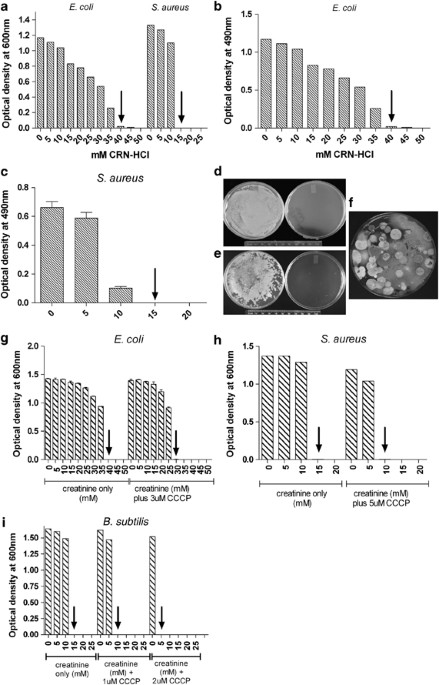
- Select a language for the TTS:
- UK English Female
- UK English Male
- US English Female
- US English Male
- Australian Female
- Australian Male
- Language selected: (auto detect) - EN
Play all audios:
Widespread antibiotic use has resulted in increased frequency of clinically important bacteria acquiring single or multiple antibiotic resistance.1, 2 Even antibiotic therapies for
relatively trivial afflictions, such as acne,3 have promoted development of microbial antibiotic resistance. The availability of non-prescription antibiotics in some areas has also resulted
in improper and/or irrational self-medication, further exacerbating this problem.4, 5 New antibacterial agents with broad-spectrum impact against both Gram-positive6 and Gram-negative7
species, as well as against drug-resistant strains such as methicillin-resistant _Staphylococcus aureus_8 are needed for wound care and to treat topical and dermatological infections.9 A
chance observation in our laboratory revealed that creatinine (CRN; creatinine hydrochloride, CRN-HCl) halted the growth of bacteria on nutrient agar plates. CRN is the naturally occurring
breakdown product of creatine phosphate, a high-energy molecule used to store and then donate, a high-energy phosphate to ADP for the synthesis of ATP in metabolism. Occurring normally in
human blood at concentrations ranging approximately between 50–100 μM and in urine at slightly higher levels, CRN is accepted to be a naturally produced inert waste product with no active
function,10 although a recently published study has challenged this dogma.11 We characterized the ability of CRN-HCl to inhibit the growth of a wide array of bacterial species, including
methicillin-resistant _Staphylococcus aureus_. CRN-HCl (Sigma-Aldrich; St Louis, MO, USA; F.W.=149.6) was evaluated for its ability to inhibit the growth of different bacteria. Bacterial
cultures were propagated in LBG (LB broth, Miller; Fisher Scientific; Fair Lawn, NJ, USA) supplemented with 1% w/v glucose) either in liquid or agar (BD Bacto Agar; Becton Dickinson, Sparks,
MD, USA) format. Overnight cultures of _Escherichia coli_ and _S. aureus_ were diluted in fresh LBG to 1–5 × 105 colony-forming units (c.f.u.) per ml, aliquoted to 2 ml tube cultures, to
each of which was added CRN-HCl in increasing 5 mM increments from 0–50 mM. After shaking at 250 r.p.m. at 37 °C for 18 h, the OD at 600 nm was recorded. _E. coli_ was inhibited from growth
in 40 mM (5.98 mg ml−1) CRN-HCl, while _S. aureus_ growth was inhibited at a concentration of 15 mM (2.24 mg ml−1) CRN-HCl (Figure 1a). These values were taken as a measurement of the MIC
for CRN-HCl under these specific assay conditions. A similar experiment was performed using a 96-well microtiter plate format in which triplicate wells were filled with 100ul total volume of
_E. coli_ or _S. aureus_ (final concentration of 1–5 × 105 c.f.u. ml−1) in LBG containing increasing concentrations of CRN-HCl. Following 18 h stationary incubation at 37 °C, wells were
examined visually to ascertain which wells appeared turbid versus clear, then the plate was assayed using an ELISA plate reader (ELX800; Bio-Tek Instruments, Winooski, VT, USA) using a 490
nm wavelength (Figures 1b and c). This format confirmed the results from the tube cultures, demonstrating the inhibition of the growth of _E. coli_ and _S. aureus_ at 40 and 15 mM CRN-HCl,
respectively. Using this microtiter plate format, MIC values for four other bacterial species were established: _Brevibacterium linens_ (MIC=10 mM), _Bacillus subtilis_ (MIC=20 mM),
_Pseudomonas aeruginosa_ (20 mM) and _Streptococcus pyogenes_ (MIC=20 mM; data not shown). Drug-resistant bacterial strains and other bacterial species were assayed for sensitivity to
inhibition of replication by CRN-HCl using a disc diffusion assay. Assays were performed as described12 with the following modifications. Twenty-five microliters of 2 M CRN-HCl in water was
added to 30 mg of a powdered carrier (Eridex; Cargill Inc., Cedar Rapids, IA, USA) and stirred into a thickened slurry in order to apply the maximum amount of CRN-HCl on the disc. Fifty
microliters of the slurry containing 5 mg CRN-HCl were applied to 6 mm diameter sterile dry paper discs (Whatman no. 3 filter paper; Whatman, Piscataway, NJ, USA) that were then inverted
onto LBG agar plates containing test bacteria. The LBG agar plates were prepared 15 min before use by spreading the test bacteria (diluted in phosphate-buffered saline to 1–5 × 105 c.f.u.
