immune responses induced by polyI:C. Although NALT CD103+ DCs upregulated IgA expression in B cells _in vitro_, IgA expression was also induced
by NALT CD103− DCs (data not shown). Thus we do not exclude the possibility that TLR3-positive DCs other than CD103+ DCs are involved in IgA production. The IgA CSR occurs in B cells in the
GCs of secondary lymphoid organs and requires AID expression and requires cytokines, especially TGF-β, with or without the help of CD4+ T cells.7, 8 Previous reports show that the formation
of GCs, AID expression, and an IgA CSR occur in NALT after i.n. inoculation of Ags.18, 43 Our findings are consistent with previous reports43 in that i.n. inoculation with polyI:C results in
increased levels of AID expression in NALT B cells (Figure 9i). Although TGF-β signaling is a potent inducer of the IgA CSR in B cells,10 polyI:C did not directly induce _Tgf-β1_, _2_, and
_3_ mRNA expression in NALT (Figure 9a). The results may reflect the posttranscriptional regulation of TGF-β activity.44 Activation of TGF-β is induced by its release from latency-associated
protein, which in turn is promoted by αV integrin and MMP proteins, because TGF-β is produced as an inactive form, which can bind latency-associated protein.44 Although _Tgf-β_ mRNA
expression is unaffected in response to polyI:C inoculation, polyI:C enables NALT cells to upregulate TGF-β-inducible genes and phosphorylate Smad2 protein (Figure 9b,c). Because Peyer’s
patch DCs and CD103+ DCs expressing _Tgf-β_ mRNA are able to induce regulatory T cells in gut-associated lymph tissue,45 these DCs may produce TGF-β in gut-associated lymph tissue. Lung
CD103+ DCs also produce TGF-β and induce IgA+ B cells.15 Yet, we could not identify cell types responsible for the supply of TGF-β or enzymes conversing to active forms in NALT. Epithelial
cells are an alternative source of active TGF-β.46 Because polyI:C did not directly induce TGF-β-inducible genes from the relevant DC subsets, such as splenic and bone marrow–derived DCs
(data not shown), NALT epithelial cells may have a role in the production of TGF-β. In fact, polyI:C and influenza viruses evoke activation of TLR3 signaling and then TGF-β in epithelial
cells.45, 46 I.n. administration of polyI:C and HA induces a high titer of anti-HA IgA2 and brings effective cross-protection against various H1N1 influenza virus challenge in mouse models.
Here we show the mechanism by which polyI:C acts as an HA-specific IgA-producing adjuvant in nasal immunity. CD103+ DCs have a critical role via endosomal TLR3 and TICAM-1, rather than MAVS
or IFNAR, in NALT IgA production; this DC subset transports influenza virus to the draining LNs in the lung, and mice were protected from infection by inducing effective cross-priming of
CD8+ T cells.47, 48, 49 Likewise, NALT CD103+ DCs may co-operate with the respiratory system in provoking virus RNA-specific CD8+ T-cell responses.50 In either case, CD4+ T cells work for
successful vaccination. TLR3 adjuvant makes appropriate cellular response against influenza throughout DC-enhanced events via NALT. METHODS HA VACCINE AND VACCINATION Split-product HA
vaccine prepared from A/California/07/2009 (H1N1)pdm09 was gifted from Dr Y. Gomi in Research Foundation for Microbial Disease of Osaka University (Osaka, Japan). PolyI:C was purchased from
GE Healthcare Japan (Tokyo, Japan). Mice were anesthetized with pentobarbital sodium and xylazine and i.n. immunized by dropping 5 μl phosphate-buffered saline containing 1 μg HA vaccine
with or without 3 μg polyI:C or 1 μg CT (Wako Pure Chemical Industries, Osaka, Japan) as liquid drops into each nostril. Four weeks later, mice were re-immunized by the same method. Two
weeks after second immunization, blood samples and NW fluid were collected from mice anesthetized by pentobarbital sodium and xylazine to measure vaccine-specific IgA and IgG Ab production.
The levels of IgA and IgG Abs against HA were measured by ELISA as described previously2 using plates coated with HA protein purified from vaccine strain. Purified HA protein was gifted from
Dr H. Hasegawa (National Institute of Infectious Disease, Tokyo, Japan). HA-coated plates were blocked with 1% bovine serum albumin/phosphate-buffered saline for 2 h and blocking buffer was
discarded. Serum and NW were overlaid and then biotin-labeled goat anti-mouse IgG (Jackson ImmunoResearch, West Grove, PA) or IgA (Kirkegaard & Perry Laboratories, Gaithersburg, MD)
were added. Streptavidin-alkaline phosphatase (Thermo Fisher Scientific, Waltham, MA) was reacted with biotin and detection was performed using p-nitrophenyl phosphate (Sigma-Aldrich, St.
