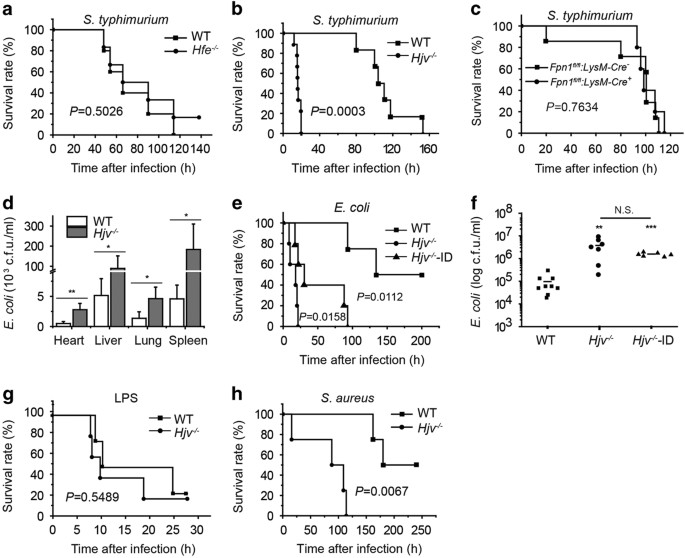
- Select a language for the TTS:
- UK English Female
- UK English Male
- US English Female
- US English Male
- Australian Female
- Australian Male
- Language selected: (auto detect) - EN
Play all audios:
ABSTRACT Hereditary hemochromatosis and iron imbalance are associated with susceptibility to bacterial infection; however, the underlying mechanisms are poorly understood. Here, we performed
_in vivo_ bacterial infection screening using several mouse models of hemochromatosis, including _Hfe_ (_Hfe__−/−_), _hemojuvelin_ (_Hjv__−/−_), and macrophage-specific _ferroportin-1_
(_Fpn1__fl/fl_;_LysM-Cre_+) knockout mice. We found that _Hjv_−/− mice, but not _Hfe__−/−_ or _Fpn1__fl/fl_;_LysM-Cre_+ mice, are highly susceptible to peritoneal infection by both
Gram-negative and Gram-positive bacteria. Interestingly, phagocytic cells in the peritoneum of _Hjv_−/− mice have reduced bacterial clearance, IFN-γ secretion, and nitric oxide production;
in contrast, both cell migration and phagocytosis are normal. Expressing _Hjv_ in RAW264.7 cells increased the level of phosphorylated Stat1 and nitric oxide production. Moreover,
macrophage-specific _Hjv_ knockout mice are susceptible to bacterial infection. Finally, we found that Hjv facilitates the secretion of IFN-γ via the IL-12/Jak2/Stat4 signaling pathway.
Together, these findings reveal a novel protective role of Hjv in the early stages of antimicrobial defense. SIMILAR CONTENT BEING VIEWED BY OTHERS EXTRACELLULAR SQSTM1 MEDIATES BACTERIAL
SEPTIC DEATH IN MICE THROUGH INSULIN RECEPTOR SIGNALLING Article 19 October 2020 GLUTAMATE OXALOACETATE TRANSAMINASE 1 IS DISPENSABLE IN MACROPHAGE DIFFERENTIATION AND ANTI-PATHOGEN RESPONSE
Article Open access 05 July 2024 NUPR1 IS A CRITICAL REPRESSOR OF FERROPTOSIS Article Open access 28 January 2021 INTRODUCTION In the 1960s, researchers first suggested a link between iron
metabolism and the immune system [1]. Since then, many iron-related genes have been found to play a role in immune function. For example, the proteins lactoferrin, hepcidin, and Hfe have all
been found to modulate the host defense against bacterial infection [2–6]. Moreover, the expression of several genes involved in iron metabolism, including hemojuvelin (_HJV_) [7], hepcidin
(_HAMP_) [8], and ferroportin1 (_FPN1_) [9, 10], are modulated during inflammation. Interestingly, a recent case report described a patient with hemochromatosis who died from bacterial
infection [11]. These findings prompted us to investigate the putative role of hemochromatosis-related genes in bacterial infection. We therefore screened several hemochromatosis mouse
models for their immune phenotype and susceptibility to bacterial infection. HJV is a bone morphogenetic protein (BMP) co-receptor that regulates the expression of hepcidin [12]. In humans,
mutations in the _HJV_ gene cause juvenile hemochromatosis [13]. In mice, deleting _Hjv_ expression causes a similar iron-overload phenotype [7, 14]. HJV is also a member of the repulsive
guidance molecule (RGM) family [15]. However, the role of HJV in the immune response is currently unknown. Recent studies suggest that two other RGM family members—RGMa and RGMb—play a role
in immune regulation. For example, RGMa is found in bone marrow-derived dendritic cells, where it modulates T cell responses [16]. RGMb is strongly expressed in macrophages, and increased
IL-6 levels were measured in macrophages isolated from _Rgmb_-knockout mice [17]. Notably, the _Hjv_ gene (also known as _RGMc_) is also expressed in macrophages [17], and studies suggest
that lipopolysaccharide (LPS) stimulation induces organ-specific patterns of _Hjv_ expression [7]. However, whether Hjv plays a role in the immune system’s response to bacterial infection is
currently unknown. Here, we used an acute infection mouse model to investigate the potential immunomodulatory roles of hemochromatosis genes. Our results indicate that Hjv plays a major
role in mediating the host innate immune response to bacterial infection. RESULTS _HJV_-KNOCKOUT MICE HAVE INCREASED SUSCEPTIBILITY TO BACTERIAL INFECTION To investigate the function of
hemochromatosis-related genes in response to acute bacterial infection, we administered an intraperitoneal (i.p.) injection of a lethal dose of the Gram-negative bacterium _Salmonella
typhimurium (S. typhimurium) _[5, 18 ] to the following hemochromatosis mouse models: _Hfe_-knockout (_Hfe__−/−_) mice (Figure 1a), hemojuvelin-knockout (_Hjv__−/−_) mice (Figure 1b), and
macrophage-specific ferroportin1-knockout (_Fpn1__fl/fl_;_LysM-Cre_+) mice (Figure 1c). Although all three models are reported to develop systemic iron overload [9], only the _Hjv__−/−_ mice
were highly susceptible to _S. typhimurium_ infection, reaching 100% mortality within 20 h of infection. Two factors may contribute to the high sensitivity of _Hjv__−/−_ mice to bacterial
infection. First, of these three models of hemochromatosis, _Hjv__−/−_ mice develop the most severe form of iron overload; second, _Hjv__−/−_ mice have the lowest basal hepcidin levels. With
respect to the first factor, iron overload has been shown to facilitate bacterial proliferation [19, 20]. Consistent with this finding, the _Hjv__−/−_ mice had significantly more bacteria
in the major organs and peritoneal fluid compared with wild-type mice (Figure 1d). In order to reduce the tissue iron burden in _Hjv__−/−_ mice to wild-type levels, we fed 4-week-old
_Hjv__−/−_ mice an iron-deficient (ID) diet for four weeks producing _Hjv__−/−_-ID mice, which had comparable iron contents compared with wild-type mice (Supplementary Figures S1A and C).
