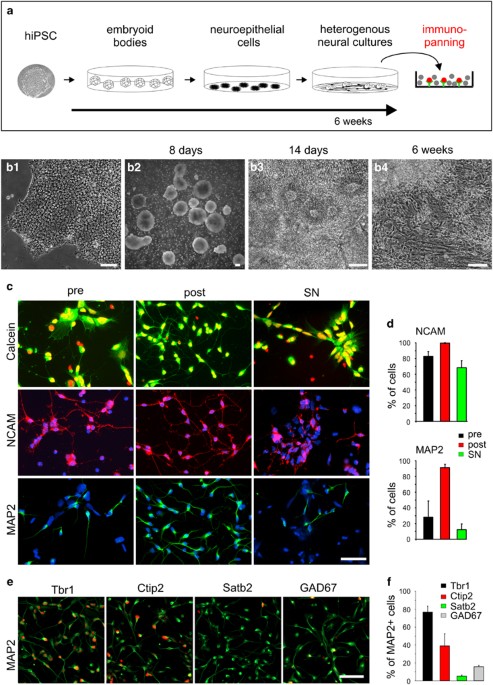
- Select a language for the TTS:
- UK English Female
- UK English Male
- US English Female
- US English Male
- Australian Female
- Australian Male
- Language selected: (auto detect) - EN
Play all audios:
ABSTRACT Human induced pluripotent stem cell (iPSC)-derived neurons have been proposed to be a highly valuable cellular model for studying the pathomechanisms of Alzheimer's disease
(AD). Studies employing patient-specific human iPSCs as models of familial and sporadic forms of AD described elevated levels of AD-related amyloid-_β_ (A_β_). However, none of the present
AD iPSC studies could recapitulate the synaptotoxic actions of A_β_, which are crucial early events in a cascade that eventually leads to vast brain degeneration. Here we established highly
reproducible, human iPSC-derived cortical cultures as a cellular model to study the synaptotoxic effects of A_β_. We developed a highly efficient immunopurification procedure yielding
immature neurons that express markers of deep layer cortical pyramidal neurons and GABAergic interneurons. Upon long-term cultivation, purified cells differentiated into mature neurons
exhibiting the generation of action potentials and excitatory glutamatergic and inhibitory GABAergic synapses. Most interestingly, these iPSC-derived human neurons were strongly susceptible
to the synaptotoxic actions of A_β_. Application of A_β_ for 8 days led to a reduction in the overall FM4–64 and vGlut1 staining of vesicles in neurites, indicating a loss of vesicle
clusters. A selective analysis of presynaptic vesicle clusters on dendrites did not reveal a significant change, thus suggesting that A_β_ impaired axonal vesicle clusters. In addition,
electrophysiological patch-clamp recordings of AMPA receptor-mediated miniature EPSCs revealed an A_β_-induced reduction in amplitudes, indicating an impairment of postsynaptic AMPA
receptors. A loss of postsynaptic AMPA receptor clusters was confirmed by immunocytochemical stainings for GluA1. Incubation with A_β_ for 8 days did not result in a significant loss of
neurites or cell death. In summary, we describe a highly reproducible cellular AD model based on human iPSC-derived cortical neurons that enables the mechanistic analysis of A_β_-induced
synaptic pathomechanisms and the development of novel therapeutic approaches. SIMILAR CONTENT BEING VIEWED BY OTHERS PROTEIN AGGREGATION AND CALCIUM DYSREGULATION ARE HALLMARKS OF FAMILIAL
PARKINSON’S DISEASE IN MIDBRAIN DOPAMINERGIC NEURONS Article Open access 24 November 2022 HIGH-CONTENT IMAGE-BASED ANALYSIS AND PROTEOMIC PROFILING IDENTIFIES TAU PHOSPHORYLATION INHIBITORS
IN A HUMAN IPSC-DERIVED GLUTAMATERGIC NEURONAL MODEL OF TAUOPATHY Article Open access 23 August 2021 TAU DEPLETION IN HUMAN NEURONS MITIGATES AΒ-DRIVEN TOXICITY Article Open access 15
February 2024 MAIN In Alzheimer's disease (AD), synapse damage and synapse loss are thought to underlie cognitive deficits.1 Oligomers of the amyloid-_β_ (A_β_) peptide appear to induce
synaptic failure as an early event in the etiology of AD.2, 3, 4 However, despite its well-established synapse-impairing effects in rodent models,5, 6, 7 the synaptotoxic actions of A_β_
most relevant for the human disease have not been identified in a human model system. Several studies have investigated the synaptotoxic effects of A_β_ in cultured rodent neurons and in
transgenic mouse models revealing a multitude of potential mechanisms affecting synapses. Postsynaptic A_β_ actions result in the loss of functional
(_α_-amino-3-hydroxy-5-methyl-4-isoxazolepropionic acid (AMPA)-type) glutamate receptors,8, 9, 10 involve long-term depression-like mechanisms,9, 11, 12 and lead to the degradation of the
entire postsynapse (dendritic spines).9, 11, 13 In addition, several distinct presynaptic A_β_ actions on the synaptic vesicle cycle have been described.10, 14 Furthermore, A_β_-induced
impairments of axonal transport regulation and A_β_-induced axon degeneration have been found in rodent neurons.15, 16, 17 This puzzling diversity of A_β_-induced synapse-related defects
raises the question whether all of them are involved in the early pathomechanisms of human AD. In addition to well-established animal systems, the modelling of human neurological disease
pathologies by human induced pluripotent stem cell (hiPSC) technology18 has been proposed as an innovative approach.19, 20, 21 The _in vitro_ differentiation of hiPSCs to excitable neurons
has been reported using a variety of protocols.22, 23, 24 However, quantitative analysis of both functional glutamatergic and GABAergic synapses has been difficult to achieve.19, 25, 26 In
addition to studying the functional properties of iPSC-derived human neurons from healthy individuals, the _in vitro_ differentiation of patient-derived iPSCs has been used to model complex
neurodevelopmental and neurodegenerative diseases.19, 27, 28 Recently, iPSCs derived from AD patients have been reported to exhibit increased secretion of A_β_ upon _in vitro_ neuronal
differentiation; however, neither a loss of synapses nor an impairment of synapse function was detected.21, 29, 30, 31, 32, 33 Here we describe a hiPSC-based, carefully optimized _in vitro_
differentiation protocol, including a novel immunopanning step, which enabled us to study the deleterious effects of application of A_β_ on human cortical neurons and on human synapses.
RESULTS NEURAL DIFFERENTIATION OF HIPSCS AND IMMUNOPURIFICATION OF HIPSC-DERIVED IMMATURE NEURONS hiPSCs were cultured (Supplementary Figure S1) and _in vitro_ differentiated using an
embryoid body (EB) system similar to published protocols.22 After initial differentiation, EBs were plated on a matrigel substrate leading to the formation of paired box protein 6
(Pax6)-expressing neuroepithelial rosettes (Supplementary Figure S2) that further differentiated to heterogeneous cultures also containing non-neuronal cells (Figures 1a and b). After 6–8
weeks of _in vitro_ differentiation, heterogeneous cultures were dissociated to single cells, which were subjected to immunopurification. Classical immunopanning34 with specific
modifications was performed using the neural cell adhesion molecule (NCAM) antibody VIN-IS-53 to isolate immature neurons expressing NCAM at a high level. To quantify immunopanning
efficiency, dissociated cells without immunopanning (control), dissociated cells isolated by NCAM immunopanning, and dissociated cells non-adherent to the panning plates, respectively
(Figure 1c), were immunocytochemically stained for NCAM and the neuronal marker microtubule-associated protein 2 (MAP2) 1 day after immunopurification (Figures 1d and e). The fraction of
MAP2-positive cells was strongly increased in cells isolated by NCAM immunopanning (91.2±4.3%) as compared with control cells (28.1±20.6%) and to cells non-adherent to the panning plates
(12.2±7.4%) (Figure 1g). The fraction of NCAM-positive cells was also increased by immunopanning (Figure 1f); however, as expected from the low level NCAM expression in neural precursor
cells, the increase was less pronounced as compared with MAP2. We next characterized the immunopurified immature neurons using immunocytochemistry. Staining for cortical marker proteins
revealed that the vast majority of MAP2-positive cells expressed markers of deep layer cortical neurons (Ctip2 (chicken ovalbumin upstream promoter transcription factor-interacting protein
2), Tbr1 (T-box, brain, 1)), while only 5.0±1.4% of the MAP2-positive neurons expressed the upper layer marker special AT-rich sequence-binding protein 2 (Satb2; Figures 1h and i). Similar
to the composition of neuronal cell types in the _in vivo_ cortex, 15.7±1.7% of the MAP2-positive neurons were GABAergic (glutamic acid decarboxylase 67 (GAD67) positive) (Figures 1h and i).