ml−1 from overnight cultures) to the plate with a sterile cotton swab. The plates with discs were incubated for 15 h at the temperature appropriate for the particular bacterial species.
Clear zones around the discs, indicative of growth inhibition, were measured. As a point of comparison, gentamicin-impregnated discs (GM-10; Becton Dickinson) were tested. For all bacteria
but drug-resistant and anaerobic bacteria, for which assays were repeated twice, discs were tested in triplicate on two different days and zones of inhibition were recorded as the average of
these six measurements. Variation was ⩽2 mm. Results for the diverse bacterial species assayed using this approach are shown in Table 1. All bacteria tested in this manner, including
drug-resistant strains, were inhibited by CRN-HCl with a range of zone sizes between 16–40 mm. The ability of CRN-HCl to suppress unknown bacterial growth in environmental samples (fecal
matter and soil) was also tested (Figures 1d and e). Solids from a human fecal swab were suspended in LBG (∼1% w/v) and spread either on control plates containing LBG agar or test plates
containing LBG agar poured in 100 mM CRN-HCl. After 24 h incubation at 37 °C, rampant bacterial growth was observed on the control plate but growth was completely suppressed by CRN-HCl (a
typical example is shown in Figure 1d; compare control plate on the left to the CRN-HCl-containing plate on the right). Similar experiments using garden soil (1% w/v) as the source of
microorganisms gave the same result: complete ablation of bacterial outgrowth in the presence of CRN-HCl (Figure 1e). Sterile swabbing of the surface of the clear CRN-HCl-containing plates
(3 days after initial plating) followed by transfer of the swabbed material to control LBG nutrient agar plates, revealed outgrowth of bacterial colonies at varying numbers depending upon
the sample used (data not shown). However, none of these outgrowing bacteria replicated when subsequently streaked onto 100 mM CRN-HCl-containing LBG nutrient agar (data not shown). We infer
that these represented outgrowth of persistent bacterial spores that had not previously germinated. Occasionally, growth of diverse fungi on CRN-HCl-containing agar days after the plating
environmental samples was observed, indicating that eukaryotic microorganisms were not inhibited by CRN-HCl (Figure 1e). We therefore tested the ability of 100 mM CRN-HCl to inhibit the
growth of three different yeasts (_Candida albicans_, a _Rhodotorula_ species, and a _Saccharomyces_ species; Table 1). No growth inhibition of any of the yeast strains was observed on these
plates (similar results were obtained in liquid culture containing 100–500 mM CRN-HCl; data not shown). Preliminary studies revealed that CRN-HCl—but neither the non-salt anhydrous form of
CRN (Sigma-Aldrich) nor the creatine monohydrate (Creapure; Degussa AG, Dusseldorf, Germany)—inhibited bacterial growth (data not shown). Using anhydrous CRN, we formed acetate and sulfate
salts of CRN and showed that these, too, functioned as antibacterial agents similar to CRN-HCl (data not shown). We hypothesized therefore that only the protonated form13 of CRN inhibited
the growth of diverse bacterial species, perhaps by overwhelming the bacterial cells capacity to pump out protons.14, 15 To test this hypothesis, we used CCCP14 (carbonyl cyanide
3-chlorophenylhydrazone; Sigma-Aldrich) to disrupt the proton gradient in cultures of _E. coli_, _S. aureus,_ and _B. subtilis_. Tube cultures from diluted overnight cultures were
established as described above, each containing the concentration of CCCP and CRN-HCl noted in the figure. Following 18 h shaking at 250 r.p.m. at 37 °C, the OD for each culture was read at