Louis, MO). The chromogen was measured by detection of absorbance at 405 nm using SUNRISE plate reader (TECAN, Mannedorf, Switzerland). For preparation of standard IgG in serum and IgA in
NW, Balb/c mice were immunized as described above. To determine ELISA unit in standard samples, standard IgG in serum and IgA in NW were diluted in twofold steps from 1:200 to 1:6,553,600
for serum and 1:1 to 1:32,768 for NW with blocking buffer and performed ELISA as described above. The standard IgG in serum and IgA in NW had an ELISA end point titer of 1:409,600 and
1:2,048, respectively. These end point titers of each standard IgG in serum and IgA in NW were arbitrarily decided as 1 ELISA unit. Standard IgG in serum and IgA in NW were incorporated into
each ELISA assay to make a standard curve. The IgG and IgA ELISA unit in test samples were calculated from their optical density at 405 nm values using the standard curve. Test serum
samples and NW samples were diluted in 1:200 and 1:1, respectively. For CD4+ T-cell depletion, mice were intraperitoneally injected with anti-murine CD4 Ab prepared from GK1.5 hybridoma
before vaccination. Optimal doses of the Abs were determined in preliminary studies (150 μl per body). We confirmed a decrease in CD4+ T cells in the spleen, mesenteric LN and NALT. Detailed
descriptions of the materials and methods used in this work are provided in Supplementary Materials and Methods. STATISTICAL ANALYSES Statistical significance of differences between groups
was determined by the Student’s _t_-test, one-way analysis of variance test with Bonferroni’s multiple comparison _post hoc_ test and Mann–Whitney _U_-test, using Prism 4 (GraphPad Software,
San Diego, CA). Values of _P_<0.05 were considered significant. REFERENCES * Houser, K. & Subbarao, K. Influenza vaccines: challenges and solutions. _Cell Host Microbe_ 17, 295–300
(2015). Article CAS Google Scholar * Ichinohe, T. _et al_. Synthetic double-stranded RNA poly(I:C) combined with mucosal vaccine protects against influenza virus infection. _J. Virol._
79, 2910–2919 (2005). Article CAS Google Scholar * Ichinohe, T., Ainai, A., Tashiro, M., Sata, T. & Hasegawa, H. PolyI:polyC12U adjuvant-combined intranasal vaccine protects mice
against highly pathogenic H5N1 influenza virus variants. _Vaccine_ 27, 6276–6279 (2009). Article CAS Google Scholar * Kawai, T. _et al_. IPS-1, an adaptor triggering RIG-I- and
Mda5-mediated type I interferon induction. _Nat. Immunol._ 6, 981–988 (2005). Article CAS Google Scholar * Oshiumi, H., Matsumoto, M., Funami, K., Akazawa, T. & Seya, T. TICAM-1, an
adaptor molecule that participates in Toll-like receptor 3-mediated interferon-beta induction. _Nat. Immunol._ 4, 161–167 (2003). Article CAS Google Scholar * Matsumoto, M. & Seya, T.
TLR3: interferon induction by double-stranded RNA including poly(I:C). _Adv Drug Deliv. Rev._ 60, 805–812 (2008). Article CAS Google Scholar * Cerutti, A. The regulation of IgA class
switching. _Nat. Rev. Immunol._ 8, 421–434 (2008). Article CAS Google Scholar * Bemark, M., Boysen, P. & Lycke, N.Y. Induction of gut IgA production through T cell-dependent and T
cell-independent pathways. _Ann. NY Acad. Sci._ 1247, 97–116 (2012). Article CAS Google Scholar * Litinskiy, M.B. _et al_. DCs induce CD40-independent immunoglobulin class switching
through BLyS and APRIL. _Nat. Immunol._ 3, 822–829 (2002). Article CAS Google Scholar * Coffman, R.L., Lebman, D.A. & Shrader, B. Transforming growth factor beta specifically enhances
IgA production by lipopolysaccharide-stimulated murine B lymphocytes. _J. Exp. Med._ 170, 1039–1044 (1989). Article CAS Google Scholar * Muramatsu, M. _et al_. Class switch recombination
and hypermutation require activation-induced cytidine deaminase (AID), a potential RNA editing enzyme. _Cell_ 102, 553–563 (2000). Article CAS Google Scholar * Tezuka, H. _et al_.