Survival of immature neurons was not affected by the immunopanning procedure (Figure 1c). In summary, NCAM immunopanning of hiPSC-derived heterogeneous cultures resulted in highly purified
MAP2-positive immature deep-layer cortical neurons. MORPHOLOGICAL MATURATION AND ACTION POTENTIAL GENERATION IN HIPSC-DERIVED NEURONS PURIFIED BY IMMUNOPANNING Further cultivation of the
MAP2-positive immature human neurons led to the formation of extended neurites after 1 week and to the formation of a dense neuritic network of mature neurons exhibiting enlarged somata at 8
weeks after immunopurification (Figure 2a). These cultures contained only very few glial cells due to the inhibition of proliferation of non-neuronal cells (see Materials and Methods). To
study whether morphologically maturated iPSC-derived human neurons exhibit essential functional properties typical of cultured cortical neurons, we performed a basic electrophysiological
characterization. Whole-cell patch-clamp recordings (at 8 weeks after immunopurification) of the membrane potential (57.8±0.7 mV resting potential) in current-clamp mode revealed the
generation of action potentials upon injection of depolarizing current in all neurons tested (Figure 2b). To demonstrate the expression of voltage-dependent Na+ currents, we did whole-cell
recordings in voltage-clamp mode at a holding potential of −60 mV. Step depolarizations of the membrane potential elicited typical inward Na+ currents that were blocked by addition of
tetrodotoxin (TTX; 1 _μ_M; Figure 2c). Thus, electrophysiological analysis revealed essential functional properties such as electrical excitability and TTX-sensitive voltage-dependent Na+
currents in hiPSC-derived neurons at 8 weeks after immunopurification. CHARACTERIZATION OF FUNCTIONAL SYNAPSES IN MATURE HIPSC-DERIVED NEURONS PURIFIED BY IMMUNOPANNING At this stage of
maturation, the formation of synaptic structures was indicated by vesicle clusters immunocytochemically stained for the synaptic vesicle-associated proteins VAMP2 (vesicle-associated
membrane protein 2)/synaptobrevin and synapsin I on dendrites (Figures 3a and b). The presence of both glutamate and γ-aminobutyric acid (GABA) containing presynaptic vesicle clusters was
confirmed by a punctate immunostaining on dendrites for the vesicular glutamate transporter 1 (vGlut1, colocalized with postsynaptic postsynaptic density protein 95 (PSD95) puncta) and for
the vesicular GABA transporter (vGAT), respectively (Figures 3c and d). Moreover, the formation of functional synapses was indicated by spontaneous miniature postsynaptic currents (mPSCs)
that were observed by whole-cell patch-clamp recording at −60 mV holding potential (Figures 3e and f). We further characterized spontaneous mPSCs pharmacologically by using specific
antagonists. AMPA receptor-mediated miniature excitatory postsynaptic currents (mEPSCs) were isolated by addition of gabazine (10 _μ_M) and TTX (1 _μ_M), and were blocked by the AMPA
receptor antagonist 6,7-dinitroquinoxaline-2,3-dione (DNQX; 10 _μ_M; Figure 3e). GABAA receptor-mediated mPSCs were isolated by addition of DNQX and TTX and were blocked by addition of
gabazine (Figure 3f). This demonstrates the presence of both functional glutamatergic and GABAergic synapses. Taken together, our findings demonstrate that at 8 weeks after
immunopurification our iPSC-derived human neurons exhibit essential functional properties, such as excitability, synaptic activity, and functional glutamatergic and GABAergic synapses. A_Β_
INDUCED LOSS OF CYCLING VESICLE CLUSTERS IN IPSC-DERIVED HUMAN NEURONS To investigate whether functional synapses in iPSC-derived human neurons are susceptible to the deleterious effects of
A_β_, we added A_β_ contained in the supernatant from cultures of 7PA2 Chinese hamster ovary (CHO) cells (expressing human APP751 carrying the familial amyloid precursor protein (APP) V717F
mutation)5, 35, 36 to hiPSC-derived neurons at 8 weeks after immunopurification. Conditioned medium from 7PA2 cells was diluted 1:1 with standard culture medium.37 To test for potential
unspecific effects of the 7PA2 supernatant, we immunodepleted A_β_ from the 7PA2 conditioned medium by using the anti-A_β_ monoclonal antibody IC16.37, 38 A_β_-induced defects were analysed
8 days after A_β_ application. First, we studied the effects of A_β_ on cycling synaptic vesicle clusters in the processes of iPSC-derived human neurons. Cycling vesicle clusters were
stained by the fluorescent dye FM4-64 (_N_-(3-triethylammoniumpropyl)-4-(6-(4-(diethylamino) phenyl) hexatrienyl) pyridinium dibromide).39 Uptake of extracellularly added FM4–64 (10 _μ_M) in
recycling vesicles was stimulated by triggering the exocytosis and endocytosis of synaptic vesicles with extracellular electrical stimulation. After removal of extracellular FM4–64 dye,
FM4–64-stained vesicle clusters were imaged by fluorescence microscopy (Figure 4a). For each FM4–64 staining experiment, the number of fluorescent puncta (i.e., vesicle clusters) was
determined after thresholding the FM4–64 fluorescence image (see Figure 4a) and was multiplied with the mean total fluorescence intensity per punctum, resulting in an overall FM4–64
fluorescence signal per area of neuropil. Strikingly, 8 days after addition of A_β_, we observed a strong, significant reduction in the overall FM4–64 fluorescence signal (Figures 4a and b)
indicating a toxic effect of A_β_ on recycling vesicle clusters. Addition of immunodepleted 7PA2 supernatant did not affect the overall FM4–64 fluorescence signal demonstrating a specific
effect of A_β_ contained in the 7PA2-conditioned medium. The A_β_-induced reduction of the overall FM4–64 fluorescence signal was largely due to a reduction in the number of FM4–64-stained
puncta (vesicle clusters) per area of neuropil as indicated by plotting FM4–64 puncta density _versus_ the mean total fluorescence intensity per punctum (Figure 4b). We also studied the
destaining of FM4–64-stained vesicle clusters by triggering vesicle re-exocytosis with extracellular electrical stimulation (1200 stimuli at 20 Hz (for 60 s)); Figure 4c). The mean
destaining kinetics of FM4–64 puncta were not significantly affected by addition of A_β_, thus further supporting that a reduction in the number of vesicle clusters was the major
A_β_-induced alteration. To further confirm an A_β_-induced impairment of vesicle clusters, we immunocytochemically stained iPSC-derived human neurons for the synaptic vesicle marker vGlut1
after incubation with A_β_. Again, we observed a significant reduction in the density of vGlut1 immunopositive puncta (vesicle clusters) in neurites of A_β_-treated neurons as compared with
vehicle-treated control cultures (Figures 4d and e). Addition of immunodepleted 7PA2 supernatant did not affect the number of vGlut1 puncta, again demonstrating a specific effect of A_β_
contained in the 7PA2-conditioned medium. To check for A_β_-induced changes in the density of processes and in cell density, we fluorescently stained iPSC-derived human neurons by addition
of the membrane permeable dye calcein-AM directly following the FM4–64 staining/destaining experiments (Figure 4f). Eight days after addition of A_β_, the number of neuronal cell bodies and
the density of processes (determined by counting processes crossing a randomly chosen line) were not significantly affected (Figures 4g and h). In summary, our results indicate that addition
of A_β_ results in a strong reduction in the density of vesicle clusters in the neurites of iPSC-derived human neurons. A_Β_ INDUCED FUNCTIONAL IMPAIRMENT OF GLUTAMATERGIC SYNAPSES IN
IPSC-DERIVED HUMAN NEURONS We next studied whether the addition of A_β_ induced deleterious changes at _bona fide_ synaptic sites contacting postsynaptic dendrites. To focus on presynaptic
vesicle clusters, we reanalysed the FM4–64 puncta (from the FM4–64 staining/destaining experiments described above) that contacted the proximal dendrites of iPSC-derived human neurons.