600 nm. Each bacterial species required a 5–10 mM lower CRN-HCl concentration to inhibit replication in the presence of 1–5 μM CCCP as measured by OD of overnight cultures (Figures 1g, h and
i). It is widely accepted that CRN is a pharmacologically inactive waste product of muscle metabolism devoid of biological activity.10, 16 Thus, our discovery that this common nitrogenous
waste molecule has antibacterial antibiotic properties is, to the best of our knowledge, unique and potentially of great interest. When tested at physiological levels (50–200 μM) on agar or
in broth culture, CRN-HCl did not suppress bacterial growth relative to control cultures (data not shown) but when used between 10–100 mM, suppression of growth and killing of all bacterial
species were observed including not only drug-resistant strains, such as methicillin-resistant _Staphylococcus aureus_, but also uncharacterized environmental bacterial strains as well. The
mechanism of action is as yet unclear. We know that _Streptococcus_ species, which lack the citric acid cycle17 and _Staphylococcus_ species, which use the citric acid pathway, were both
inhibited by CRN-HCl (data not shown). CRN-HCl also inhibited the growth of an arginine-deficient mutant of _Staphylococcus epidermidis_ compared with the wild-type control strain of _S.
epidermidis_ (both courtesy of Dr P Fey), indicating the CRN-HCl-induced growth inhibition was not arginine deaminase dependent.18 As _Clostridium difficile_ growth and replication was
inhibited under anaerobic conditions, thus, we can infer that a lack of oxygen does not affect the suppressive mechanism of CRN-HCl. Our observations that CRN required protonation for the
antibacterial function and that inhibition of proton conductance by CCCP lowered the concentration of CRN-HCl required to suppress bacterial growth are consistent with a working hypothesis
that inhibitory CRN-HCl concentrations lead to acidification of the bacterial cytoplasm. Collectively, the results indicate that the mechanism of the suppressive action of CRN-HCl used in
this study is likely held in common across diverse bacterial species. The antibacterial characteristics suggest CRN-HCl or other salts of CRN may have potential for the addition to the
armamentarium of agents to inhibit dermatological bacterial infections. We propose that CRN-HCl may find diverse applications in wound care and treatment as a supplement to, or replacement
for, currently used antibacterial agents. Various other clinical, veterinary and industrial applications of CRN-HCL can also be envisaged. REFERENCES * Andersson, D. & Hughes, D.
Antibiotic resistance and its cost: is it possible to reverse resistance? _Nat. Rev. Microbiol._ 8, 260–271 (2010). Article CAS Google Scholar * Boucher, H. _et al_. Bad bugs, no drugs:
no ESKAPE! An update from the Infectious Diseases Society of America. _Clin. Infect. Dis._ 48, 1–12 (2009). Article Google Scholar * Patel, M., Boew, W., Heughebaert, C. & Shalita, A.
R. The development of antimicrobial resistance due to antibiotic treatment of acne vulgaris: a review. _J. Drugs Dermatol._ 9, 655–664 (2010). PubMed Google Scholar * Vannanen, M.,
Pietila, K. & Airaksanen, M. Self-medication with antibiotics - does it really happen in Europe? _Health Policy_ 77, 166–171 (2006). Article Google Scholar * Grigoryan, J. _et al_. Is
self-medication with antibiotics in Europe driven by prescribed use? _J. Antimicrob. Chemother._ 59, 152–156 (2007). Article CAS Google Scholar * Cornaglia, G. & Rossolini, G.
Forthcoming therapeutic perspectives for infections due to multidrug resistant Gram-positive pathogens. _Clin. Microbiol. Infect._ 15, 281–323 (2009). Article Google Scholar * Peleg, A.
& Hooper, D. Hospital-acquired infections due to gram negative bacteria. _New Engl. J. Med._ 362, 1804–1813 (2010). Article CAS Google Scholar * David, M. Z. & Daum, R. S.