Injecting _Escherichia coli_ into both _Hjv__−/−_ and _Hjv__−/−_-ID mice caused high mortality (Figure 1e). Given its relatively higher safety compared with _S. typhimurium_, we used _E.
coli_ infection for further experiments. Consistent with increased mortality, the peritoneal cavity of _Hjv__−/−_-ID mice contained higher levels of bacterial colony-forming units (CFUs)
(Figure 1f). Thus, decreasing iron levels to wild-type levels was not sufficient to fully restore bacterial clearance in _Hjv__−/−_ mice; therefore, factors other than iron overload likely
contribute to the susceptibility of _Hjv__−/−_ mice to bacterial infection. Hepcidin, the downstream target of Hjv encoded by the _Hamp_ gene, has been reported to regulate inflammatory
responses in mice [4]. Moreover, hepcidin-knockout mice are sensitive to LPS stimulation [4]. Because hepcidin levels are low in _Hjv__−/−_ mice under basal conditions [7], we hypothesized
that low hepcidin levels may contribute to the high susceptibility of _Hjv__−/−_ mice to bacterial infection. To test this hypothesis, we challenged _Hjv__−/−_ mice with LPS and
D-galactosamine [21] to induce endotoxic shock. We found that the mortality rate was similar between LPS-treated _Hjv__−/−_ mice and LPS-treated wild-type mice (Figure 1g). Interestingly,
LPS stimulation induces hepcidin in _Hjv__−/−_ mice [7], and _Hamp_ mRNA levels were similar between wild-type and _Hjv__−/−_ mice following _E. coli_ infection (Supplementary Figure S2A).
Moreover, serum TNF-α levels (measured 1 h after LPS stimulation) and IL-6 levels (measured 2.5 h after LPS stimulation) were similar between _Hjv__−/−_ and wild-type mice (Supplementary
Figure S2B and C). Taken together, these results suggest that reduced hepcidin expression does not contribute significantly to the increased mortality of _Hjv__−/−_ mice in response to
bacterial infection. To determine whether _Hjv__−/−_ mice are also susceptible to infection by Gram-positive bacterium, we gave _Hjv__−/−_ and wild-type mice a lethal i.p. dose of the
Gram-positive bacterium _Staphylococcus aureus (S. aureus)_ and found considerably higher mortality in the infected _Hjv__−/−_ mice (Figure 1h). Thus, our acute lethal infection experiments
indicate that _Hjv__−/−_ mice are highly susceptible to infection by both Gram-negative and Gram-positive bacteria, suggesting that Hjv may play an essential role in the host defense against
bacterial infection. IMPAIRED MICROBIAL CLEARANCE IN _HJV__−/−_ MACROPHAGES AND NEUTROPHILS Our findings suggest that bacterial clearance is impaired in _Hjv__−/−_ mice. However, bacteremia
induced by an intravenous (i.v.) injection of _E. coli_ [22, 23] caused similar mortality between _Hjv__−/−_ and wild-type mice (Figure 2a). Houghton _et al._ [18] reported that impaired
macrophage function within the peritoneal cavity can result in bacterial dissemination to other sites. Thus, local defense processes are partially independent of systemic protection and may
play an essential role in host survival. Based on these findings, we hypothesized that the local defense process is impaired in the peritoneal cavity of _Hjv__−/−_ mice. We therefore
measured the immune response in the peritoneal cavity following bacterial infection. In the early stages of bacterial infection, macrophages and neutrophils mediate bacterial clearance [24].
To determine the relative proportions of these two cell types in infected _Hjv__−/−_ mice, we isolated peritoneal cells, stained the cells with F4/80 and Gr-1, and performed flow cytometry
[25]. Our analysis revealed that 6–12 h after bacterial infection, neutrophils (F4/80−, Gr-1+) and macrophages (F4/80+) comprised ~80% and 10%, respectively, of the total population of
phagocytes; after 24 h, the percentage of neutrophils decreased to ~50%, and the percentage of macrophages increased to ~40% (Figure 2b). Interestingly, the relative proportions of both cell
types were similar between _Hjv__−/−_ and wild-type mice following bacterial infection, indicating that the migration of macrophages and neutrophils to the peritoneal cavity is intact in
_Hjv__−/−_ mice. Next, we examined further the phagocytic function of peritoneal cells. Primary peritoneal macrophages were obtained from _Hjv__−/−_ and wild-type mice by peritoneal lavage
with Thioglycollate medium [26]. We then incubated the cells with Alexa Fluor 488 conjugated heat-killed _E. coli_ and measured phagocytosis using flow cytometry. We found no difference
between _Hjv__−/−_ macrophages and wild-type macrophages with respect to phagocytosis ratio (Figure 2c) or mean fluorescence intensity (Figure 2d), suggesting that _Hjv__−/−_ macrophages
have normal phagocytic activity. Despite normal phagocytosis measured _in vitro_, we observed more GFP fluorescence in peritoneal cells obtained from _Hjv__−/−_ mice 12 h after infection
with GFP-expressing _E. coli_ compared with wild-type mice (Figure 2e). This stronger immune response in _Hjv__−/−_ mice may be due to bacterial overload in the phagocytes; on the other
hand, bacteria in the peritoneal cavity were over-proliferated in _Hjv__−/−_ mice (Figure 1f). Taken together, these data suggest that although more bacteria are engulfed by _Hjv__−/−_
phagocytes, they are not efficiently killed and/or degraded. REDUCED IFN-Γ EXPRESSION IN _HJV__−/−_ NEUTROPHILS AND MACROPHAGES To test for a possible immune deficiency in _Hjv__−/−_ mice,
we measured the levels of circulating inflammatory cytokines, which generally reflect the presence of an ongoing immune response and can be used to monitor bacteria clearance. We therefore
collected serum samples after infecting mice with of _E. coli_ (2×108 CFU) by i.p. injection; serum was collected 1, 2.5, and 6 h after infection for measuring TNF-α, IL-6 and IFN-γ levels,
respectively. Both TNF-α and IL-6 levels were approximately twofold higher in the serum of _Hjv__−/−_ mice compared with wild-type mice; in contrast, IFN-γ levels were significantly lower in
the _Hjv__−/−_ mice (Figure 3a). IFN-γ is secreted primarily by NK (natural killer) cells and T cells [27]. However, in our acute infection models, the mice died within 24 h, well before NK
cells and T cells are activated [27]. We first confirmed that splenocytes isolated from infected _Hjv__−/−_ mice and wild-type mice have extremely low IFN-γ secretion (Supplementary Figure
S3A). We then examined several cell types in the peritoneal cavity. Macrophages and neutrophils are potential sources of IFN-γ in the early stage of infection [28, 29]. Thus, a lack of IFN-γ
autocrine secretion may underlie the impaired killing capacity of _Hjv__−/−_ macrophages and neutrophils. Indeed, the myeloid lineage cell fraction in the peritoneal cavity contained
considerable numbers of IFN-γ positive (IFN-γ+) cells (Figure 3b). Six hours after _E. coli_ infection, 17.5 and 4% of wild-type and _Hjv__−/−_ peritoneal myeloid lineage cells were IFN-γ+