Proximal dendrites arising from neuron somata were identified by calcein-AM staining of whole neurons after FM4–64 staining/destaining experiments, and FM4–64 puncta were analysed after
thresholding the FM4–64 fluorescence image (Figure 5a). Eight days after addition of A_β_, we did not observe a significant change in the dendritic density of FM4–64 puncta or a significant
change in total FM4–64 fluorescence intensity per punctum or a significant change in the overall presynaptic FM4–64 fluorescence signal per dendrite (Figure 5b). Furthermore, the
quantitative analysis of the stimulation-induced destaining of FM4–64 puncta did not reveal any significant changes in exocytosis (Figure 5c). We also reanalysed the presynaptic vGlut1
immunopositive puncta (described above) located on dendrites, which were identified by MAP2 co-immunostaining of dendrites (Figure 5d). Again, 8 days after addition of A_β_, we observed no
significant changes in the density of vGlut1 puncta on dendrites (Figure 5e). Taken together, the analysis of FM4–64 fluorescent and of vGlut1 immunopositive puncta on dendrites thus
revealed no significant presynaptic alterations at a relatively early phase of incubation with A_β_, suggesting that _bona fide_ presynaptic vesicle clusters and functional release
properties were not affected by short-term application of A_β_. We next addressed whether the deleterious effects of A_β_ on functional properties of glutamatergic synapses, which are well
described in rodent neurons, are also observed in iPSC-derived human neurons. We recorded AMPA receptor-mediated mEPSCs (AMPA mEPSCs) using the whole-cell patch-clamp technique (1 _μ_M TTX,
10 _μ_M gabazine added, holding potential: −60 mV). We again applied A_β_ containing supernatant of the 7PA2 CHO cell line at 8 weeks after immunopurification and studied its effects on AMPA
mEPSCs 8 days later. Addition of A_β_ resulted in a strong, significant reduction of the mean amplitude of AMPA mEPSCs (Figures 6a and b), indicating a loss of postsynaptic AMPA receptor
function. This amplitude reduction was not observed upon addition of 7PA2 supernatant immunodepleted of A_β_, demonstrating a specific A_β_ action. Addition of A_β_ did not lead to a
significant reduction of the mean frequency of AMPA mEPSCs (Figures 6a and b). The observed trend to a reduced AMPA mEPSC frequency is likely to be caused by limitations in the detection of
small amplitude minis. To further confirm an A_β_ induced impairment of postsynaptic AMPA receptors, we performed immunocytochemical stainings for GluA1(GluR1) (AMPA receptor subunit 1),
PSD95, and MAP2 (to visualize dendrites) in iPSC-derived human neurons. Eight days addition of A_β_ resulted in a significant reduction of the density of GluA1 immunopositive puncta on
MAP2-immunostained dendrites (Figures 6c and d). Furthermore, the density of synaptic GluA1 immunopositive puncta (colocalized with PSD95 puncta) was also significantly reduced 8 days after
A_β_ application (Figure 6d). The dendritic density of PSD95 immunopositive puncta was not significantly altered (Figures 6c and d). These A_β_-induced changes were not detectable upon
addition of 7PA2 supernatant immunodepleted of A_β_, again demonstrating a specific A_β_ action. Taken together, the observed A_β_-induced reduction of AMPA mEPSC amplitudes and of dendritic
GluA1 expression indicates an early action of A_β_ on postsynaptic AMPA receptors in iPSC-derived human neurons. Interestingly, as indicated by the lack of A_β_ effects on vesicle clusters
on dendrites, an early A_β_-induced presynaptic impairment was not detectable. A_Β_-INDUCED EFFECTS ON CELL SURVIVAL, CELLULAR STRESS AND TAU PROTEIN IN HIPSC-DERIVED NEURONS To study
whether our short-term application of A_β_ compromises cell survival, we stained healthy neurons and nuclei of disrupted cells, respectively by performing a live/dead assay (see Materials
and Methods). Healthy neurons were fluorescently stained by addition of membrane permeable calcein-AM, which is intracellularly converted to fluorescent calcein. Nuclei without an intact
plasma membrane were stained by Ethidium Homodimer 1 (Figure 7a). At 8 days of incubation, we did not observe significant effects of the addition of A_β_ on cell survival (Figure 7b).
Because A_β_-induced cellular stress might be detectable prior to cell death, we studied the expression of marker proteins for endoplasmic reticulum (ER) stress and autophagy by western
blotting analysis. Interestingly, we found a slight A_β_-induced (8 days incubation) increase in the autophagy marker microtubule-associated protein 1A/1B-light chain II (LC3-II) and in the
ER stress markers binding immunoglobulin protein (BiP) and CCAAT-enhancer-binding protein homologous protein (CHOP) (Figures 7c and d). This is in line with the recent description of
increased ER stress in AD patient-derived iPSC lines upon neuronal differentiation.40 We further analysed A_β_-induced changes in tau protein expression and phosphorylation state by western
blotting. Although the expression level of tau protein appeared not to be affected by short-term application of A_β_ (8 days incubation), an increased phosphorylation of tau was clearly
detectable (Figures 7e and f). This finding in human neurons is in line with the A_β_-induced phosphorylation of tau protein, which has been described in mouse models previously.4, 41 In
summary, our results indicate that at an early phase of A_β_ actions defects in synapse function are clearly revealed in iPSC-derived human neurons by changes in postsynaptic AMPA receptors.