Regulation of IgA production by naturally occurring TNF/iNOS-producing dendritic cells. _Nature_ 448, 929–933 (2007). Article CAS Google Scholar * Tezuka, H. _et al_. Prominent role for
plasmacytoid dendritic cells in mucosal T cell-independent IgA induction. _Immunity_ 34, 247–257 (2011). Article CAS Google Scholar * Fujimoto, K. _et al_. A new subset of CD103+CD8alpha+
dendritic cells in the small intestine expresses TLR3, TLR7, and TLR9 and induces Th1 response and CTL activity. _J. Immunol._ 186, 6287–6295 (2011). Article CAS Google Scholar * Ruane,
D. _et al_. Microbiota regulate the ability of lung dendritic cells to induce IgA class-switch recombination and generate protective gastrointestinal immune responses. _J. Exp. Med._ 213,
53–73 (2016). Article CAS Google Scholar * Kiyono, H. & Fukuyama, S. NALT- versus Peyer’s-patch-mediated mucosal immunity. _Nat. Rev. Immunol._ 4, 699–710 (2004). Article CAS Google
Scholar * Liang, B., Hyland, L. & Hou, S. Nasal-associated lymphoid tissue is a site of long-term virus-specific antibody production following respiratory virus infection of mice. _J.
Virol._ 75, 5416–5420 (2001). Article CAS Google Scholar * Nacer, A. _et al_. Imaging murine NALT following intranasal immunization with flagellin-modified circumsporozoite protein
malaria vaccines. _Mucosal Immunol._ 7, 304–314 (2014). Article CAS Google Scholar * Errea, A., González Maciel, D., Hiriart, Y., Hozbor, D. & Rumbo, M. Intranasal administration of
TLR agonists induces a discriminated local innate response along murine respiratory tract. _Immunol. Lett._ 164, 33–39 (2015). Article CAS Google Scholar * Longhi, M.P. _et al_. Dendritic
cells require a systemic type I interferon response to mature and induce CD4+ Th1 immunity with poly IC as adjuvant. _J. Exp. Med._ 206, 1589–1602 (2009). Article CAS Google Scholar *
Kawai, T. & Akira, S. Signaling to NF-kappaB by Toll-like receptors. _Trends Mol. Med._ 13, 460–469 (2007). Article CAS Google Scholar * Desch, A.N. _et al_. CD103+ pulmonary
dendritic cells preferentially acquire and present apoptotic cell-associated antigen. _J. Exp. Med._ 208, 1789–1797 (2011). Article CAS Google Scholar * Edwards, A.D. _et al_. Toll-like
receptor expression in murine DC subsets: lack of TLR7 expression by CD8 alpha+ DC correlates with unresponsiveness to imidazoquinolines. _Eur. J. Immunol._ 33, 827–833 (2003). Article CAS
Google Scholar * Hildner, K. _et al_. Batf3 deficiency reveals a critical role for CD8alpha+ dendritic cells in cytotoxic T cell immunity. _Science_ 322, 1097–1100 (2008). Article CAS
Google Scholar * Awe, O. _et al_. PU.1 expression in T follicular helper cells limits CD40L-dependent germinal center B cell development. _J. Immunol._ 195, 3705–3715 (2015). Article CAS
Google Scholar * Koyama, S. _et al_. Differential role of TLR- and RLR-signaling in the immune responses to influenza A virus infection and vaccination. _J. Immunol._ 179, 4711–4720 (2007).
Article CAS Google Scholar * Jenkins, G. The role of proteases in transforming growth factor-beta activation. _Int. J. Biochem. Cell Biol._ 40, 1068–1078 (2008). Article CAS Google
Scholar * Keeton, M.R., Curriden, S.A., van Zonneveld, A.J. & Loskutoff, D.J. Identification of regulatory sequences in the type 1 plasminogen activator inhibitor gene responsive to
transforming growth factor beta. _J. Biol. Chem._ 266, 23048–23052 (1991). CAS PubMed Google Scholar * Skonier, J. _et al_. cDNA cloning and sequence analysis of beta ig-h3, a novel gene
induced in a human adenocarcinoma cell line after treatment with transforming growth factor-beta. _DNA Cell Biol._ 11, 511–522 (1992). Article CAS Google Scholar * Spolski, R. &
Leonard, W.J. Interleukin-21: a double-edged sword with therapeutic potential. _Nat. Rev. Drug Discov._ 13, 379–395 (2014). Article CAS Google Scholar * Gavin, A.L. _et al_.