(Figure 3b). Twelve hours after infection, the percentage of IFN-γ+ cells decreased in both genotypes; however, the percentage of IFN-γ+ cell was still 2-fold higher in the wild-type mice
than in the _Hjv__−/−_ mice. We then examined the identity of the IFN-γ+ cells 6, 12, and 24 h after infection. For wild-type peritoneal cells, 6 h after infection, 75% and 25% of the IFN-γ+
cells were neutrophils (that is, Gr-1+, F4/80− cells) and macrophages (that is, F4/80+ cells), respectively; however, 12 h after infection, the percentage of IFN-γ+ neutrophils cells
decreased to only 13%, and the percentage of macrophages increased to 87%. Moreover, in wild-type mice we detected only a very small proportion of T cells (CD8b+) and NK cells (NK1.1+)
within the peritoneal population of IFN-γ+ cells 12 h after infection (Supplementary Figure S3B); thus, phagocytes—rather than NK cells or T cells—are the principal source of IFN-γ in the
early stages of peritoneal infection. Recent studies found that cellular iron content can affect macrophage function [19, 30]. In _Hjv__−/−_ mice, splenic iron content is low (Supplementary
Figure S1A) because ferroportin retained in the plasma membrane exports more iron out of these macrophages in the spleen. To exclude this effect, we generated macrophage-specific
_Hjv_-knockout (_Hjv__fl/fl_;_LysM-Cre_+) mice; iron content in the major organs and macrophages of these mice was normal (Supplementary Figure S1B and D), and _E. coli_-induced mortality
was similar between _Hjv__fl/fl_;_LysM-Cre_+ mice and _Hjv_−/−-ID mice (compare Figure 3c with Figure 1e). Notably, treating _Hjv__fl/fl_;_LysM-Cre_+ mice with IFN-γ (10 units per mouse)
[31, 32 ] significantly improved survival following _E. coli_ infection (Figure 3d). Next, we examined the specific functions of macrophages and neutrophils. We performed adoptive transfer
experiments by injecting 2×106 wild-type neutrophils or macrophages into the peritoneal cavity of _Hjv_−/− mice; 4 h later, these mice were challenged with a lethal dose of _E. coli_ (2×108
CFU). Although transferring wild-type neutrophils conferred no protection to these mice (Figure 3e), transferring wild-type macrophages conferred a significant protective effect against
bacterial infection compared with _Hjv_−/− macrophages (Figure 3f). Thus, impaired macrophage function, possibly due to reduced IFN-γ production and/or secretion, appears to play a key role
in the susceptibility of _Hjv__−/−_ mice to bacterial infection. DECREASED IFN-Γ SECRETION IN _HJV__−/−_ MACROPHAGES REDUCES THEIR NITRIC OXIDE PRODUCTION AND KILLING CAPACITY IFN-γ is a
critical mediator of protective immunity against both viral and bacterial infections; consistent with this role, _IFN-γ_-knockout mice are highly susceptible to bacterial infection [33, 34].
Specifically, peritoneal cells produce IFN-γ and are essential for the survival of infected mice, and bacterial clearance by these cells is impaired when IFN-γ secretion is reduced [35]. To
further investigate the function of IFN-γ release by macrophages in the early stages of bacterial infection, we isolated and cultured peritoneal macrophages, then stimulated the cells with
_E. coli_ that was heat-killed to prevent lethality due to uncontrolled bacterial replication, thereby mimicking infection _in vivo_. Twenty-four hours after stimulation, both _Hjv__−/−_ and
_Hjv__fl/fl_;_LysM-Cre_+ macrophages secreted significantly fewer amounts of IFN-γ compared with wild-type macrophages (Figure 4a); in contrast, both TNF-α and IL-6 were produced at
wild-type levels (Supplementary Figure S4A and B). In macrophages, IFN-γ activates the enzyme iNOS (inducible nitric oxide synthase), thereby increasing nitric oxide (NO) production in these
cells [36, 37]. We used thioglycollate-elicited macrophages as our primary cell model, as NO synthesis is robust in these cells [38, 39]. After cells were stimulated with heat-killed _E.
coli_, both phosphorylated Stat1 (pStat1, an upstream activator of iNOS) and iNOS expression were significantly lower in _Hjv__−/−_ macrophages compared with wild-type cells (Figure 4b and
c). Moreover, the amount of NO released was significantly lower in both _Hjv__−/−_ and _Hjv__fl/fl_;_LysM-Cre_+ macrophages compared with wild-type cells (Figure 4d). Applying exogenous
IFN-γ (100 ng ml−1) to _Hjv__−/−_ macrophages restored both their pStat1 levels and NO secretion (Figure 4e and f). Macrophages utilize NO production to confer protection from invading
pathogens [40]. We, therefore, collected thioglycollate-elicited macrophages from both _Hjv__fl/fl_;_LysM-Cre_+ mice and _Hjv__fl/fl_;_LysM-Cre__−_ control mice. After 90 min in culture, the
bacterial killing capacity of the _Hjv__fl/fl_;_LysM-Cre_+ macrophages was significantly decreased compared with _Hjv__fl/fl_;_LysM-Cre__−_ controls (that is, the _Hjv__fl/fl_;_LysM-Cre_+
macrophages contained significantly more CFUs than the control cells) (Figure 4g). Notably, pretreating both _Hjv__fl/fl_;_LysM-Cre_+ and control macrophages with IFN-γ significantly
increased bacterial killing capacity, thereby rescuing the phenotype in _Hjv__fl/fl_;_LysM-Cre_+ macrophages. Moreover, co-treating cells with IFN-γ and an IFN-γ neutralizing antibody
(NeuAb) significantly reduced the bacterial killing capacity of both _Hjv__fl/fl_;_LysM-Cre_+ and control macrophages (Figure 4g). To investigate other possible mechanisms involved in the
killing capacity of macrophages, we also measured the level of reactive oxygen species (ROS) within the cells. However, we found no significant difference between _Hjv__−/−_ and wild-type
macrophages (Figure 4h). We therefore conclude that impaired IFN-γ production in _Hjv_−/− macrophages plays a principal role in the reduced killing capacity of these cells, possibly
explaining the unrestricted growth of bacteria in the peritoneal cavity and major organs of _Hjv_−/− mice. HJV IS UPREGULATED AND FACILITATES THE NO CASCADE IN INFECTED MACROPHAGES We found
that elicited wild-type macrophages treated with heat-killed _E. coli_ had significantly increased levels of _Hjv_ mRNA (Figure 5a); in contrast, and consistent with a previous report [7],
_Hjv_ expression was decreased in the liver (Supplementary Figure S5). These results suggest that Hjv may play a regulatory role during infection. Interestingly, stimulating
_Hjv_-transfected RAW264.7 cells (a murine macrophage cell line) with either LPS or heat-killed _E. coli_ led to significantly increased NO production compared with control cells and cells
transfected with an empty vector (Figure 5b). Consistent with these results, both iNOS expression (Figure 5c) and pStat1 levels (Figure 5d) were also increased. These results suggest that
Hjv plays a key role in both iNOS expression and NO production in macrophages. Given that IFN-γ treatment rescued iNOS signaling (Figure 4e, f), Hjv likely modulates the iNOS cascade