In addition, A_β_-induced alterations in neuritic vesicle clusters, in ER stress/autophagy marker proteins, and in tau protein phosphorylation were found. Our work thus establishes that
iPSC-derived human neurons represent an innovative model system to study the molecular mechanisms of A_β_-induced synapse damage in AD. DISCUSSION In this study, we used hiPSCs to establish
an innovative cell culture model of cortical neurons for specific aspects of AD. We analysed the effects of A_β_ on human neurons, human synapses, and synaptic vesicle clusters. We observed
a loss of axonal vesicle clusters, a loss of postsynaptic AMPA receptors, and increased tau protein phosphorylation, thus revealing complex deleterious actions of A_β_. _IN VITRO_
DIFFERENTIATION OF HUMAN IPSCS AND IMMUNOPURIFICATION OF IPSC-DERIVED IMMATURE HUMAN NEURONS _In vitro_ differentiation of human pluripotent stem cells to neurons has been established by
several groups.22, 23, 42 Without addition of specific morphogens/growth factors,43 iPSCs differentiate to dorsal, cortical-like neuronal progenitor cells characterized by Pax6
expression;20, 22, 44 however, other contaminating cell types are also generated. The invention of immunopanning protocols34 enables the highly efficient purification of specific types of
neuronal precursors, neurons, or astrocytes.45, 46, 47 Similarly, the presence of NCAM has been used for selecting neuronally differentiated cells.48, 49 Here we have developed an
immunopanning procedure based on NCAM antibodies, which yielded >90% MAP2-positive immature neurons. Immunocytochemical characterization of the purified neurons revealed typical
cortical-like cultures consisting of mainly glutamaterigc and about 20% GABAergic neurons. FUNCTIONAL MATURATION OF HUMAN IPSC-DERIVED IMMATURE NEURONS, INCLUDING SYNAPSE FORMATION In line
with our results, it has been shown that neurons derived from human iPSCs generate action potentials and exhibit voltage-activated Na+ and K+ currents.19, 24, 44, 50 In addition, our
iPSC-derived human neurons fired repetitive action potentials reminiscent of the firing pattern of regular spiking cortical pyramidal neurons.24 Similar to primary cultured rodent neurons,51
the formation of functional synapses has also been observed in human iPSC-derived neurons; however, reliable synapse formation and function appears to be difficult to achieve.19, 25, 26
Importantly, our iPSC _in vitro_ differentiation protocol resulted reliably in the formation of both functional glutamatergic and GABAergic synapses, thus leading to synaptically active
networks. SYNAPTOTOXIC EFFECTS OF A_Β_ IN HUMAN IPSC-DERIVED NEURONS We used our innovative model system for studying synaptic aspects of AD. Oligomeric A_β_ peptides are thought to be
crucial molecular entities in AD1, 2, 3, 5, 52 and exhibit—in rodent neurons—toxic effects at glutamatergic synapses leading ultimately to synapse loss.8, 11, 37, 52, 53, 54, 55, 56 The
initial deleterious actions of A_β_ on the function of glutamatergic synapses might be specifically localized either presynaptically or postsynaptically. In rodent neurons, an initial
A_β_-induced removal of postsynaptic AMPA receptors by facilitating AMPA receptor endocytosis has been described.9, 10, 12 Furthermore, A_β_ disrupts membrane trafficking and synaptic
recruitment of AMPA receptors by reducing surface expression.57 In line with an initially postsynaptic mechanism, we found a pronounced A_β_-induced reduction in both the amplitudes of
AMPAR-mediated mEPSCs and in postsynaptic AMPA receptor clusters in hiPSC-derived neurons. In addition, also presynaptic mechanisms of action of A_β_ affecting both exocytosis and
endocytosis have been proposed in rodent neurons.10, 14 However, our analysis of both FM-stained and immunocytochemically stained vesicle clusters apposed to dendrites in hiPSC-derived
neurons did not reveal any short-term presynaptic effects of A_β_, thus indicating that A_β_-induced alterations in postsynaptic AMPA receptors might be of crucial importance in the human
disease. In addition to A_β_-induced synaptotoxicity, AD is characterized by the formation of neurofibrillary tangles and tau hyperphosphorylation.58 A_β_-induced tau phosphorylation4, 13,
41 has been proposed to be an early event leading to the formation of neurofibrillary tangles typical of tauopathy.41 In line with familial AD patient-derived iPSC-derived neurons,33 our
data demonstrate that A_β_-induced tau phosphorylation occurs in hiPSC-derived neurons after short-term application of A_β_. Furthermore, we observed a slight A_β_-induced increase in
proteins related to the ER stress-induced unfolded protein response (UPR). UPR includes enhanced expression of chaperones such as BiP, increased degradation of misfolded proteins, and
activates autophagy.59 Failure of this system to restore ER homeostasis initiates apoptotic mechanisms. In line with our results, A_β_ oligomers have been described to induce the UPR.60
EFFECTS OF A_Β_ ON VESICLE CLUSTERS IN HIPSC-DERIVED NEURONS Intriguingly, upon A_β_ application we observed a reduced density of non-synaptic axonal vesicle clusters, which are well known
to undergo exocytosis and endocytosis.61, 62 Because we did not observe any A_β_-induced changes in the FM destaining kinetics (reflecting exocytosis) and in the FM fluorescence intensity
(reflecting endocytosis), an A_β_-induced impairment of the axonal transport of vesicles without axon degeneration appeared to underlie the reduction in the density of axonal vesicle
clusters. In line with this idea, in rodent neurons an impairment of the axonal transport by A_β_ has been observed.15, 16, 17 A_β_-induced defects in the transport of organelles, for
example, mitochondria,63 and in the transport of dense core and synaptic vesicles63, 64 without axon degeneration have been described previously. Thus our results in iPSC-derived human
neurons strongly support an important role of an A_β_-induced impairment in axonal transport of synaptic vesicles at early stages of the human disease. CONCLUSION: HUMAN IPSC DERIVED NEURONS
AS AN INNOVATIVE MODEL SYSTEM TO STUDY TOXIC EFFECTS OF A_Β_ Here we have established hiPSC-derived neurons as an innovative AD model system to study the effects of A_β_. Toxic effects of
A_β_ were complex, including both non-synaptic effects on axonal vesicles and _bona fide_ postsynaptic impairment of AMPA receptors. In addition, an A_β_ induced increase in tau protein
phosphorylation was found. Most importantly, our iPSC-derived human model system will aid the development of novel AD therapeutics based on the investigation of the molecular mechanisms of
deleterious A_β_ actions in human neurons. MATERIALS AND METHODS HIPSC CULTURE AND NEURAL DIFFERENTIATION Experiments were approved by the local ethics committee of the University of
Düsseldorf. Human iPSC line 8/25 (ref. 65) and in some experiments human iPSC line DF6–9–9T.B (WiCell, provided by Dr. J Thomson, University of Wisconsin66) were cultured according to
standard procedures in mTeSr medium (STEMCELL Technologies, Köln, Germany) or in E8 medium67 on hESC qualified matrigel (Corning, Corning, NY, USA) coated cell culture six-well plates
(Sarstedt, Nürnbrecht, Germany). Neural differentiation was performed according to a modified protocol of Li _et al._22 EBs were allowed to form in T25 cell culture flasks (Sarstedt) in
N2B27 medium containing 50% DMEM/F12 (Biochrom, Berlin, Germany), 50% Neurobasal, 2 mM Glutamax, 100 units/ml penicillin and 100 _μ_g/ml streptomycin, 0.1 mM _β_-mercaptoethanol, 1% B27
without vitamin A (all Gibco, Darmstadt, Germany), 3 _μ_g/ml heparin (Sigma Aldrich, St. Louis, MO, USA), and a modified N2 supplement with final concentrations of 7.5 _μ_g/ml human insulin,
50 _μ_g/ml BSA, 8 _μ_g/ml putrescine, 3.1 ng/ml progesterone, 2.6 ng/ml sodium selenite (all Sigma Aldrich), and 25 _μ_g/ml human holo-transferrin (Calbiochem, Darmstadt, Germany). After 14
days of suspension culture, EBs were plated on 100-mm cell culture dishes coated with growth factor reduced matrigel (Corning) in N2B27 medium. Six-to-8 weeks after starting
differentiation, heterogeneous cultures were harvested for immunopanning by scraping in DPBS without calcium and magnesium (PanBiotech, Aidenbach, Germany). IMMUNOPANNING OF HIPSC-DERIVED
IMMATURE NEURONS AND NEURONAL MATURATION Panning plates (100 mm, bacterial petri dish quality; Falcon/Corning, Darmstadt, Germany) were prepared by incubating goat anti mouse IgG+IgM
antibody (Jackson Immunoresearch, Suffolk, UK) at 10 _μ_g/ml in 9 ml of 50 mM Tris HCl/pH 9.5 overnight at 4 °C. After three washing steps with DPBS, the panning plates were incubated
overnight at 4 °C with the primary mouse anti-NCAM antibody (VIN-IS-53, kindly provided by Dr. Peter Andrews, University of Sheffield, UK) (0.55 _μ_g/cm2 in 0.2% BSA Fraction V (Gibco)/DPBS,
7 ml per plate). Plates were washed twice with DPBS and once with 0.02% BSA. Cells harvested from one 100-mm differentiation plate were enzymatically digested in accutase (Sigma Aldrich)
for 30 min. After enzyme removal, cells were dissociated in 0.2% BSA in DPBS supplemented with 1 mg/ml glucose (Sigma Aldrich) and 0.33 mM sodium pyruvate (Gibco), pelleted at 200 g,
resuspended in 0.02% BSA plus glucose and sodium pyruvate, and distributed to four panning plates (7 ml per plate). Cells were incubated on the panning plates for 10 min at RT. Non-adherent
cells were removed by washing 10 times with DPBS. Adherent cells were detached by repeated pipetting (P1000, Eppendorf, Wesseling-Berzdorf, Germany) and rinsing of the plates with 0.02% BSA.