Community-associated methicillin-resistant Staphylococcus aureus: epidemiological and clinical consequences of an emerging epidemic. _Clin. Microbiol. Rev._ 23, 616–687 (2010). Article CAS
Google Scholar * Lipsky, B. & Hoey, C. Topical antimicrobial therapy for treating chronic wounds. _Clin. Practice_ 49, 1541–1549 (2009). Google Scholar * Harris,, R. & Crabb,, D.
in _Textbook of Biochemistry with Clinical Correlations_ (ed. Devlin, T.) 884 (Wiley-Liss, New York, 2005). Google Scholar * Leland, K., Drescher, K. M. & McDonald, T. L. Effect of
creatine, creatinine, and creatine ethyl ester on TLR expression in macrophages. _Intl. Immunopharmacol._ 11, 1341–1347 (2011). Article CAS Google Scholar * Clinical and Laboratory
Standards Institute. _Performance Standards for Antimicrobial Disk Susceptibility Tests_ Clinical and Laboratory Standards Institute (CLSI) document M2–A9 (CLSI, Wayne, PA, 2006). *
Srinivasan, R. & Stewart, R. The catalysis of proton exchange in creatinine by general acids and bases. _Can. J. Chem._ 53, 224–231 (1974). Article Google Scholar * Nikaido, H. &
Takatsuka, Y. Mechanisms of RND multidrug efflux pumps. _BBA - Proteins Proteomics_ 1794, 769–781 (2009). Article CAS Google Scholar * Piddock, L. J. Multi-drug resistance efflux pumps -
not just for resistance. _Nat. Rev. Microbiol._ 4, 629–636 (2006). Article CAS Google Scholar * Wyss, M. & Kaddurah-Daouk, R. Creatine and creatinine metabolism. _Phyisol. Rev._ 80,
1107–1213 (2000). CAS Google Scholar * Somerville, G. _et al_. Correlation of acetate catabolism and growth yield in Staphylococcus aureus: implications for host-pathogen interactions.
_Infect. Immun._ 71, 4724–4732 (2003). Article CAS Google Scholar * Zhu, Y. _et al_. Staphylococcus aureus biofilm metabolism and the influence of arginine on polysaccharide intercellular
adhesin synthesis, biofilm formation and pathogenesis. _Infect. Immun._ 75, 4219–4226 (2007). Article CAS Google Scholar Download references ACKNOWLEDGEMENTS We thank Drs Peter Iwen and
Paul Fey (University of Nebraska Medical Center, Omaha NE) for their advice and gracious assistance in assaying restricted bacterial species, and for their useful discussions and for
providing various _S. aureus_ strains. This study was funded entirely by departmental funds made available to TM and ST. AUTHOR INFORMATION AUTHORS AND AFFILIATIONS * Department of Pathology
and Microbiology, University of Nebraska Medical Center, 986495 Nebraska Medical Center, Omaha, NE, USA Thomas McDonald, Annika Weber & Steven Tracy * Department of Medical Microbiology
and Immunology, Creighton University, Medical Center, Omaha, NE, USA Kristen M Drescher Authors * Thomas McDonald View author publications You can also search for this author inPubMed
Google Scholar * Kristen M Drescher View author publications You can also search for this author inPubMed Google Scholar * Annika Weber View author publications You can also search for this
author inPubMed Google Scholar * Steven Tracy View author publications You can also search for this author inPubMed Google Scholar CORRESPONDING AUTHOR Correspondence to Thomas McDonald.
RIGHTS AND PERMISSIONS Reprints and permissions ABOUT THIS ARTICLE CITE THIS ARTICLE McDonald, T., Drescher, K., Weber, A. _et al._ Creatinine inhibits bacterial replication. _J Antibiot_
65, 153–156 (2012). https://doi.org/10.1038/ja.2011.131 Download citation * Received: 24 August 2011 * Revised: 31 October 2011 * Accepted: 07 December 2011 * Published: 01 February 2012 *
Issue Date: March 2012 * DOI: https://doi.org/10.1038/ja.2011.131 SHARE THIS ARTICLE Anyone you share the following link with will be able to read this content: Get shareable link Sorry, a
shareable link is not currently available for this article. Copy to clipboard Provided by the Springer Nature SharedIt content-sharing initiative KEYWORDS * antibacterial * antibiotic *
bacteria * creatinine