primarily via IFN-γ signaling. IL-12/STAT4 SIGNALING IS IMPAIRED IN _HJV__−/−_ MACROPHAGES Hjv is a major regulator of Bmp/Smad signaling [41]. However, whether Bmp/Smad signaling is altered
in _Hjv__−/−_ macrophages and/or whether crosstalk exists between the inflammatory response and Bmp/Smad signaling is not currently known. Bmp6 can induce NO production in macrophages
independent of Smad [42], and inflammatory stimuli can reduce Smad1/5/8 phosphorylation in macrophages [43]. However, we found similar levels of phosphorylated Smad1/5/8 levels between
_Hjv__−/−_ and wild-type macrophages following stimulation with heat-killed _E. coli_ (Supplementary Figure S6A). When we treated wild-type macrophages with LDN193189, a classic inhibitor of
Bmp/Smad signaling, [44] pSmad1/5/8 levels were significantly reduced (Supplementary Figure S6A), whereas iNOS expression was increased (Supplementary Figure S6B). Moreover, the effect of
heat-killed _E. coli_ on Bmp/Smad signaling was unaffected by Hjv deletion (Supplementary Figure S6A). Together, these results indicate that Bmp/Smad signaling is likely not altered in
_Hjv__−/−_ macrophages during bacterial infection. Both IL-18 [45] and IL-12 [46] are key regulators of IFN-γ secretion. Therefore, to investigate the possible mechanism by which Hjv
regulates IFN-γ, we collected serum from _Hjv__−/−_ and wild-type mice following _E. coli_ infection. We found no significant difference between _Hjv__−/−_ and wild-type mice with respect to
either IL-12 (Figure 6a) or IL-18 (Figure 6b). We therefore hypothesized that the capacity for IL-12 and IL-18 to induce IFN-γ might be reduced in _Hjv__−/−_ mice. To test this hypothesis,
we examined the signaling pathways downstream of IL-12 and IL-18. Previous studies showed that IL-18 regulates IFN-γ via the MAP kinase (MAPK) pathway, [47] and IL-12 drives the
phosphorylation of Stat4 to induce IFN-γ expression. [48] To investigate the role of these two pathways, we measured phosphorylated MAPK proteins and phosphorylated Stat4 in macrophages
stimulated with heat-killed _E. coli_. We found no defect in _Hjv__−/−_ macrophages with respect to p38 (Figure 4c), Erk1/2, or Jnk phosphorylation (Figure 6c). However, _E. coli_ stimulated
phosphorylation of Stat4 was significantly impaired in _Hjv__−/−_ macrophages compared with wild-type macrophages (Figure 6d). Consistent with reduced Stat4 phosphorylation, Jak2
phosphorylation was also reduced in _Hjv__−/−_ macrophages compared with wild-type cells (Figure 6e). Finally, we performed _in vitro_ co-immunoprecipitation experiments using HEK293 cells
co-transfected with Flag-tagged HJV and Myc-tagged IL-12RB1 (the beta-1 subunit of the IL-12 receptor). Although weakly pulled down by IL-12RB1-Myc, HJV-Flag was highly effective at pulling
down IL-12RB1-Myc (Figure 6f), suggesting that HJV may play a direct role in IL-12 signaling. Taken together, these results suggest that HJV may regulate IFN-γ in macrophages via the
IL-12/Jak2/Stat4 pathway. DISCUSSION A clear relationship between hemochromatosis and infection has been established by several groups [49–52]. The majority of these results suggest that
both HFE and Hepcidin (encoded by the _HFE_ and _HAMP_ genes, respectively) play a protective role against bacterial infection in human and mouse. Here, we performed a novel screen using
several hemochromatosis mouse models to investigate the mechanisms that underlie the putative link between iron metabolism and immunity. Importantly, our _in vivo_ screening strategy
differed from previous studies [5, 18] in that we focused on the acute infection phase. Thus, our bacterial dose was based on the lethal dose established for modeling severe peritonitis in
129/svj mice [18]. Of the three hemochromatosis mouse models in our initial screen, we found that only _Hjv__−/−_ mice were highly susceptible to bacterial infection, and we excluded iron
burden as the main contributing factor using iron-deficient _Hjv__−/−_ (_Hjv__−/−_-ID) mice. However, under standard dietary conditions, splenic iron content is lower in _Hjv__−/−_ mice than
in wild-type mice [7], and placing _Hjv__−/−_ mice on an iron-deficient diet lowered iron content in the macrophages even further (Supplementary Figure S1A). These factors complicate the
phenotype of _Hjv__−/−_ mice and may have affected survival. Therefore, after we identified which specific immune cells (that is, macrophages and neutrophils) are functionally impaired in
_Hjv__−/−_ mice, we performed survival experiments using _Hjv__fl/fl_;_LysM-Cre_+ mice, in which Hjv expression is deleted selectively in macrophages and neutrophils. Our results revealed
that _Hjv__fl/fl_;_LysM-Cre_+ mice have a survival profile similar to _Hjv__−/−_-ID mice. Given that the iron content in the major organs and macrophages of _Hjv__fl/fl_;_LysM-Cre_+ mice was
similar to wild-type littermates (Supplementary Figure S1B and C), we conclude that iron burden does not play a major role in the high susceptibility of _Hjv__−/−_ mice to bacterial
infection. We observed significantly reduced levels of circulating IFN-γ in _Hjv__−/−_ mice 6 h after bacterial infection. IFN-γ is primarily secreted by T helper type 1 (Th1) cells [53] and
NK cells [54]. However, given the time frame of the increased IFN-γ levels (that is, within 6 h), the principal source of IFN-γ during the early stages of infection is likely not Th1 or NK
cells. Interestingly, peritoneal macrophages and neutrophils have been reported as a source of IFN-γ [55–58], although this remains controversial [59]; nevertheless, it is worth noting that
Schleicher _et al._ [59] treated peritoneal cells with IL-12, IL-18, and LPS for 72 h, which differs from our bacterial infection model (6–24 h). A recent study in mice also found that Gr-1+
cells produce IFN-γ in the early stages of _Streptococcus_ infection, and these cells are responsible for promoting the survival of infected mice [35]. We therefore conclude that peritoneal
phagocytes secrete IFN-γ, thereby self-activating via an autocrine pathway, providing an essential step in fighting peritoneal infection and improving survival. Our results indicate that
_Hjv_ deletion affects the IL-12/Jak2/Stat4 signaling pathway, but not the IL-18/MAPK pathway. Nevertheless, additional research is warranted in order to better understand the underlying
mechanisms. In conclusion, we identified a novel role for HJV in mediating bacterial clearance via macrophages. Our findings indicate that HJV may serve as a potential target for boosting
protection against bacterial peritoneal infection, thereby improving clinical outcome. MATERIALS AND METHODS MICE _Hfe__−/−__, Hjv__−/−__, Fpn1__floxflox_, _Hjv__flox/flox_ and wild-type