Cells were pelleted at 130 × _g_ for 10 min. Twenty-five thousand cells were plated on PO (1 mg/ml in borate buffer, coating overnight at RT) and laminin (10 mg/ml, coating 3 h at 37 °C)
coated coverslips (12 mm, Assistant, Sondheim/Rhön, Germany) in a 10-_μ_l drop of neuronal culture medium, that is, Neurobasal, 2 mM Glutamax, 1 mM Sodium pyruvate, 100 units/ml penicillin,
and 100 _μ_g/ml streptomycin (all Gibco), 2% NS21 supplement,68 25 _μ_g/ml human insulin, 100 _μ_g/ml BSA, 16 _μ_g/ml putrescine, 62 ng/ml progesterone, 40 ng/ml sodium selenite (all Sigma
Aldrich), 50 _μ_g/ml human holo-transferrin (Calbiochem), and 30 ng/ml BDNF (Peprotech, Hamburg, Germany). Two millilitres of medium was added after neurons had attached (30 min at 37 °C).
To inhibit proliferation of neural stem cells, cultures were treated with 10 _μ_M cytosine _β_-D-arabinofuranoside hydrochloride (Sigma Aldrich) for the first 5 days. After removal of the
mitotic inhibitor, half of the medium was changed once per week over a period of 2–3 months. Evaporation was compensated for by the addition of aqua bidest (Sigma Aldrich). A_Β_ CONTAINING
7PA2-CONDITIONED MEDIUM AND A_Β_ IMMUNODEPLETION All procedures were carried out as described.37 In brief, conditioned neuronal culture medium containing cell-secreted A_β_ was obtained from
a CHO cell line (7PA2) that expresses mutant human APP751 (V717F).35, 36 As determined by ELISA (A_β_40), 7PA2-conditioned medium contained A_β_ at a concentration of 25–50 ng/ml. A_β_
containing 7PA2-conditioned medium was immunodepleted with anti-A_β_ antibody (IC16)38 coupled to NHS-Sepharose (GE Healthcare, Chalfont St Giles, UK) by overnight incubation at 4 °C
followed by centrifugation. Depletion of A_β_ was confirmed by immunoblotting. IMMUNOCYTOCHEMISTRY AND IMMUNOBLOTTING Immunocytochemistry was carried out according to standard protocols, and
quantification of images was carried out as described previously.37 Antibodies used are listed in Supplementary Table S1. DAPI (10 _μ_g/ml) was used to stain nuclei. Immunoblotting was
performed according to standard protocols as described previously.69 Briefly, cells were scraped in 2 × loading buffer (200 mM Tris/HCl pH 6.8, 5% SDS, 20% glycerol, 0.04% bromphenol blue,
200 mM DTT), boiled for 5 min and centrifuged at 16.000 × _g_ for 3 min. Lysates of different samples were adjusted for GAPDH content after analyzing triplicates by SDS-PAGE and
immunoblotting. Adjusted samples were separated by SDS-PAGE (12%, Tris-Glycine) and blotted on nitrocellulose membranes. Immunoblotting and protein detection was performed according to
standard procedures. Membranes were blocked with 10% Roti-Block (Carl Roth, Karlsruhe, Germany) in Tris-buffered saline with 0.1% Tween 20. Proteins of interest were visualized using Western
Bright Quantum ECL (Biozym, Hessisch Oldendorf, Germany) and the Chemi Doc XRS System (Biorad, Munich, Germany). CALCEIN STAINING, FLUORESCENCE IMAGING, AND ANALYSIS OF CELL DEATH For
staining of healthy cells, we incubated cells with the calcein-green component (1/2000) of the LIVE/DEAD Kit (Molecular Probes, Darmstadt, Germany) for 10 min at 37 °C followed by
immunocytochemistry (or direct imaging), which was performed according to standard procedures.37 Fluorescence imaging was carried out on a Zeiss Axiovert 200 M inverted fluorescence
microscope (Zeiss, Oberkochen, Germany) equipped with a 12-bit monochrome CoolSNAP ES2 CCD camera (Photometrics, Tucson, AZ, USA) using the MetaVue and Metamorph (analysis) software
(Visitron Systems, Puchheim, Germany). A_β_-induced cell death was studied by using the LIVE/DEAD Kit (Molecular Probes) according to the manufacturer’s instructions. ELECTROPHYSIOLOGY AND
DATA ANALYSIS Whole-cell patch-clamp recordings were carried out using an EPC7 patch-clamp amplifier (HEKA, Ludwigshafen/Rhein, Germany) and pCLAMP software (Molecular Devices, Sunnyvale,
CA, USA) as described.37, 70 Intracellular solution contained 110 mM KCl, 0.25 mM CaCl2, 10 mM EGTA, and 20 mM HEPES, pH 7.3. The extracellular solution contained 130 mM NaCl, 5 mM KCl, 2.5
mM CaCl2, 1 mM MgCl2, and 20 mM HEPES, pH 7.3. Action potentials were elicited by depolarizing current injection in current-clamp mode (membrane potential −50 to −60 mV). Voltage-dependent
currents (elicited by step depolarizations) and mPSCs were recorded in voltage-clamp mode (−60 mV holding potential). Quantitative analysis of AMPA mEPSCs was carried out using the Mini
Analysis software (Synaptosoft, Decatur, GA, USA). FLUORESCENCE IMAGING OF CYCLING VESICLE CLUSTERS Cycling vesicle clusters were fluorescently stained by FM4–64 uptake.39 FM4–64 (10 _μ_M)
was added to the extracellular solution (containing in mM: 119 NaCl, 2,5 KCl, 2 CaCl2, 2 MgCl2, 25 HEPES, 30 glucose, pH=7.4), and extracellular stimulation (400 stimuli at 20 Hz) was used
to elicit exocytic/endocytic cycling of vesicles and FM4–64 uptake. Sixty seconds after stimulation, extracellular FM4–64 was removed by washing with extracellular solution (0 mM CaCl2 with
addition of DNQX (10 _μ_M) and D-AP5 (50 _μ_M); 2 min wash, then 2 min wash in the presence of ADVASEP-7 (1 mM), and then 10 min wash), and fluorescent FM4–64 puncta were imaged. For
studying exocytosis of FM4–64 stained vesicles, destaining of FM4–64 puncta was elicited by extracellular electrical stimulation (1200 stimuli at 20 Hz (for 60 s)). After 100 s of
destaining, a second stronger stimulation (2000 stimuli at 20 Hz without imaging (for 100 s)) was carried out to reach background fluorescence levels. Quantitative image analysis was carried
out by defining regions of interest (ROI) around FM4–64 puncta. Total fluorescence intensity was determined for each FM4–64 punctum using the Metamorph software (Molecular Devices). Total
background intensity was determined after the second stimulation within the same ROI and was subtracted from the total fluorescence intensity within the ROI to obtain the total FM4–64
fluorescence signal per punctum. Decay time constants of FM4–64 destaining were determined by monoexponentially fitting (SigmaPlot11 software, Systat Software Inc, San Jose, CA, USA) the
mean fluorescence decay after averaging the normalized (intensity at the start of stimulation was set to 100%) destaining curves of FM4–64 puncta obtained from a field of view (Figure 4) or
a proximal dendrite (Figure 5). STATISTICS All data are given as means±S.E.M. Results were statistically analysed using one-way ANOVA with Holm–Sidak _posthoc_ test (SigmaPlot11 software).