Adjuvant-enhanced antibody responses in the absence of Toll-like receptor signaling. _Science_ 314, 1936–1938 (2006). Article CAS Google Scholar * Proietti, E. _et al_. Type I IFN as a
natural adjuvant for a protective immune response: lessons from the influenza vaccine model. _J. Immunol._ 169, 375–383 (2002). Article CAS Google Scholar * Tovey, M.G., Lallemand, C.
& Thyphronitis, G. Adjuvant activity of type I interferons. _Biol. Chem._ 389, 541–545 (2008). Article CAS Google Scholar * Meylan, E. _et al_. RIP1 is an essential mediator of
Toll-like receptor 3-induced NF-kappa B activation. _Nat. Immunol._ 5, 503–507 (2004). Article CAS Google Scholar * Matsumoto, M. _et al_. Defined TLR3-specific adjuvant that induces NK
and CTL activation without significant cytokine production _in vivo_. _Nat. Commun._ 6, 6280 (2015). Article CAS Google Scholar * Takaki, H. _et al_. MAVS-dependent IRF3/7 bypass of
interferon β-induction restricts the response to measles infection in CD150Tg mouse bone marrow-derived dendritic cells. _Mol. Immunol._ 57, 100–110 (2013). Article Google Scholar *
Mashayekhi, M. _et al_. CD8α(+) dendritic cells are the critical source of interleukin-12 that controls acute infection by Toxoplasma gondii tachyzoites. _Immunity_ 35, 249–259 (2011).
Article CAS Google Scholar * Bedoui, S. _et al_. Cross-presentation of viral and self antigens by skin-derived CD103+ dendritic cells. _Nat. Immunol._ 10, 488–495 (2009). Article CAS
Google Scholar * Nakano, H. _et al_. Pulmonary CD103(+) dendritic cells prime Th2 responses to inhaled allergens. _Mucosal Immunol._ 5, 53–65 (2012). Article CAS Google Scholar * Takaki,
H. _et al_. The MyD88 pathway in plasmacytoid and CD4+ dendritic cells primarily triggers type I IFN production against measles virus in a mouse infection model. _J. Immunol._ 191,
4740–4747 (2013). Article CAS Google Scholar * Takaki, H., Oshiumi, H., Matsumoto, M. & Seya, T. Dendritic cell subsets involved in type I IFN induction in mouse measles virus
infection models. _Int. J. Biochem. Cell Biol._ 53, 329–333 (2014). Article CAS Google Scholar * Tussiwand, R. _et al_. Compensatory dendritic cell development mediated by BATF-IRF
interactions. _Nature_ 490, 502–507 (2012). Article CAS Google Scholar * Shikina, T. _et al_. IgA class switch occurs in the organized nasopharynx- and gut-associated lymphoid tissue, but
not in the diffuse lamina propria of airways and gut. _J. Immunol._ 172, 6259–6264 (2004). Article CAS Google Scholar * Massagué, J. & Chen, Y.G. Controlling TGF-beta signaling.