mice were maintained on the 129S6/SvEvTac background and were housed in a specific pathogen-free animal facility [9, 14, 60, 61]. _Fpn1_ knockout in macrophages (_Fpn1__fl/fl_;_LysM-Cre_+)
was achieved by crossing _LysM_-_Cre_ mice [62] with _Fpn1__floxflox_ mice, and _Hjv_ knockout in macrophages (_Hjv__fl/fl_;_LysM-Cre_+) was achieved by crossing _LysM-Cre_ mice [62] with
_Hjv__flox/flox_ mice. LysM-Cre mice were maintained as heterozygotes. All animal experiments were approved and performed in accordance with guidelines established by the Institutional
Animal Care and Use Committee of the School of Medicine, Zhejiang University. _IN VIVO_ INFECTION MODELS AND BACTERIAL GROWTH MEASUREMENTS Mice were infected with various bacterial species
via i.p. or i.v. injection in order to induce peritonitis and bacteremia, respectively. _S. typhimurium_, _E. coli_ and _S. aureus_ were grown in LB and isolated on LB agar plates. For the
IFN-γ rescue experiments, 10 units of IFN-γ in saline (or saline alone) were subcutaneously injected into the right flank of the mice, immediately followed by an i.p. injection of _E. coli_
TOP10 bacteria, which carries the GFP gene on the Puc19 plasmid [63]. Organ/peritoneal cavity colony-forming units (CFUs) were determined by plating dilutions of organ homogenates or
peritoneal lavage fluid on LB plates. DIETARY INDUCTION OF IRON DEFICIENCY To induce iron deficiency, 4-week-old mice were fed a diet containing reduced iron content (0.9 mg Fe kg−1,
AIN-76A-diet, D08080402, Research Diets, Inc., New Brunswick, NJ, USA) for 4 weeks. Standard diet contained 50 mg Fe kg−1, (AIN-76A-diet, D08080401, Research Diets, Inc.). PHAGOCYTOSIS ASSAY
AND MACROPHAGE KILLING ASSAYS For the phagocytosis assay, thioglycollate-stimulated peritoneal macrophages were incubated with Alexa Fluor 488-conjugated heat-killed _E. coli_ (Molecular
Probes) for 1 h. Extracellular fluorescence was then quenched by adding 100 μl of trypan blue [64]. Cells were then washed in cold PBS and examined using fluorescence microscopy or flow
cytometry (FACS analysis). For the killing assay, thioglycollate-stimulated peritoneal macrophages were incubated with TOP10 _E. coli_ for 20 min. The macrophages were then washed with
phosphate-buffered saline (PBS) to remove the bacteria, incubated in 100 μg ml−1 gentamycin in DMEM for 20 min (to kill extracellular bacteria), and then incubated for an additional 120 min
in 25 μg ml−1 gentamycin in DMEM. CFUs were then measured from macrophage lysates [40, 65]. In some experiments, the cells were pretreated for 12 h with IFN-γ (Peprotech) in the presence or
absence of IFN-γ-neutralizing antibody (NeuAb; bp0055, Bio X Cell) prior to adding the bacteria. ELISA ELISA kits for measuring IL-6, TNF-α and IFN-γ were purchased from R&D Systems.
ELISA kits for measuring IL-12 and IL-18 were purchased from Pierce. Sera were collected from _E. coli_-infected wild-type and _Hjv__−/−_ mice at indicated time. Briefly, 50 μl serum, blank
or standard were added in 96-well plate, incubated at room temperature for 2 h, washed by wash buffer for five times. Then, 100 μl conjugated antibodies were incubated at room temperature
for 2 h, washed by wash buffer for five times. TMB mix (100 μl) was added into wells for 30 min, and stop solution was then added to stop the reaction. OD values were read at 450 nm by
Microplate reader (Molecular Devices, Sunnyvale, CA , USA). ANTIBODIES Primary antibodies: rabbit anti-pStat1 (1:1000, Cell Signaling Technology [CST, Boston, MA, USA]), rabbit anti-Stat1
(1:1000, CST), rabbit anti-pErk1/2 (1:1000, CST), rabbit anti-Erk1/2 (1:1000, CST), mouse anti-pJnk (1:1000, CST), rabbit anti-Jnk (1:1000, CST), rabbit anti-pStat4 (1:500, Santa Cruz (Santa
Cruz, CA, USA)), rabbit anti-Stat4 (1:1000, CST), rabbit anti-pJak2 (1:500, CST), rabbit anti-Jak2 (1:1000, CST), rabbit anti-pSmad1/5/8 (1:1000, CST), rabbit anti-Smad1 (1:1000, CST),
rabbit anti-pSmad3 (1:1000, CST), rabbit anti-Smad3 (1:1000, CST), rabbit anti-FLAG (1:1000, CST), mouse anti-Myc (1:1000, CST), or mouse anti-β-actin (Sigma, San francisco, CA, USA).
Secondary antibodies: peroxidase-conjugated goat anti-Rabbit or Goat anti mouse IgG (Proteintech (Chicago, IL,USA), 1:2000). WESTERN BLOT ANALYSIS Western blot was perform as previously
described [9]. Briefly, cells/tissues were lysed by RIPA buffer (Beyotime, Shanghai, China) with protease inhibitor cocktail (Sigma) for 10 min; Sonicate for 10 s to complete cell lysis and
shear DNA followed by adding loading buffer (containing 5% β-Me). Then samples were heated to 95–100 °C for 5 min (alternatively 37 °C 30 min for membrane protein detection), cool on ice and
microcentrifuge for 5 min. Samples were loaded onto SDS-PAGE gel and then electrotransfer to PVDF membrane (Bio-Rad, Hercules, CA, USA). The transferred membrane was incubated in blocking
buffer (5% skim milk in TBST) for 1 h followed by incubation with primary antibody overnight at 4 °C. Membrane was washed three times for 10 min each with TBST, and incubated with HRP-linked
secondary antibody for 1 h at room temperature. Wash three times for 10 min each with TBST. After incubation with ECL (Pierce (Rockford, IL, USA), Thermo (Waltham, MA, USA)) mixture for 5
min, wrap the membrane in plastic and expose to X-ray film. Co-IP experiments were performed using anti-Flag (1:50, CST) and anti-Myc (1:1000, CST) antibodies. REAL-TIME QUANTITATIVE PCR
Total RNA was isolated from peritoneal macrophages or liver tissue using Trizol (Invitrogen, Carlsbad, CA, USA). Real-time PCR was then performed using two-step quantitative real-time PCR
with the primer pairs listed in Supplementary Table S1. The expression level of each target gene was normalized to the corresponding _β-actin_ mRNA level. EXPRESSION OF _HJV_ IN RAW264.7
MACROPHAGES _Hjv_-expressing constructs were cloned into the pCMV-3Tag-3A vector and transfected into RAW264.7 cells using the FuGENE HD transfection reagent (Promega, Madison, WI, USA).
QUANTIFICATION OF NITRIC OXIDE (NO) AND ROS NO concentration was measured using the Griess assay [66]. 50 μl cell medium, blank or standard control were added into a 96-well plate, followed
by adding 50 μl Griess reagent I and II, incubated at room temperature for 10 min, OD values were read at 540 nm by Microplate reader (Molecular Devices). Intracellular reactive oxygen
species were measured using the dichloro-dihydro-fluorescein diacetate (DCFH-DA) method [67]. HK-_E. coli_-stimulated macrophages in 6-well plate were incubated in serum-free DMEM-containing
10 μM DCFH-DA for 20 min at 37 °C in cell culture hood. Cells were then detached by trypsin digestion and followed by FACS analysis at the excitation wavelength of 488 nm and emission
wavelength of 525 nm. STATISTICAL ANALYSIS All summary data are presented as the mean±s.e.m. The log-rank test or Mann–Whitney _U_-test was used to analyze the Kaplan–Meier survival curves.