ABBREVIATIONS * A_β_: amyloid-_β_ * AD: Alzheimer's disease * AMPA: _α_-amino-3-hydroxy-5-methyl-4-isoxazolepropionic acid * APP: amyloid precursor protein * BiP: binding immunoglobulin
protein * CHO: Chinese hamster ovary * CHOP: CCAAT-enhancer-binding protein homologous protein * Ctip2: chicken ovalbumin upstream promoter transcription factor-interacting protein 2 *
DNQX: 6,7-dinitroquinoxaline-2,3-dione * EB: embryoid body * EPSC: excitatory postsynaptic current * ER: endoplasmic reticulum * FM4–64: _N_-(3-triethylammoniumpropyl)-4-(6-(4-(diethylamino)
phenyl) hexatrienyl) pyridinium dibromide * GABA: γ-aminobutyric acid * GAD67: glutamic acid decarboxylase 67 * iPSC: induced pluripotent stem cell * LC3: microtubule-associated protein
1A/1B-light chain 3 * MAP2: microtubule-associated protein 2 * mPSC: miniature postsynaptic current * NCAM: neural cell adhesion molecule * Pax6: paired box protein 6 * PSD95: postsynaptic
density protein 95 * Satb2: special AT-rich sequence-binding protein 2 * Tbr1: T-box, brain, 1 * TTX: tetrodotoxin * UPR: unfolded protein response * VAMP2: vesicle-associated membrane
protein 2 * vGAT: vesicular GABA transporter * vGlut1: vesicular glutamate transporter 1 REFERENCES * Terry RD, Masliah E, Salmon DP, Butters N, DeTeresa R, Hill R _et al_. Physical basis of
cognitive alterations in Alzheimer's disease: synapse loss is the major correlate of cognitive impairment. _Ann Neurol_ 1991; 30: 572–580. Article CAS Google Scholar * Selkoe DJ .
Alzheimer's disease is a synaptic failure. _Science_ 2002; 298: 789–791. Article CAS Google Scholar * Haass C, Selkoe DJ . Soluble protein oligomers in neurodegeneration: lessons
from the Alzheimer's amyloid _β_-peptide. _Nat Rev Mol Cell Biol_ 2007; 8: 101–112. Article CAS Google Scholar * Spires-Jones TL, Hyman BT . The intersection of amyloid beta and tau
at synapses in Alzheimer's disease. _Neuron_ 2014; 82: 756–771. Article CAS Google Scholar * Walsh DM, Klyubin I, Fadeeva JV, Cullen WK, Anwyl R, Wolfe MS _et al_. Naturally secreted
oligomers of amyloid _β_ protein potently inhibit hippocampal long-term potentiation _in vivo_. _Nature_ 2002; 416: 535–539. Article CAS Google Scholar * Oddo S, Caccamo A, Shepherd JD,
Murphy MP, Golde TE, Kayed R _et al_. Triple-transgenic model of Alzheimer's disease with plaques and tangles: intracellular Abeta and synaptic dysfunction. _Neuron_ 2003; 39: 409–421.
Article CAS Google Scholar * Walsh DM, Selkoe DJ . A beta oligomers—a decade of discovery. _J Neurochem_ 2007; 101: 1172–1184. Article CAS Google Scholar * Kamenetz F, Tomita T, Hsieh
H, Seabrook G, Borchelt D, Iwatsubo T _et al_. APP processing and synaptic function. _Neuron_ 2003; 37: 925–937. Article CAS Google Scholar * Hsieh H, Boehm J, Sato C, Iwatsubo T, Tomita
T, Sisodia S _et al_. AMPAR removal underlies A_β_-induced synaptic depression and dendritic spine loss. _Neuron_ 2006; 52: 831–843. Article CAS Google Scholar * Ting JT, Kelley BG,
Lambert TJ, Cook DG, Sullivan JM . Amyloid precursor protein overexpression depresses excitatory transmission through both presynaptic and postsynaptic mechanisms. _Proc Natl Acad Sci USA_
2007; 104: 353–358. Article CAS Google Scholar * Shankar GM, Bloodgood BL, Townsend M, Walsh DM, Selkoe DJ, Sabatini BL . Natural oligomers of the Alzheimer amyloid-beta protein induce
reversible synapse loss by modulating an NMDA-type glutamate receptor-dependent signaling pathway. _J Neurosci_ 2007; 27: 2866–2875. Article CAS Google Scholar * Li S, Hong S, Shepardson
NE, Walsh DM, Shankar GM, Selkoe D . Soluble oligomers of amyloid beta protein facilitate hippocampal long-term depression by disrupting neuronal glutamate uptake. _Neuron_ 2009; 62:
788–801. Article CAS Google Scholar * Spires-Jones T, Knafo S . Spines, plasticity, and cognition in Alzheimer's model mice. _Neural Plast_ 2012; 2012: 319836. Article Google
Scholar * Parodi J, Sepulveda FJ, Roa J, Opazo C, Inestrosa NC, Aguayo LG . Beta-amyloid causes depletion of synaptic vesicles leading to neurotransmission failure. _J Biol Chem_ 2010; 285:
2506–2514. Article CAS Google Scholar * Hiruma H, Katakura T, Takahashi S, Ichikawa T, Kawakami T . Glutamate and amyloid beta-protein rapidly inhibit fast axonal transport in cultured
rat hippocampal neurons by different mechanisms. _J Neurosci_ 2003; 23: 8967–8977. Article CAS Google Scholar * Stokin GB, Lillo C, Falzone TL, Rockenstein E, Mount SL, Raman R _et al_.
Axonopathy and transport deficits early in the pathogenesis of Alzheimer's disease. _Science_ 2005; 307: 1282–1288. Article CAS Google Scholar * Millecamps S, Julien J . Axonal
transport deficits and neurodegenerative diseases. _Nat Rev Neurosci_ 2013; 14: 161–176. Article CAS Google Scholar * Takahashi K, Tanabe K, Ohnuki M, Narita M, Ichisaka T, Tomoda K _et
al_. Induction of pluripotent stem cells from adult human fibroblasts by defined factors. _Cell_ 2007; 131: 861–872. Article CAS Google Scholar * Marchetto MCN, Carromeu C, Acab A, Yu D,
Yeo GW, Mu Y _et al_. A model for neural development and treatment of Rett syndrome using human induced pluripotent stem cells. _Cell_ 2010; 143: 527–539. Article CAS Google Scholar *
Hansen DV, Rubenstein JLR, Kriegstein AR . Deriving excitatory neurons of the neocortex from pluripotent stem cells. _Neuron_ 2011; 70: 645–660. Article CAS Google Scholar * Young JE,
Goldstein LS . Alzheimer's disease in a dish: promises and challenges of human stem cell models. _Hum Mol Genet_ 2012; 21: R82–R89. Article CAS Google Scholar * Li XY, Zhang X,
Johnson MA, Wang ZB, Lavaute T, Zhang SC . Coordination of sonic hedgehog and Wnt signaling determines ventral and dorsal telencephalic neuron types from human embryonic stem cells.