_Genes Dev._ 14, 627–644 (2000). PubMed Google Scholar * Coombes, J.L. _et al_. A functionally specialized population of mucosal CD103+ DCs induces Foxp3+ regulatory T cells via a TGF-beta
and retinoic acid-dependent mechanism. _J. Exp. Med._ 204, 1757–1764 (2007). Article CAS Google Scholar * Jolly, L. _et al_. Influenza promotes collagen deposition via _α_v_β_6
integrin-mediated transforming growth factor _β_ activation. _J. Biol. Chem._ 289, 35246–35263 (2014). Article CAS Google Scholar * Helft, J. _et al_. Cross-presenting CD103+ dendritic
cells are protected from influenza virus infection. _J. Clin. Invest._ 122, 4037–4047 (2012). Article CAS Google Scholar * Sung, S.S. _et al_. A major lung CD103 (alphaE)-beta7
integrin-positive epithelial dendritic cell population expressing Langerin and tight junction proteins. _J. Immunol._ 176, 2161–2172 (2006). Article CAS Google Scholar * del Rio, M.L.,
Rodriguez-Barbosa, J.I., Kremmer, E. & Förster, R. CD103- and CD103+ bronchial lymph node dendritic cells are specialized in presenting and cross-presenting innocuous antigen to CD4+ and
CD8+ T cells. _J. Immunol._ 178, 6861–6866 (2007). Article CAS Google Scholar * Waithman, J. _et al_. Resident CD8(+) and migratory CD103(+) dendritic cells control CD8 T cell immunity
during acute influenza infection. _PLoS ONE_ 8, e66136 (2013). Article CAS Google Scholar Download references ACKNOWLEDGEMENTS This work was supported in part by Grants-in-Aid from the
Ministry of Education, Culture, Sports, Science, and Technology, Research program on Emerging and Re-emerging Infection Diseases from Japan Agency for Medical Research and Development
(AMED), Takeda Science Foundation, Uehara Memorial Foundation, Isukura, and the Akiyama Life Science Foundation. AUTHOR CONTRIBUTIONS T. Seya, H.T., and M.M. contributed to conception and
design; H.T. and S.K. contributed to acquisition of data; H.T., T. Suzuki, A.A., H.H., H.O., and Y.S. contributed to the development of methodology; H.T., H.O., M.M., and T. Seya contributed
to analysis and interpretation of data; H.T., H.O., and T. Seya contributed to writing the manuscript. AUTHOR INFORMATION AUTHORS AND AFFILIATIONS * Department of Microbiology and
Immunology, Graduate School of Medicine, Hokkaido University, Sapporo, Japan H Takaki, S Kure, M Matsumoto & T Seya * Department of Immunology, Graduate School of Medical Sciences,
Kumamoto University, Kumamoto, Japan H Oshiumi * Department of Disease Control, Laboratory of Microbiology, Graduate School of Veterinary Medicine, Hokkaido University, Sapporo, Japan Y
Sakoda * Global Station for Zoonosis Control, Global Institution for Collaborative Research and Education (GI-CoRE), Hokkaido University, Sapporo, Japan Y Sakoda * Department of Pathology,
National Institute of Infectious Diseases, Shinjuku, Tokyo, Japan T Suzuki, A Ainai & H Hasegawa Authors * H Takaki View author publications You can also search for this author inPubMed
Google Scholar * S Kure View author publications You can also search for this author inPubMed Google Scholar * H Oshiumi View author publications You can also search for this author inPubMed
Google Scholar * Y Sakoda View author publications You can also search for this author inPubMed Google Scholar * T Suzuki View author publications You can also search for this author
inPubMed Google Scholar * A Ainai View author publications You can also search for this author inPubMed Google Scholar * H Hasegawa View author publications You can also search for this
author inPubMed Google Scholar * M Matsumoto View author publications You can also search for this author inPubMed Google Scholar * T Seya View author publications You can also search for
this author inPubMed Google Scholar CORRESPONDING AUTHORS Correspondence to H Takaki or T Seya. ETHICS DECLARATIONS COMPETING INTERESTS The authors declared no conflict of interest.
ADDITIONAL INFORMATION Supplementary Material is linked to the online version of the paper SUPPLEMENTARY INFORMATION SUPPLEMENTARY INFORMATION (PDF 1246 KB) POWERPOINT SLIDES POWERPOINT
SLIDE FOR FIG. 1 POWERPOINT SLIDE FOR FIG. 2 POWERPOINT SLIDE FOR FIG. 3 POWERPOINT SLIDE FOR FIG. 4 POWERPOINT SLIDE FOR FIG. 5 POWERPOINT SLIDE FOR FIG. 6 POWERPOINT SLIDE FOR FIG. 7
POWERPOINT SLIDE FOR FIG. 8 POWERPOINT SLIDE FOR FIG. 9 RIGHTS AND PERMISSIONS Reprints and permissions ABOUT THIS ARTICLE CITE THIS ARTICLE Takaki, H., Kure, S., Oshiumi, H. _et al._
Toll-like receptor 3 in nasal CD103+ dendritic cells is involved in immunoglobulin A production. _Mucosal Immunol_ 11, 82–96 (2018). https://doi.org/10.1038/mi.2017.48 Download citation *
Received: 24 August 2016 * Accepted: 23 April 2017 * Published: 15 June 2017 * Issue Date: January 2018 * DOI: https://doi.org/10.1038/mi.2017.48 SHARE THIS ARTICLE Anyone you share the
following link with will be able to read this content: Get shareable link Sorry, a shareable link is not currently available for this article. Copy to clipboard Provided by the Springer
Nature SharedIt content-sharing initiative