The Student’s _t_-test was used to compare two groups, and an ANOVA was used to compare multiple groups. Differences with a _P_-value<0.05 were considered significant. REFERENCES * Bullen
JJ, Rogers HJ . Bacterial iron metabolism and immunity to Pasteurella septica and _Escherichia coli_. _Nature_ 1969; 224: 380–382. Article CAS Google Scholar * Farnaud S, Evans RW .
Lactoferrin--a multifunctional protein with antimicrobial properties. _Mol Immunol_ 2003; 40: 395–405. Article CAS Google Scholar * Park CH, Valore EV, Waring AJ, Ganz T . Hepcidin, a
urinary antimicrobial peptide synthesized in the liver. _J Biol Chem_ 2001; 276: 7806–7810. Article CAS Google Scholar * De Domenico I, Zhang TY, Koening CL et al. Hepcidin mediates
transcriptional changes that modulate acute cytokine-induced inflammatory responses in mice. _J Clin Invest_ 2010; 120: 2395–2405. Article CAS Google Scholar * Flo TH, Smith KD, Sato S et
al. Lipocalin 2 mediates an innate immune response to bacterial infection by sequestrating iron. _Nature_ 2004; 432: 917–921. Article CAS Google Scholar * Nairz M, Theurl I, Schroll A et
al. Absence of functional Hfe protects mice from invasive Salmonella enterica serovar Typhimurium infection via induction of lipocalin-2. _Blood_ 2009; 114: 3642–3651. Article CAS Google
Scholar * Niederkofler V, Salie R, Arber S . Hemojuvelin is essential for dietary iron sensing, and its mutation leads to severe iron overload. _J Clin Invest_ 2005; 115: 2180–2186. Article
CAS Google Scholar * Armitage AE, Eddowes LA, Gileadi U et al. Hepcidin regulation by innate immune and infectious stimuli. _Blood_ 2011; 118: 4129–4139. Article Google Scholar * Zhang
Z, Zhang F, An P et al. Ferroportin1 deficiency in mouse macrophages impairs iron homeostasis and inflammatory responses. _Blood_ 2011; 118: 1912–1922. Article CAS Google Scholar * Naz
N, Malik IA, Sheikh N et al. Ferroportin-1 is a 'nuclear'-negative acute-phase protein in rat liver: a comparison with other iron-transport proteins. _Lab Invest_ 2012; 92:
842–856. Article CAS Google Scholar * Frank KM, Schneewind O, Shieh WJ . Investigation of a researcher's death due to septicemic plague. _N Engl J Med_ 2011; 364: 2563–2564. Article
CAS Google Scholar * Andriopoulos B Jr, Corradini E, Xia Y et al. BMP6 is a key endogenous regulator of hepcidin expression and iron metabolism. _Nat Genet_ 2009; 41: 482–487. Article
CAS Google Scholar * Papanikolaou G, Samuels ME, Ludwig EH et al. Mutations in HFE2 cause iron overload in chromosome 1q-linked juvenile hemochromatosis. _Nat Genet_ 2004; 36: 77–82.
Article CAS Google Scholar * Huang FW, Pinkus JL, Pinkus GS, Fleming MD, Andrews NC . A mouse model of juvenile hemochromatosis. _J Clin Invest_ 2005; 115: 2187–2191. Article CAS Google
Scholar * Corradini E, Babitt JL, Lin HY . The RGM/DRAGON family of BMP co-receptors. _Cytokine Growth Factor Rev_ 2009; 20: 389–398. Article CAS Google Scholar * Muramatsu R, Kubo T,
Mori M et al. RGMa modulates T cell responses and is involved in autoimmune encephalomyelitis. _Nat Med_ 2011; 17: 488–494. Article CAS Google Scholar * Xia Y, Cortez-Retamozo V,
Niederkofler V et al. Dragon (repulsive guidance molecule b) inhibits IL-6 expression in macrophages. _J Immunol_ 2011; 186: 1369–1376. Article CAS Google Scholar * Houghton AM, Hartzell
WO, Robbins CS, Gomis-Ruth FX, Shapiro SD . Macrophage elastase kills bacteria within murine macrophages. _Nature_ 2009; 460: 637–641. Article CAS Google Scholar * Paradkar PN, De
Domenico I, Durchfort N, Zohn I, Kaplan J, Ward DM . Iron depletion limits intracellular bacterial growth in macrophages. _Blood_ 2008; 112: 866–874. Article CAS Google Scholar *
Sunder-Plassmann G, Patruta SI, Horl WH . Pathobiology of the role of iron in infection. _Am J Kidney Dis_ 1999; 34: S25–S29. Article CAS Google Scholar * Chang M, Jin W, Sun SC . Peli1
facilitates TRIF-dependent Toll-like receptor signaling and proinflammatory cytokine production. _Nat Immunol_ 2009; 10: 1089–1095. Article CAS Google Scholar * Starnes HF Jr, Pearce MK,
Tewari A, Yim JH, Zou JC, Abrams JS . Anti-IL-6 monoclonal antibodies protect against lethal _Escherichia coli_ infection and lethal tumor necrosis factor-alpha challenge in mice. _J
Immunol_ 1990; 145: 4185–4191. CAS PubMed Google Scholar * Haziot A, Ferrero E, Kontgen F et al. Resistance to endotoxin shock and reduced dissemination of gram-negative bacteria in
CD14-deficient mice. _Immunity_ 1996; 4: 407–414. Article CAS Google Scholar * Dale DC, Boxer L, Liles WC . The phagocytes: neutrophils and monocytes. _Blood_ 2008; 112: 935–945. Article
CAS Google Scholar * Henderson RB, Hobbs JA, Mathies M, Hogg N . Rapid recruitment of inflammatory monocytes is independent of neutrophil migration. _Blood_ 2003; 102: 328–335. Article
CAS Google Scholar * Keisari Y, Braun L, Flescher E . The oxidative burst and related phenomena in mouse macrophages elicited by different sterile inflammatory stimuli. _Immunobiology_
1983; 165: 78–89. Article CAS Google Scholar * Schroder K, Hertzog PJ, Ravasi T, Hume DA . Interferon-gamma: an overview of signals, mechanisms and functions. _J Leukoc Biol_ 2004; 75:
163–189. Article CAS Google Scholar * Gessani S, Belardelli F . IFN-gamma expression in macrophages and its possible biological significance. _Cytokine Growth Factor Rev_ 1998; 9:
117–123. Article CAS Google Scholar * Yeaman GR, Collins JE, Currie JK, Guyre PM, Wira CR, Fanger MW . IFN-gamma is produced by polymorphonuclear neutrophils in human uterine endometrium
and by cultured peripheral blood polymorphonuclear neutrophils. _J Immunol_ 1998; 160: 5145–5153. CAS PubMed Google Scholar * Cairo G, Recalcati S, Mantovani A, Locati M . Iron
trafficking and metabolism in macrophages: contribution to the polarized phenotype. _Trends Immunol_ 2011; 32: 241–247. Article CAS Google Scholar * Gennari R, Alexander JW, Eaves-Pyles T
. IFN-gamma decreases translocation and improves survival following transfusion and thermal injury. _J Surg Res_ 1994; 56: 530–536. Article CAS Google Scholar * Miles RH, Paxton TP,
Dries DJ, Gamelli RL . Interferon-gamma increases mortality following cecal ligation and puncture. _J Trauma_ 1994; 36: 607–611. Article CAS Google Scholar * Dalton DK, Pitts-Meek S,
Keshav S, Figari IS, Bradley A, Stewart TA . Multiple defects of immune cell function in mice with disrupted interferon-gamma genes. _Science_ 1993; 259: 1739–1742. Article CAS Google
Scholar * Simmons CP, Goncalves NS, Ghaem-Maghami M et al. Impaired resistance and enhanced pathology during infection with a noninvasive, attaching-effacing enteric bacterial pathogen,
Citrobacter rodentium, in mice lacking IL-12 or IFN-gamma. _J Immunol_ 2002; 168: 1804–1812. Article CAS Google Scholar * Matsumura T, Ato M, Ikebe T, Ohnishi M, Watanabe H, Kobayashi K .