_Development_ 2009; 36: 4055–4063. Article Google Scholar * Chambers SM, Fasano CA, Papapetrou EP, Tomishima M, Sadelain M, Studer L . Highly efficient neural conversion of human ES and
iPS cells by dual inhibition of SMAD signaling. _Nat Biotechnol_ 2009; 27: 275–280. Article CAS Google Scholar * Shi Y, Kirwan P, Smith J, Robinson HPC, Livesey FJ . Human cerebral cortex
development from pluripotent stem cells to functional excitatory synapses. _Nat Neurosci_ 2012; 15: 477–486. Article CAS Google Scholar * Weick JP, Held DL, Bonadurer GF 3rd, Doers ME,
Liu Y, Maguire C _et al_. Deficits in human trisomy 21 iPSCs and neurons. _Proc Natl Acad Sci U S A_ 2013; 110: 9962–9967. Article CAS Google Scholar * Shcheglovitov A, Shcheglovitova O,
Yazawa M, Portmann T, Shu R, Sebastiano V _et al_. SHANK3 and IGF1 restore synaptic deficits in neurons from 22q13 deletion syndrome patients. _Nature_ 2013; 503: 267–271. Article CAS
Google Scholar * Chen H, Qian K, Du Z, Cao J, Petersen A, Liu H _et al_. Modeling ALS with iPSCs reveals that mutant SOD1 misregulates neurofilament balance in motor neurons. _Cell Stem
Cell_ 2014; 14: 796–809. Article CAS Google Scholar * Kiskinis E, Sandoe J, Williams LA, Boulting GL, Moccia R, Wainger BJ _et al_. Pathways disrupted in human ALS motor neurons
identified through genetic correction of mutant SOD1. _Cell Stem Cell_ 2014; 14: 781–795. Article CAS Google Scholar * Yagi T, Ito D, Okada Y, Akamatsu W, Nihei Y, Yoshizaki T _et al_.
Modeling familial Alzheimer's disease with induced pluripotent stem cells. _Hum Mol Genet_ 2011; 20: 4530–4539. Article CAS Google Scholar * Koch P, Tamboli IY, Mertens J, Wunderlich
P, Ladewig J, Stüber K _et al_. Presenilin-1 L166P mutant human pluripotent stem cell-derived neurons exhibit partial loss of γ-secretase activity in endogeneous amyloid-_β_ generation. _Am
J Pathol_ 2012; 180: 2404–2416. Article CAS Google Scholar * Israel MA, Yuan SH, Bardy C, Reyna SM, Mu Y, Herrera C _et al_. Probing sporadic and familial Alzheimer's disease using
induced pluripotent stem cells. _Nature_ 2012; 482: 216–220. Article CAS Google Scholar * Kondo T, Asai M, Tsukita K, Kutoku Y, Ohsawa Y, Sunada Y _et al_. Modeling Alzheimer's
disease with iPSCs reveals stress phenotypes associated with intracellular A_β_ and differential drug responsiveness. _Cell Stem Cell_ 2013; 12: 487–496. Article CAS Google Scholar *
Muratore CR, Rice HC, Srikanth P, Callahan DG, Shin T, Benjamin LN _et al_. The familial Alzheimer's disease AppV717I mutation alters APP processing and Tau expression in iPSC-derived
neurons. _Hum Mol Genet_ 2014; 23: 3523–3536. Article CAS Google Scholar * Barres BA, Silverstein BE, Corey DP, Chun LL . Immunological, morphological, and electrophysiological variation
among retinal ganglion cells purified by panning. _Neuron_ 1988; 1: 791–803. Article CAS Google Scholar * Podlisny MB, Ostaszewski BL, Squazzo SL, Koo EH, Rydell RE, Teplow DB _et al_.
Aggregation of secreted amyloid beta-protein into sodium dodecyl sulphate-stable oligomers in cell culture. _J Biol Chem_ 1995; 270: 9564–9570. Article CAS Google Scholar * Welzel AT,
Maggio JE, Shankar GM, Walker DE, Ostaszewski BL, Li S _et al_. Secreted amyloid _β_-proteins in a cell culture model include N-terminally extended peptides that impair synaptic plasticity.
_Biochemistry_ 2014; 53: 3908–3921. Article CAS Google Scholar * Andreyeva A, Nieweg K, Horstmann K, Klapper S, Müller-Schiffmann A, Korth C _et al_. C-terminal fragment of N-cadherin
accelerates synapse destabilization by amyloid-_β_. _Brain_ 2012; 135: 2140–2154. Article Google Scholar * Müller-Schiffmann A, März-Berberich J, Andreyeva A, Rönicke R, Bartnik D, Brener
O _et al_. Combining independent drug classes into superior, synergistically acting hybrid molecules. _Angew Chem Int Ed Engl_ 2010; 49: 8743–8746. Article Google Scholar * Gaffield MA,
Betz WJ . Imaging synaptic vesicle exocytosis and endocytosis with FM dyes. _Nat Protoc_ 2006; 1: 2916–2921. Article CAS Google Scholar * Kondo T, Asai M, Tsukita K, Kutoku Y, Ohsawa Y,
Sunada Y _et al_. Modelling Alzheimer's disease with iPSCs reveals stress phenotypes associated with intracellular A_β_ and differential drug responsiveness. _Cell Stem Cell_ 2013; 12:
487–496. Article CAS Google Scholar * Zempel H, Mandelkow E . Lost after translation: missorting of Tau protein and consequences for Alzheimer disease. _Trends Neurosci_ 2014; 37:
721–732. Article CAS Google Scholar * Koch P, Opitz T, Steinbeck JA, Ladewig J, Brüstle O . A rosette-type, self-renewing human ES cell-derived neural stem cell with potential for _in
vitro_ instruction and synaptic integration. _Proc Natl Acad Sci USA_ 2009; 106: 3225–3230. Article CAS Google Scholar * Liu H, Zhang SC . Specification of neuronal and glial subtypes
from human pluripotent stem cells. _Cell Mol Life Sci_ 2011; 68: 3995–4008. Article CAS Google Scholar * Zeng H, Guo M, Martins-Taylor K, Wang X, Zhang Z, Park JW _et al_. Specification
of region-specific neurons including forebrain glutamatergic neurons from human induced pluripotent stem cells. _PLoS One_ 2010; 7: e11853. Article Google Scholar * Jüngling K, Nägler K,
Pfrieger FW, Gottmann K . Purification of embryonic stem cell-derived neurons by immunoisolation. _FASEB J_ 2003; 17: 2100–2102. Article Google Scholar * Pielarski KN, van Stegen B,
Andreyeva A, Nieweg K, Jüngling K, Redies C _et al_. Asymmetric N-cadherin expression results in synapse dysfunction, synapse elimination, and axon retraction in cultured mouse neurons.
_PLoS One_ 2013; 8: e54105. Article CAS Google Scholar * Mujtaba T, Rao MS . Isolation of lineage-restricted neural precursors from cultured ES cells. _Methods Mol Biol_ 2002; 185:
189–204. CAS PubMed Google Scholar * Pruszak J, Sonntag K, Aung MH, Sanchez-Pernaute, Isacson O . Markers and methods for cell sorting of human embryonic stem cell-derived neural cell
populations. _Stem Cells_ 2007; 25: 2257–2268. Article Google Scholar * Auer S, Lappalainen RS, Skottmann H, Suuronen R, Narkilahti S, Vikholm-Lundin I . An antibody surface for selective
neuronal cell attachment. _J Neurosci Methods_ 2010; 186: 72–76. Article CAS Google Scholar * Hu BY, Weick JP, Yu J, Ma LX, Zhang XQ, Thomson JA _et al_. Neural differentiation of human
induced pluripotent stem cells follows developmental principles but with variable potency. _Proc Natl Acad Sci USA_ 2010; 107: 4335–4340. Article CAS Google Scholar * Mohrmann R, Lessmann
V, Gottmann K . Developmental maturation of synaptic vesicle cycling as a distinctive feature of central glutamatergic synapses. _Neuroscience_ 2003; 117: 7–18. Article CAS Google Scholar
* Wilcox KC, Lacor PN, Pitt J, Klein WL . A_β_ oligomer-induced synapse degeneration in Alzheimer's disease. _Cell Mol Neurobiol_ 2011; 31: 939–948. Article CAS Google Scholar *
Lacor PN, Buniel MC, Furlow PW, Clemente AS, Velasco PT, Wood M _et al_. A_β_ oligomer-induced aberrations in synapse composition, shape, and density provide a molecular basis for loss of
connectivity in Alzheimer's disease. _J Neurosci_ 2007; 27: 796–807. Article CAS Google Scholar * Evans NA, Facci L, Owen DA, Soden PE, Burbidge SA, Prinjha RK _et al_. A_β_1-42
reduces synapse number and inhibits neurite outgrowth in primary cortical and hippocampal neurons: a quantitative analysis. _J Neurosci Methods_ 2008; 175: 96–103. Article CAS Google
Scholar * Wu HY, Hudry E, Hashimoto T, Kuchibhotla K, Rozkaine A, Fan S _et al_. Amyloid beta induces the morphological neurodegenerative triad of spine loss, dendritic simplification, and
neuritic dystrophies through calcineurin activation. _J Neurosci_ 2010; 30: 2636–2649. Article CAS Google Scholar * Koffie RM, Hashimoto T, Tai H, Kay KR, Serrano-Pozo A, Joyner D _et
al_. Apolipoprotein E4 effects in Alzheimer's disease are mediated by synaptotoxic oligomeric amyloid-_β_. _Brain_ 2012; 135: 2155–2168. Article Google Scholar * Gu Z, Liu W, Yan Z .