Interferon-gamma-producing immature myeloid cells confer protection against severe invasive group A Streptococcus infections. _Nat Commun_ 2012; 3: 678. Article Google Scholar * Karupiah
G, Xie QW, Buller RM, Nathan C, Duarte C, MacMicking JD . Inhibition of viral replication by interferon-gamma-induced nitric oxide synthase. _Science_ 1993; 261: 1445–1448. Article CAS
Google Scholar * Xie QW, Whisnant R, Nathan C . Promoter of the mouse gene encoding calcium-independent nitric oxide synthase confers inducibility by interferon gamma and bacterial
lipopolysaccharide. _J Exp Med_ 1993; 177: 1779–1784. Article CAS Google Scholar * Jun CD, Choi BM, Hoon R et al. Synergistic cooperation between phorbol ester and IFN-gamma for induction
of nitric oxide synthesis in murine peritoneal macrophages. _J Immunol_ 1994; 153: 3684–3690. CAS PubMed Google Scholar * Zhang X, Alley EW, Russell SW, Morrison DC . Necessity and
sufficiency of beta interferon for nitric oxide production in mouse peritoneal macrophages. _Infect Immun_ 1994; 62: 33–40. CAS PubMed PubMed Central Google Scholar * Vazquez-Torres A,
Jones-Carson J, Mastroeni P, Ischiropoulos H, Fang FC . Antimicrobial actions of the NADPH phagocyte oxidase and inducible nitric oxide synthase in experimental salmonellosis. I. Effects on
microbial killing by activated peritoneal macrophages in vitro. _J Exp Med_ 2000; 192: 227–236. Article CAS Google Scholar * Babitt JL, Huang FW, Wrighting DM et al. Bone morphogenetic
protein signaling by hemojuvelin regulates hepcidin expression. _Nat Genet_ 2006; 38: 531–539. Article CAS Google Scholar * Kwon SJ, Lee GT, Lee JH, Kim WJ, Kim IY . Bone morphogenetic
protein-6 induces the expression of inducible nitric oxide synthase in macrophages. _Immunology_ 2009; 128: e758–e765. Article Google Scholar * Wu X, Yung LM, Cheng WH et al. Hepcidin
regulation by BMP signaling in macrophages is lipopolysaccharide dependent. _PLoS ONE_ 2012; 7: e44622. Article CAS Google Scholar * Yu PB, Deng DY, Lai CS et al. BMP type I receptor
inhibition reduces heterotopic [corrected] ossification. _Nat Med_ 2008; 14: 1363–1369. Article CAS Google Scholar * Dinarello CA . Interleukin-18, a proinflammatory cytokine. _Eur
Cytokine Netw_ 2000; 11: 483–486. CAS PubMed Google Scholar * Magram J, Connaughton SE, Warrier RR et al. IL-12-deficient mice are defective in IFN gamma production and type 1 cytokine
responses. _Immunity_ 1996; 4: 471–481. Article CAS Google Scholar * Kalina U, Kauschat D, Koyama N et al. IL-18 activates STAT3 in the natural killer cell line 92, augments cytotoxic
activity, and mediates IFN-gamma production by the stress kinase p38 and by the extracellular regulated kinases p44erk-1 and p42erk-21. _J Immunol_ 2000; 165: 1307–1313. Article CAS Google
Scholar * Nakahira M, Ahn HJ, Park WR et al. Synergy of IL-12 and IL-18 for IFN-gamma gene expression: IL-12-induced STAT4 contributes to IFN-gamma promoter activation by up-regulating the
binding activity of IL-18-induced activator protein 1. _J Immunol_ 2002; 168: 1146–1153. Article CAS Google Scholar * Schaible UE, Collins HL, Priem F, Kaufmann SH . Correction of the
iron overload defect in beta-2-microglobulin knockout mice by lactoferrin abolishes their increased susceptibility to tuberculosis. _J Exp Med_ 2002; 196: 1507–1513. Article CAS Google
Scholar * Wang L, Johnson EE, Shi HN, Walker WA, Wessling-Resnick M, Cherayil BJ . Attenuated inflammatory responses in hemochromatosis reveal a role for iron in the regulation of
macrophage cytokine translation. _J Immunol_ 2008; 181: 2723–2731. Article CAS Google Scholar * Gomes-Pereira S, Rodrigues PN, Appelberg R, Gomes MS . Increased susceptibility to
Mycobacterium avium in hemochromatosis protein HFE-deficient mice. _Infect Immun_ 2008; 76: 4713–4719. Article CAS Google Scholar * Arezes J, Jung G, Gabayan V et al. Hepcidin-induced
hypoferremia is a critical host defense mechanism against the siderophilic bacterium Vibrio vulnificus. _Cell Host Microbe_ 2015; 17: 47–57. Article CAS Google Scholar * Kasahara T, Hooks
JJ, Dougherty SF, Oppenheim JJ . Interleukin 2-mediated immune interferon (IFN-gamma) production by human T cells and T cell subsets. _J Immunol_ 1983; 130: 1784–1789. CAS PubMed Google
Scholar * Scharton TM, Scott P . Natural killer cells are a source of interferon gamma that drives differentiation of CD4+ T cell subsets and induces early resistance to Leishmania major in
mice. _J Exp Med_ 1993; 178: 567–577. Article CAS Google Scholar * Salins S, Newton C, Widen R, Klein TW, Friedman H . Differential induction of gamma interferon in Legionella
pneumophila-infected macrophages from BALB/c and A/J mice. _Infect Immun_ 2001; 69: 3605–3610. Article CAS Google Scholar * Puddu P, Fantuzzi L, Borghi P et al. IL-12 induces IFN-gamma
expression and secretion in mouse peritoneal macrophages. _J Immunol_ 1997; 159: 3490–3497. CAS PubMed Google Scholar * Kirby AC, Yrlid U, Wick MJ . The innate immune response differs in
primary and secondary Salmonella infection. _J Immunol_ 2002; 169: 4450–4459. Article CAS Google Scholar * Yin J, Ferguson TA . Identification of an IFN-gamma-producing neutrophil early
in the response to Listeria monocytogenes. _J Immunol_ 2009; 182: 7069–7073. Article CAS Google Scholar * Schleicher U, Hesse A, Bogdan C . Minute numbers of contaminant CD8+ T cells or
CD11b+CD11c+ NK cells are the source of IFN-gamma in IL-12/IL-18-stimulated mouse macrophage populations. _Blood_ 2005; 105: 1319–1328. Article CAS Google Scholar * Wu Q, Wang H, An P et
al. HJV and HFE Play Distinct Roles in Regulating Hepcidin. _Antioxid Redox Signal_ 2015; 22: 1325–1336. Article CAS Google Scholar * Chen W, Huang FW, de Renshaw TB, Andrews NC .
Skeletal muscle hemojuvelin is dispensable for systemic iron homeostasis. _Blood_ 2011; 117: 6319–6325. Article CAS Google Scholar * Clausen BE, Burkhardt C, Reith W, Renkawitz R, Forster
I . Conditional gene targeting in macrophages and granulocytes using LysMcre mice. _Transgenic Res_ 1999; 8: 265–277. Article CAS Google Scholar * Kong L, Sun L, Zhang H et al. An
essential role for RIG-I in toll-like receptor-stimulated phagocytosis. _Cell Host Microbe_ 2009; 6: 150–161. Article CAS Google Scholar * Soehnlein O, Kai-Larsen Y, Frithiof R et al.
Neutrophil primary granule proteins HBP and HNP1-3 boost bacterial phagocytosis by human and murine macrophages. _J Clin Invest_ 2008; 118: 3491–3502. Article CAS Google Scholar *
Gilberthorpe NJ, Lee ME, Stevanin TM, Read RC, Poole RK . NsrR: a key regulator circumventing Salmonella enterica serovar Typhimurium oxidative and nitrosative stress in vitro and in
IFN-gamma-stimulated J774.2 macrophages. _Microbiology_ 2007; 153: 1756–1771. Article CAS Google Scholar * Leopold Wager CM, Hole CR, Wozniak KL, Olszewski MA, Wormley FL Jr . STAT1
signaling is essential for protection against Cryptococcus neoformans infection in mice. _J Immunol_ 2014; 193: 4060–4071. Article CAS Google Scholar * Soumyarani VS, Jayakumari N .
Oxidatively modified high density lipoprotein promotes inflammatory response in human monocytes-macrophages by enhanced production of ROS, TNF-alpha, MMP-9, and MMP-2. _Mol Cell Biochem_
2012; 366: 277–285. Article CAS Google Scholar Download references ACKNOWLEDGEMENTS We are grateful to Dr Nancy Andrews for generously providing the _Hfe__−/−__, Hjv__−/−__,
Hjv__flox/flox_, and _Fpn1__flox/flox_ mice. We also thank Dr Baoxue Ge at Tongji University for providing the _E. coli_ TOP10 strain carrying the _GFP_ gene on the Puc-19 plasmid. This work
was supported by grants from the National Natural Science Foundation of China (31530034, 31330036, and 31225013 to FW; 31570791 and 91542205 to JM), and Zhejiang Provincial Natural Science
Foundation of China (LZ15H160002 to JM). We thank members of the Wang and Min laboratories for discussion. AUTHOR INFORMATION Author notes * Qian Wu and Yuanyuan Shen: These authors
contributed equally to this work. * Fudi Wang Tel: +86 571 88206385; Fax: +86 571 88206385 E-mail: [email protected] * Fudi [email protected] AUTHORS AND AFFILIATIONS * The First
Affiliated Hospital, Institute of Translational Medicine, School of Public Health, Collaborative Innovation Center for Diagnosis and Treatment of Infectious Diseases, School of Medicine,
Zhejiang University, Hangzhou, China Qian Wu, Yuanyuan Shen, Yunlong Tao, Jiayu Wei, Hao Wang, Peng An, Zhuzhen Zhang, Hong Gao, Tianhua Zhou, Fudi Wang & Junxia Min * Department of
Nutrition, Precision Nutrition Innovation Center, School of Public Health, Zhengzhou University, Zhengzhou, China Qian Wu, Hao Wang & Fudi Wang Authors * Qian Wu View author publications
You can also search for this author inPubMed Google Scholar * Yuanyuan Shen View author publications You can also search for this author inPubMed Google Scholar * Yunlong Tao View author
publications You can also search for this author inPubMed Google Scholar * Jiayu Wei View author publications You can also search for this author inPubMed Google Scholar * Hao Wang View
author publications You can also search for this author inPubMed Google Scholar * Peng An View author publications You can also search for this author inPubMed Google Scholar * Zhuzhen Zhang
View author publications You can also search for this author inPubMed Google Scholar * Hong Gao View author publications You can also search for this author inPubMed Google Scholar *
Tianhua Zhou View author publications You can also search for this author inPubMed Google Scholar * Fudi Wang View author publications You can also search for this author inPubMed Google
Scholar * Junxia Min View author publications You can also search for this author inPubMed Google Scholar CONTRIBUTIONS QW, YS, FW and JM designed the experiments. QW, YS, YT, PA, HW, ZZ, JW
and HG performed the experiments. QW, YS, YT, FW and JM analyzed the data. QW, YS, TZ, FW and JM drafted and revised the manuscript. CORRESPONDING AUTHORS Correspondence to Fudi Wang or
Junxia Min. ETHICS DECLARATIONS COMPETING INTERESTS The authors declare no conflict of interest. ADDITIONAL INFORMATION ( SUPPLEMENTARY INFORMATION is linked to the online version of the
paper on the _Cell Discovery_ website.) SUPPLEMENTARY INFORMATION SUPPLEMENTARY INFORMATION (PDF 3830 KB) RIGHTS AND PERMISSIONS This work is licensed under a Creative Commons Attribution
4.0 International License. The images or other third party material in this article are included in the article’s Creative Commons license, unless indicated otherwise in the credit line; if
the material is not included under the Creative Commons license, users will need to obtain permission from the license holder to reproduce the material. To view a copy of this license, visit
http://creativecommons.org/licenses/by/4.0/ Reprints and permissions ABOUT THIS ARTICLE CITE THIS ARTICLE Wu, Q., Shen, Y., Tao, Y. _et al._ Hemojuvelin regulates the innate immune response
to peritoneal bacterial infection in mice. _Cell Discov_ 3, 17028 (2017). https://doi.org/10.1038/celldisc.2017.28 Download citation * Received: 10 May 2017 * Accepted: 13 July 2017 *
Published: 15 August 2017 * DOI: https://doi.org/10.1038/celldisc.2017.28 SHARE THIS ARTICLE Anyone you share the following link with will be able to read this content: Get shareable link
Sorry, a shareable link is not currently available for this article. Copy to clipboard Provided by the Springer Nature SharedIt content-sharing initiative