_β_-Amyloid impairs AMPA receptor trafficking and function by reducing Ca2+/calmodulin-dependent protein kinase II synaptic distribution. _J Biol Chem_ 2009; 284: 10639–10649. Article CAS
Google Scholar * Braak H, Alafuzoff I, Arzberger T, Kretzschmar H, Del Tredici K . Staging of Alzheimer disease-associated neurofibrillary pathology using paraffin sections and
immunocytochemistry. _Acta Neuropathol_ 2006; 112: 389–404. Article Google Scholar * Sano R, Reed JC . ER stress-induced cell death mechanisms. _Biochim Biophys Acta_ 2013; 1833:
3460–3470. Article CAS Google Scholar * Endres K, Reinhardt S . ER-stress in Alzheimer's disease: turning the scale? _Am J Neurodegener Dis_ 2013; 2: 247–265. PubMed PubMed Central
Google Scholar * Krueger SR, Kolar A, Fitzsimonds RM . The presynaptic release apparatus is functional in the absence of dendritic contact and highly mobile within isolated axons.
_Neuron_ 2003; 40: 945–957. Article CAS Google Scholar * Ratnayaka A, Marra V, Branco T, Staras K . Extrasynaptic vesicle recycling in mature hippocampal neurons. _Nat Commun_ 2011; 2:
531. Article Google Scholar * Decker H, Lo KY, Unger SM, Ferreira ST, Silverman MA . Amyloid-_β_ peptide oligomers disrupt axonal transport through an NMDA receptor-dependent mechanism
that is mediated by glycogen synthase kinase 3_β_ in primary cultured hippocampal neurons. _J Neurosci_ 2010; 30: 9166–9171. Article CAS Google Scholar * Tang Y, Scott DA, Das U, Endland
SD, Radomski K, Koo EH _et al_. Early and selective impairments in axonal transport kinetics of synaptic cargoes induced by soluble amyloid _β_-protein. _Traffic_ 2012; 13: 681–693. Article
CAS Google Scholar * Zaehres H, Kögler G, Arauzo-Bravo MJ, Bleidissel M, Santourlidis S, Weinhold S _et al_. Induction of pluripotency in human cord blood unrestricted somatic stem
cells. _Exp Hematol_ 2010; 38: 809–818. Article CAS Google Scholar * Yu J, Hu K, Smuga-Otto K, Tian S, Stewart R, Slukvin II _et al_. Human induced pluripotent stem cells free of vector
and transgene sequences. _Science_ 2009; 324: 797–801. Article CAS Google Scholar * Chen G, Gulbranson DR, Hou Z, Bolin JM, Ruotti V, Probasco MD _et al_. Chemically defined conditions
for human iPSC derivation and culture. _Nat Methods_ 2011; 8: 424–429. Article CAS Google Scholar * Chen Y, Stevens B, Chang J, Milbrandt J, Barres BA, Hell JW . NS21: re-defined and
modified supplement B27 for neuronal cultures. _J Neurosci Methods_ 2008; 171: 239–247. Article CAS Google Scholar * Nieweg K, Schaller H, Pfrieger FW . Marked differences in cholesterol
synthesis between neurons and glial cells from postnatal rats. _J Neurochem_ 2009; 109: 125–134. Article CAS Google Scholar * Jüngling K, Eulenburg V, Moore R, Kemler R, Lessmann V,
Gottmann K . N-cadherin transsynaptically regulates short-term plasticity at glutamatergic synapses in embryonic stem cell-derived neurons. _J Neurosci_ 2006; 26: 6968–6978. Article Google
Scholar Download references ACKNOWLEDGEMENTS We thank Dr. PW Andrews for providing hybridoma supernatant containing NCAM antibody, and Dr. H Zaehres, Dr. H Schöler, Dr. P Wernet, and Dr. G
Kögler for providing human iPSCs (USSC-iPSCs line 08/25). We also thank Dr. DJ Selkoe for providing 7PA2 CHO cells, and Dr. C Korth and Dr. A Müller-Schiffmann for providing IC16 antibody
for A_β_ immunodepletion. We further thank M Bohndorf for excellent technical assistance. This work was supported by the Forschungskommission (No. 52/2011), Medical Faculty,
Heinrich-Heine-University Düsseldorf and the Strategischer Forschungsfonds, Heinrich-Heine-University Düsseldorf. AUTHOR INFORMATION Author notes * A Andreyeva and B van Stegen: These
authors contributed equally to this work. AUTHORS AND AFFILIATIONS * Institute of Neuro- and Sensory Physiology, Medical Faculty, Heinrich-Heine-University Düsseldorf, Düsseldorf, Germany K
Nieweg, A Andreyeva, B van Stegen & K Gottmann * Institute of Pharmacology and Clinical Pharmacy, Phillips University, Marburg, Germany K Nieweg & G Tanriöver Authors * K Nieweg View
author publications You can also search for this author inPubMed Google Scholar * A Andreyeva View author publications You can also search for this author inPubMed Google Scholar * B van
Stegen View author publications You can also search for this author inPubMed Google Scholar * G Tanriöver View author publications You can also search for this author inPubMed Google Scholar
* K Gottmann View author publications You can also search for this author inPubMed Google Scholar CORRESPONDING AUTHORS Correspondence to K Nieweg or K Gottmann. ETHICS DECLARATIONS
COMPETING INTERESTS The authors declare no conflict of interest. ADDITIONAL INFORMATION Edited by A Verkhratsky Supplementary Information accompanies this paper on Cell Death and Disease
website SUPPLEMENTARY INFORMATION SUPPLEMENTARY TABLE 1 (DOC 59 KB) SUPPLEMENTARY FIGURE 1 (JPG 416 KB) SUPPLEMENTARY FIGURE 2 (JPG 576 KB) SUPPLEMENTARY FIGURE LEGENDS (DOC 33 KB) RIGHTS
AND PERMISSIONS _Cell Death and Disease_ is an open-access journal published by _Nature Publishing Group_. This work is licensed under a Creative Commons Attribution 4.0 International
License. The images or other third party material in this article are included in the article’s Creative Commons license, unless indicated otherwise in the credit line; if the material is
not included under the Creative Commons license, users will need to obtain permission from the license holder to reproduce the material. To view a copy of this license, visit
http://creativecommons.org/licenses/by/4.0/ Reprints and permissions ABOUT THIS ARTICLE CITE THIS ARTICLE Nieweg, K., Andreyeva, A., van Stegen, B. _et al._ Alzheimer's disease-related
amyloid-_β_ induces synaptotoxicity in human iPS cell-derived neurons. _Cell Death Dis_ 6, e1709 (2015). https://doi.org/10.1038/cddis.2015.72 Download citation * Received: 02 August 2014 *
Revised: 13 February 2015 * Accepted: 17 February 2015 * Published: 02 April 2015 * Issue Date: April 2015 * DOI: https://doi.org/10.1038/cddis.2015.72 SHARE THIS ARTICLE Anyone you share
the following link with will be able to read this content: Get shareable link Sorry, a shareable link is not currently available for this article. Copy to clipboard Provided by the Springer
Nature SharedIt content-sharing initiative